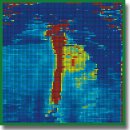
A New Criterion for Shear Wave Elastometric Assessment Using Modulus of Stiffness Difference between Object and Environment
Ultrasound shear wave elastography is a modern method that is based on measuring the shear wave velocity making it possible to determine stiffness of soft biological tissues at an arbitrary point (point elastography) or to construct a two-dimensional color image with a subsequent point measurement of stiffness (two-dimensional elastography) and therefore to compare the stiffness of an object with a medium or objects with each other.
The aim of the study is to develop a new criterion for the comparative assessment of objects with different stiffness during shear wave elastometry: modulus of stiffness difference between object and environment.
Materials and Methods. Using the original technology of building two-dimensional color elastogram, point and two-dimensional shear wave elastography were performed using linear sensors on commercial ultrasound scanners: Aixplorer (SuperSonic Imagine, France), Acuson S2000 (Siemens, Germany), and Verasonics acoustic system (Verasonics Inc., USA) with an open architecture to determine the stiffness values of focal inclusions and compare them with each other with the help of a new comparative elastomeric assessment criterion: modulus of stiffness difference between object and environment. First, the accuracy of the scanners under test was compared on a calibrated Elasticity QA Phantom, model 049 (Computerized Imaging Reference Systems Company, USA) with a known stiffness of various inclusions and thereafter on an uncalibrated BP1901 phantom (Blue Phantom, USA) with unknown stiffness of inclusions. The obtained values were compared to determine the influence of subjective factors on the measurement results.
Results. To assess the stiffness of the foci and compare the values with each other taking into account the rigidity of the environment, it is proposed to use a new criterion for the comparative assessment — the modulus of stiffness difference between focus and environment, which quantitatively characterizes the difference between these values. According to this criterion, all three ultrasound scanners have been established to show high and comparable accuracy in determining the stiffness of inclusions within the homogeneous medium in the experiments on phantoms. Two-dimensional shear wave elastography has revealed the effect of the control volume size and the correctness of the color scale setting, especially in the heterogeneous objects, on the results of elastometry. Methodological techniques to reduce the influence of subjective factors have also been proposed.
Conclusion. The study has showed the possibility of using the modulus of stiffness difference between object and environment as a new criterion for comparative assessment of objects in shear wave elastometry taking into account stiffness of the environment. To reduce operator-dependence, it is necessary to take into consideration both the way of realizing elastometry (point or two-dimensional color elastography) and a number of other methodological factors.
- Sarvazyan A.P., Rudenko O.V., Swanson S.D., Fowlkes J.B., Emelianov S.Y. Shear wave elasticity imaging: a new ultrasonic technology of medical diagnostics. Ultrasound Med Biol 1998; 24(9): 1419–1435, https://doi.org/10.1016/s0301-5629(98)00110-0.
- Sarvazyan A.P., Rudenko O.V., Fatemi M. Acoustic radiation force: a review of four mechanisms for biomedical applications. IEEE Trans Ultrason Ferroelectr Freq Control 2021; 68(11): 3261–3269, https://doi.org/10.1109/tuffc.2021.3112505.
- Shiina T., Nightingale K.R., Palmeri M.L., Hall T.J., Bamber J.C., Barr R.G., Castera L., Choi B.I., Chou Y.H., Cosgrove D., Dietrich C.F., Ding H., Amy D., Farrokh A., Ferraioli G., Filice C., Friedrich-Rust M., Nakashima K., Schafer F., Sporea I., Suzuki S., Wilson S., Kudo M. WFUMB guidelines and recommendations for clinical use of ultrasound elastography: part 1: basic principles and terminology. Ultrasound Med Biol 2015; 41(5): 1126–1147, https://doi.org/10.1016/j.ultrasmedbio.2015.03.009.
- Mitkov V.V., Mitkova M.D. Ultrasound shear wave elastography. Ul’trazvukovaa i funkcional’naa diagnostika 2015; 2: 94–108.
- Rudenko O.V., Safonov D.V., Demin I.Yu., Rykhtik P.I., Andreev V.G., Gurbatov S.N., Romanov S.V. Glava 1. Osnovy elastografii sdvigovoy volnoy: teoriya i fizicheskiy eksperiment. V kn.: Elastografiya sdvigovoy volny: analiz klinicheskikh primerov [Chapter 1. Fundamentals of shear wave elastography: theory and physical experiment. In: Shear wave elastography: an analysis of clinical cases]. Borsukov A.V. (editor). Smolensk: Smolenskaya gorodskaya tipografiya; 2017; р. 8–41.
- Dietrich C.F., Bamber J., Berzigotti A., Bota S., Cantisani V., Castera L., Cosgrove D., Ferraioli G., Friedrich-Rust M., Gilja O.H., Goertz R.S., Karlas T., de Knegt R., de Ledinghen V., Piscaglia F., Procopet B., Saftoiu A., Sidhu P.S., Sporea I., Thiele M. EFSUMB guidelines and recommendations on the clinical use of liver ultrasound elastography, update 2017 (long version). Ultraschall Med 2017; 38(4): e16–e47, https://doi.org/10.1055/s-0043-103952.
- Safonov D.V., Rykhtik P.I., Shatokhina I.V., Romanov S.V., Gurbatov S.N., Demin I.Yu. Shear wave elastography: comparing the accuracy of ultrasound scanners using calibrated phantoms in experiment. Sovremennye tehnologii v medicine 2017; 9(4): 51, https://doi.org/10.17691/stm2017.9.4.06.
- Kulberg N.S., Osipov L.V., Usanov M.S. Comparative analysis of the elastography technologies in ultrasonic diagnostic devices using elastography phantom. Radiologia – praktika 2016; 56(2): 6–23.
- Khalitov R.S., Gurbatov S.N., Demin I.Y. The use of the Verasonics ultrasound system to measure shear wave velocities in CIRS phantoms. Phys Wave Phenom 2016; 24(1): 73–76, https://doi.org/10.3103/s1541308x16010143.
- Yu Y., Xiao Y., Cheng J., Chiu B. Breast lesion classification based on supersonic shear-wave elastography and automated lesion segmentation from B-mode ultrasound images. Comput Biol Med 2018; 93: 31–46, https://doi.org/10.1016/j.compbiomed.2017.12.006.
- Chamming’s F., Hangard C., Gennisson J.L., Reinhold C., Fournier L.S. Diagnostic accuracy of four levels of manual compression applied in supersonic shear wave elastography of the breast. Acad Radiol 2021; 28(4): 481–486, https://doi.org/10.1016/j.acra.2020.03.012.