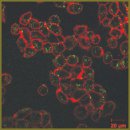
Integrated Study of the Interaction Between Gold Nanorods and Cancer Cells
The aim of the investigation was to study the interaction of the gold nanorods stabilized by biocompatible agents with cancer cells.
Materials and Methods. The research was carried out on gold nanorods with extinction peak about 808 nm and 41×10 nm in size. Pluronic F127, chitosan and polyethylene glycol (PEG) solutions were used for stabilization. Optical characteristics of the solutions were investigated by a double-beam spectrophotometer. A cytotoxic effect of the nanoparticles was estimated by MTT assay. The interaction of the nanorods with eukaryotic cells was studied by two-photon luminescence microscopy and atomic force microscopy techniques. Transmission electron microscopy was used to perform a semi-quantitative analysis and study intracellular distribution.
Results. Stabilization of nanoparticles by Pluronic F127, chitosan and PEG resulted in the changes of their spectral characteristics with broadening of extinction peak and decrease of its intensity. All studied solutions of nanoparticles had the similar level of cytotoxicity. The nanoparticles found in cells were in the form of separate nanoparticles, larger assemblages and aggregates, the last two fractions prevailing. PEG-stabilized nanoparticles (after 1–3 h) and chitosan-stabilized nanoparticles (after 6 h) penetrated into cells the most efficiently. Endocytosis has been shown to play the main role in the internalization of nanoparticles.
Conclusion. The obtained results may lead to better understanding of the interaction process of nanomaterials with living cells, the influence of the stabilizing agent nature on optical properties of nanoparticles and their cytotoxic effects. Besides, the data on the accumulation of nanoparticles in cells will be useful when developing imaging techniques and therapy using nanomaterials.
- Astruc D. Transition-metal nanoparticles in catalysis: from historical background to the state-of-the art. In: Nanoparticles and catalysis. Edited by Astruc D. Weinheim, Germany: Wiley-VCH Verlag GmbH & Co. KGaA; 2008, http://dx.doi.org/10.1002/9783527621323.ch1.
- Saha K., Agasti S.S., Kim C., Li X.N., Rotello V.M. Gold nanoparticles in chemical and biological sensing. Chem Rev 2012; 112(5): 2739–2779, http://dx.doi.org/10.1021/cr2001178.
- Khlebtsov N.G. Optics and biophotonics of nanoparticles with a plasmon resonance. Quantum Electronics 2008, 38(6): 504–529, http://dx.doi.org/10.1070/QE2008v038n06ABEH013829.
- Khlebtsov N.G., Dykman L.A. Optical properties and biomedical applications of plasmonic nanoparticles. J Quant Spectrosc Radiat Transfer 2010; 111(1): 1–35, http://dx.doi.org/10.1016/j.jqsrt.2009.07.012.
- Stark W.J. Nanoparticles in biological systems. Angew Chem Int Ed Engl 2011; 50(6): 1242–1258, http://dx.doi.org/10.1002/anie.200906684.
- Ali Z., Abbasi A.Z., Zhang F., Arosio P., Lascialfari A., Casula M.F., Wenk A., Kreyling W., Plapper R., Seidel M., Niessner R., Knöll J., Seubert A., Parak W.J. Multifunctional nanoparticles for dual imaging. Anal Chem 2011; 83(8): 2877–2882, http://dx.doi.org/10.1021/ac103261y.
- Krpetić Z., Nativo P., Sée V., Prior I.A., Brust M., Volk M. Inflicting controlled nonthermal damage to subcellular structures by laser-activated gold nanoparticles. Nano Lett 2010; 10(11): 4549–4554, http://dx.doi.org/10.1021/nl103142t.
- Chen J., Glaus C., Laforest R., Zhang Q., Yang M., Gidding M., Welch M.J., Xia Y. Gold nanocages as photothermal transducers for cancer treatment. Small 2010; 6(7): 811–817, http://dx.doi.org/10.1002/smll.200902216.
- Phillips M.A., Gran M.L., Peppas N.A. Targeted nanodelivery of drugs and diagnostics. Nano Today 2010; 5(2): 143–159, http://dx.doi.org/10.1016/j.nantod.2010.03.003.
- Kang B., Mackey M.A., El-Sayed M.A. Nuclear targeting of gold nanoparticles in cancer cells induces DNA damage, causing cytokinesis arrest and apoptosis. J Am Chem Soc 2010; 132(5): 1517–1519, http://dx.doi.org/10.1021/ja9102698.
- von Maltzahn G., Park J.H., Agrawal A., Bandaru N.K., Das S.K., Sailor M.J., Bhatia S.N. Computationally guided photothermal tumor therapy using long-circulating gold nanorod antennas. Cancer Res 2009; 69(9): 3892–3900, http://dx.doi.org/10.1158/0008-5472.CAN-08-4242.
