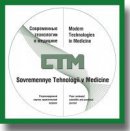
Computer Program for Detection and Analyzing the Porin-Mediated Antibiotic Resistance of Bacteria
The aim of this work was to develop a new software tool for identifying gene mutations that determine the porin-mediated resistance to antibiotics in gram-negative bacteria and to demonstrate the functionality of this program by detecting porin-mediated resistance to carbapenems in clinical isolates of Pseudomonas aeruginosa.
Materials and Methods. The proposed algorithm is based on searching for a correspondence between the reference and the studied genes. When the sought nucleotide sequence is found in the analyzed genome, it is compared with the reference one and analyzed. The genomic analysis is then verified by comparing between the amino acid sequences encoded by the reference and studied genes. The genes of the susceptible P. aeruginosa ATCC 27853 strain were used as the reference nucleotide sequences encoding for porins (OprD, OpdD, and OpdP) involved in the transport of carbapenems into the bacterial cell. The complete genomes of clinical P. aeruginosa isolates from the PATRIC database 3.6.9 and our own collection were used to test the functionality of the proposed program. The analyzed isolates were phenotypically characterized according to the CLSI standard. The search for carbapenemase genes in the studied genomes of P. aeruginosa was carried out using the ResFinder 4.1.
Results. The developed program for detecting the genetic determinants of non-plasmid antibiotic resistance made it possible to identify mutations of various types and significance in the porin genes of P. aeruginosa clinical isolates. These mutations led to modifications of the peptide structure of porin proteins. Single amino acid substitutions prevailed in the OpdD and OpdP porins of carbapenem-susceptible and carbapenem-resistant isolates. In the carbapenem-resistant strains, the gene encoding for OprD porin was found heavily modified, including insertions and/or deletions, which led to premature termination of porin synthesis. In several isolates resistant to meropenem, no mutations were detected in the gene encoding for OprD, which might be associated with alternative mechanisms of resistance to carbapenems.
Conclusion. The proposed software product can become an effective tool for deciphering the molecular genetic mechanisms of bacterial chromosomal resistance to antibiotics. Testing the program revealed differences between the occurrences of mutations significant for carbapenem resistance in the oprD, opdD, and opdP genes.
- Talebi Bezmin Abadi A., Rizvanov A.A., Haertlé T., Blatt N.L. World Health Organization report: current crisis of antibiotic resistance. Bionanoscience 2019; 9: 778–788, https://doi.org/10.1007/s12668-019-00658-4.
- Devi S. No time to lower the guard on AMR. Lancet Microbe 2020; 1(5): e198, https://doi.org/10.1016/s2666-5247(20)30129-4.
- Knight G.M., Glover R.E., McQuaid C.F., Olaru I.D., Gallandat K., Leclerc Q.J., Fuller N.M., Willcocks S.J., Hasan R., van Kleef E., Chandler C.I. Antimicrobial resistance and COVID-19: intersections and implications. Elife 2021; 10: e64139, https://doi.org/10.7554/elife.64139.
- World Health Organization. Global action plan on antimicrobial resistance. WHO, Library Cataloguing-in-Publication Data; 2015. URL: https://www.who.int/publications/i/item/9789241509763.
- Bennett P.M. Plasmid encoded antibiotic resistance: acquisition and transfer of antibiotic resistance genes in bacteria. Br J Pharmacol 2008; 153 Suppl 1(Suppl 1): S347–S357, https://doi.org/10.1038/sj.bjp.0707607.
- Partridge S.R. Analysis of antibiotic resistance regions in Gram-negative bacteria. FEMS Microbiol Rev 2011; 35(5): 820–855, https://doi.org/10.1111/j.1574-6976.2011.00277.x.
- van Hoek H.A.M., Mevius D., Guerra B., Mullany P., Roberts A.P., Aarts H.J. Acquired antibiotic resistance genes: an overview. Front Microbiol 2011; 2: 203, https://doi.org/10.3389/fmicb.2011.00203.
- Woodford N., Ellington M.J. The emergence of antibiotic resistance by mutation. Clin Microbiol Infect 2007; 13(1): 5–18, https://doi.org/10.1111/j.1469-0691.2006.01492.x.
- Sultan I., Rahman S., Jan A.T., Siddiqui M.T., Mondal A.H., Haq Q.M.R. Antibiotics, resistome and resistance mechanisms: a bacterial perspective. Front Microbiol 2018; 9: 2066, https://doi.org/10.3389/fmicb.2018.02066.
- López-Causapé C., Sommer L.M., Cabot G., Rubio R., Ocampo-Sosa A.A., Johansen H.K., Figuerola J., Cantón R., Kidd T.J., Molin S., Oliver A. Evolution of the Pseudomonas aeruginosa mutational resistome in an international cystic fibrosis clone. Sci Rep 2017; 7(1): 5555, https://doi.org/10.1038/s41598-017-05621-5.
- Bortolaia V., Kaas R.S., Ruppe E., Roberts M.C., Schwarz S., Cattoir V., Philippon A., Allesoe R.L., Rebelo A.R., Florensa A.F., Fagelhauer L., Chakraborty T., Neumann B., Werner G., Bender J.K., Stingl K., Nguyen M., Coppens J., Xavier B.B., Malhotra-Kumar S., Westh H., Pinholt M., Anjum M.F., Duggett N.A., Kempf I., Nykäsenoja S., Olkkola S., Wieczorek K., Amaro A., Clemente L., Mossong J., Losch S., Ragimbeau C., Lund O., Aarestrup F.M. ResFinder 4.0 for predictions of phenotypes from genotypes. J Antimicrob Chemother 2020; 75(12): 3491–3500, https://doi.org/10.1093/jac/dkaa345.
