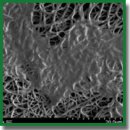
A Technology for Anti-Thrombogenic Drug Coating of Small-Diameter Biodegradable Vascular Prostheses
The aim of the study was to develop a technology for anti-thrombogenic drug coating of biodegradable porous scaffolds and to evaluate the physicomechanical and hemocompatible properties of functionally active vascular prostheses with and without a drug coating.
Materials and Methods. Vascular prostheses from polyhydroxybutyrate/valerate and polycaprolactone with the incorporated vascular endothelial growth factor, the main fibroblast growth factor, and the chemoattractant SDF-1α were made by emulsion electrospinning. Additional surface modification of the prostheses was carried out by forming a hydrogel coating of polyvinylpyrrolidone capable of binding drugs as a result of complexation. Unfractionated heparin and iloprost were used as anti-thrombogenic drugs.
Results. We show that after the modification of vascular prostheses with heparin and iloprost, a 5.8-fold increase in the Young’s modulus value was noted, which indicated a greater stiffness of these grafts compared to the unmodified controls. Platelet aggregation on the surface of heparin + iloprost coated vascular prostheses was 3.3 times less than that with the unmodified controls, and 1.8 times less compared to intact platelet-rich plasma. The surface of vascular prostheses with heparin and iloprost was resistant to adhesion of platelets and blood proteins.
Conclusion. Drug (unfractionated heparin and iloprost) coating of the surface of biodegradable prostheses significantly improved the anti-thrombogenic properties of these grafts but contributed to the increased stiffness of the prostheses.
- Pashneh-Tala S., MacNeil S., Claeyssens F. The tissue-engineered vascular graft — past, present, and future. Tissue Eng Part B Rev 2016; 22(1): 68–100, https://doi.org/10.1089/ten.teb.2015.0100.
- Devine C., McCollum C.; North West Femoro-Popliteal Trial Participants. Heparin-bonded Dacron or polytetrafluorethylene for femoropopliteal bypass: five-year results of a prospective randomized multicenter clinical trial. J Vasc Surg 2004; 40(5): 924–931, https://doi.org/10.1016/j.jvs.2004.08.033.
- Samson R.H., Morales R., Showalter D.P., Lepore M.R., Nair D.G. Heparin-bonded expanded polytetrafluoroethylene femoropopliteal bypass grafts outperform expanded polytetrafluoroethylene grafts without heparin in a long-term comparison. J Vasc Surg 2016; 64(3): 638–647, https://doi.org/10.1016/j.jvs.2016.03.414.
- Shoji T., Shinoka T. Tissue engineered vascular grafts for pediatric cardiac surgery. Transl Pediatr 2018; 7(2): 188–195, https://doi.org/10.21037/tp.2018.02.01.
- Ren X., Feng Y., Guo J., Wang H., Li Q., Yang J., Hao X., Lv J., Ma N., Li W. Surface modification and endothelialization of biomaterials as potential scaffolds for vascular tissue engineering applications. Chem Soc Rev 2015; 44(15): 5680–5742, https://doi.org/10.1039/c4cs00483c.
- Choi D.H., Kang S.N., Kim S.M., Gobaa S., Park B.J., Kim I.H., Joung Y.K., Han D.K. Growth factors-loaded stents modified with hyaluronic acid and heparin for induction of rapid and tight re-endothelialization. Colloids Surf B Biointerfaces 2016; 141: 602–610, https://doi.org/10.1016/j.colsurfb.2016.01.028.
- Hu Y.T., Pan X.D., Zheng J., Ma W.G., Sun L.Z. In vitro and in vivo evaluation of a small-caliber coaxial electrospun vascular graft loaded with heparin and VEGF. Int J Surg 2017; 44: 244–249, https://doi.org/10.1016/j.ijsu.2017.06.077.
- Duan H.Y., Ye L., Wu X., Guan Q., Yang X.F., Han F., Liang N., Wang Z.F., Wang Z.G. The in vivo characterization of electrospun heparin-bonded polycaprolactone in small-diameter vascular reconstruction. Vascular 2015; 23(4): 358–365, https://doi.org/10.1177/1708538114550737.