- Alcock B.P., Raphenya A.R., Lau T.T.Y., Tsang K.K., Bouchard M., Edalatmand A., Huynh W., Nguyen A.V., Cheng A.A., Liu S., Min S.Y., Miroshnichenko A., Tran H.K., Werfalli R.E., Nasir J.A., Oloni M., Speicher D.J., Florescu A., Singh B., Faltyn M., Hernandez-Koutoucheva A., Sharma A.N., Bordeleau E., Pawlowski A.C., Zubyk H.L., Dooley D., Griffiths E., Maguire F., Winsor G.L., Beiko R.G., Brinkman F.S.L., Hsiao W.W.L., Domselaar G.V., McArthur A.G. CARD 2020: antibiotic resistome surveillance with the comprehensive antibiotic resistance database. Nucleic Acids Res 2020; 48(D1): D517–D525, https://doi.org/10.1093/nar/gkz935.
- Liu B., Pop M. ARDB — Antibiotic Resistance Genes Database. Nucleic Acids Res 2009; 37(Database issue): D443–D447, https://doi.org/10.1093/nar/gkn656.
- Gupta S.K., Padmanabhan B.R., Diene S.M., Lopez-Rojas R., Kempf M., Landraud L., Rolain J.M. ARG-ANNOT, a new bioinformatic tool to discover antibiotic resistance genes in bacterial genomes. Antimicrob Agents Chemother 2014; 58(1): 212–220, https://doi.org/10.1128/aac.01310-13.
- Software “Computer program for the detection of genetic determinants of non-plasmid antibiotic resistance. Federal Service for Intellectual Property (Rospatent). Certificate of registration 2021665744. Oct 1, 2021.
- Trias J., Nikaido H. Outer membrane protein D2 catalyzes facilitated diffusion of carbapenems and penems through the outer membrane of Pseudomonas aeruginosa. Antimicrob Agents Chemother 1990; 34(1): 52–57, https://doi.org/10.1128/aac.34.1.52.
- Quinn J.P., Darzins A., Miyashiro D., Ripp S., Miller R.V. Imipenem resistance in Pseudomonas aeruginosa PAO: mapping of the OprD2 gene. Antimicrob Agents Chemother 1991; 35(4): 753–755, https://doi.org/10.1128/aac.35.4.753.
- Chevalier S., Bouffartigues E., Bodilis J., Maillot O., Lesouhaitier O., Feuilloley M.G.J., Orange N., Dufour A., Cornelis P. Structure, function and regulation of Pseudomonas aeruginosa porins. FEMS Microbiol Rev 2017; 41(5): 698–722, https://doi.org/10.1093/femsre/fux020.
- Sonnleitner E., Pusic P., Wolfinger M.T., Bläsi U. Distinctive regulation of carbapenem susceptibility in Pseudomonas aeruginosa by Hfq. Front Microbiol 2020; 11: 1001, https://doi.org/10.3389/fmicb.2020.01001.
- Soundararajan G., Bhamidimarri S.P., Winterhalter M. Understanding carbapenem translocation through OccD3 (OpdP) of Pseudomonas aeruginosa. ACS Chem Biol 2017; 12(6): 1656–1664, https://doi.org/10.1021/acschembio.6b01150.
- Camacho C., Coulouris G., Avagyan V., Ma N., Papadopoulos J., Bealer K., Madden T.L. BLAST+: architecture and applications. BMC Bioinformatics 2009; 10: 421, https://doi.org/10.1186/1471-2105-10-421.
- Pang Z., Raudonis R., Glick B.R., Lin T.J., Cheng Z. Antibiotic resistance in Pseudomonas aeruginosa: mechanisms and alternative therapeutic strategies. Biotechnol Adv 2019; 37(1): 177–192, https://doi.org/10.1016/j.biotechadv.2018.11.013.
- Chebotar I.V., Bocharova Yu.A., Mayansky N.A. Mechanisms and regulation of antimicrobial resistance in Pseudomonas aeruginosa. Kliniceskaa mikrobiologia i antimikrobnaa himioterapia 2017; 19(4): 308–319.
- Saito R., Koyano S., Dorin M., Higurashi Y., Misawa Y., Nagano N., Kaneko T., Moriya K. Evaluation of a simple phenotypic method for the detection of carbapenemase-producing Enterobacteriaceae. J Microbiol Methods 2015; 108: 45–48, https://doi.org/10.1016/j.mimet.2014.11.008.
- Dallenne C., Da Costa A., Decré D., Favier C., Arlet G. Development of a set of multiplex PCR assays for the detection of genes encoding important beta-lactamases in Enterobacteriaceae. J Antimicrob Chemother 2010; 65(3): 490–495, https://doi.org/10.1093/jac/dkp498.
- Suresh M., Skariyachan S., Narayanan N., Pullampara Rajamma J., Panickassery Ramakrishnan M.K. Mutational variation analysis of oprD porin gene in multidrug-resistant clinical isolates of Pseudomonas aeruginosa. Microb Drug Resist 2020; 26(8): 869–879, https://doi.org/10.1089/mdr.2019.0147.