- Gao J., Jiang L., Liang Q., Shi J., Hou D., Tang D., Chen S., Kong D., Wang S. The grafts modified by heparinization and catalytic nitric oxide generation used for vascular implantation in rats. Regen Biomater 2018; 5(2): 105–114, https://doi.org/10.1093/rb/rby003.
- Wang W., Liu D., Li D., Du H., Zhang J., You Z., Li M., He C. Nanofibrous vascular scaffold prepared from miscible polymer blend with heparin/stromal cell-derived factor-1 alpha for enhancing anticoagulation and endothelialization. Colloids Surf B Biointerfaces 2019; 181: 963–972, https://doi.org/10.1016/j.colsurfb.2019.06.065.
- Antonova L.V., Matveeva V.G., Velikanova E.A., Khanova M.Y., Sevostyanova V.V., Tsepokina A.V., Elgudin Y.L., Barbarash L.S. In vitro activity of bioactive molecules incorporated into poly (3-hydroxybutyrate-co-3-hydroxyvalerate)/poly(ε-caprolactone) scaffolds. Kompleksnye problemy serdechno-sosudistyh zabolevanij 2018; 7(2): 89–101, https://doi.org/10.17802/2306-1278-2018-7-2-89-101.
- Antonova L.V., Sevostyanova V.V., Mironov A.V., Krivkina E.O., Velikanova E.A., Matveeva V.G., Glushkovа T.V., Elgudin Ya.L., Barbarash L.S. In situ vascular tissue remodeling using biodegradable tubular scaffolds with incorporated growth factors and chemoattractant molecules. Kompleksnye problemy serdechno-sosudistyh zabolevanij 2018; 7(2): 25–36, https://doi.org/10.17802/2306-1278-2018-7-2-25-36.
- Antonova L.V., Sevostyanova V.V., Kutikhin A.G., Velikanova Е.A., Matveeva V.G., Glushkova T.V., Mironov A.V., Krivkina E.O., Barbarash O.L., Barbarash L.S. Influence of bFGF, SDF-1α, or VEGF incorporated into tubular polymer scaffolds on the formation of small-diameter tissue-engineered blood vessel in vivo. Vestnik transplantologii i iskusstvennyh organov 2018; 20(1): 96–109, https://doi.org/10.15825/1995-1191-2018-1-96-109.
- Antonova L.V., Sevostyanova V.V., Rezvova M.A., Krivkina E.O., Kudryavtseva Yu.A., Barbarash O.L., Barbarash L.S. Technology of producing functionally active biodegradable small-diameter vascular prostheses with drug coating. Patent RU 2702239. 2019.
- Ye X., Wang Z., Zhang X., Zhou M., Cai L. Hemocompatibility research on the micro-structure surface of a bionic heart valve. Biomed Mater Eng 2014; 24(6): 2361–2369, https://doi.org/10.3233/bme-141049.
- Shen X., Su F., Dong J., Fan Z., Duan Y., Li S. In vitro biocompatibility evaluation of bioresorbable copolymers prepared from L-lactide, 1, 3-trimethylene carbonate and glycolide for cardiovascular applications. J Biomater Sci Polym Ed 2015; 26(8): 497–514, https://doi.org/10.1080/09205063.2015.1030992.
- Jung F., Braune S., Lendlein A. Haemocompatibility testing of biomaterials using human platelets. Clin Hemorheol Microcirc 2013; 53(1–2): 97–115, https://doi.org/10.3233/ch-2012-1579.
- Jolee Bartrom B.S. ASTM hemolysis. NAMSA; 2008.
- Sevostyanova V.V., Krivkina E.O., Antonova L.V. Approaches to antithrombotic modification of vascular implants. Kazanskij medicinskij zurnal 2020; 101(2): 232–242, https://doi.org/10.17816/kmj2020-232.