- Rozanova N., Zhang J. Photothermal ablation therapy for cancer based on metal nanostructures. Sci China Ser B: Chem 2009, 52(10): 1559–1575, http://dx.doi.org/10.1007/s11426-009-0247-0.
- Chithrani B.D., Ghazani A.A., Chan W.C. Determining the size and shape dependence of gold nanoparticle uptake into mammalian cells. Nano Lett 2006; 6(4): 662–668, http://dx.doi.org/10.1021/nl052396o.
- Escobar-Chavez J.J., Lopez-Cervantes M., Naik A., Kalia Y.N., Quintanar-Guerrero D., Ganem-Quintanar A. Applications of thermo-reversible Pluronic F127 gels in pharmaceutical formulations. J Pharm Pharm Sci 2006; 9(3): 339–358.
- Manaspon C., Viravaidya-Pasuwat K., Pimpha N. Preparation of folate-conjugated pluronic F127/chitosan core-shell nanoparticles encapsulating doxorubicin for breast cancer treatment. Journal of Nanomaterials 2012; Article ID 593878, http://dx.doi.org/10.1155/2012/593878.
- Dumortier G., Grossiord J.L., Agnely F., Chaumeil J.C. A review of poloxamer 407 pharmaceutical and pharmacological characteristics. Pharm Res 2006; 23(12): 2709–2728, http://dx.doi.org/10.1007/s11095-006-9104-4.
- Artursson P., Lindmark T., Davis S.S., Illum L. Effect of chitosan on the permeability of monolayers of intestinal epithelial cells (Caco-2). Pharm Res 1994; 11(9): 1358–1361.
- Chitosan-based systems for biopharmaceuticals: delivery, targeting and polymer therapeutics. Edited by Sarmento B., das Neves J. Willey 2012; 583 p., http://dx.doi.org/10.1002/9781119962977.
- Ahmad M.B., Tay M.Y., Shameli K., Hussein M.Z., Lim J.J. Green synthesis and characterization of silver/chitosan/polyethylene glycol nanocomposites without any reducing agent. Int J Mol Sci 2011; 12: 4872–4884, http://dx.doi.org/10.3390/ijms12084872.
- Vasir J.K., Labhasetwar V. Quantification of the force of nanoparticle-cell membrane interactions and its influence on intracellular trafficking of nanoparticles. Biomaterials 2008; 29(31): 4244–4252, http://dx.doi.org/10.1016/j.biomaterials.2008.07.020.
- Tapia-Tapia M., Batina N. Nanoscopic characterization of the membrane surface of the HeLa cancer cells in the presence of the gold nanoparticles: an AFM study. Revista Mexicana de F′isica S 2009; 55(1): 64–67.
- He R.-Y., Su Y.-D., Cho K.-C., Lin C.-Y., Chang N.-S., Chang C.-H., Chen S.-J. Surface plasmon-enhanced two-photon fluorescence microscopy for live cell membrane imaging. Optics Express 2009; 17(8): 5987–5997, http://dx.doi.org/10.1364/OE.17.005987.
- Durr N.J., Larson T., Smith D.K., Korgel B.A., Sokolov K., Ben-Yakar A. Two-photon luminescence imaging of cancer cells using molecularly targeted gold nanorods. Nano Lett 2007; 7(4): 941–945, http://dx.doi.org/10.1021/nl062962v.
- Peckys D.B., de Jonge N. Visualizing gold nanoparticle uptake in live cells with liquid scanning transmission electron microscopy. Nano Lett 2011; 11: 1733–1738.
- Freese C., Gibson M.I., Klok H.-A., Unger R.E., Kirkpatrick C.J. Size- and coating-dependent uptake of polymer-coated gold nanoparticles in primary human dermal microvascular endothelial cells. Biomacromolecules 2012; 13: 1533–1543, http://dx.doi.org/10.1021/bm300248u.
- Coulter J.A., Jain S., Butterworth K.T., Taggart L.E., Dickson G.R., McMahon S.J., Hyland W.B., Muir M.F., Trainor C., Hounsell A.R., O’Sullivan J.M., Schettino G., Currell F.J., Hirst D.G., Prise K.M. Cell type-dependent uptake, localization, and cytotoxicity of 1.9 nm gold nanoparticles. Int J Nanomedicine 2012; 7: 2673–2685, http://dx.doi.org/10.2147/IJN.S31751.
- Guan Z., Polavarapu L., Xu Q.H. Enhanced two-photon emission in coupled metal nanoparticles induced by conjugated polymers. Langmuir 2010; 26(23): 18020–18023, http://dx.doi.org/10.1021/la103668k.
- Popov V.I., Davies H.A., Rogachevsky V.V., Patrushev I.V., Errington M.L., Gabbott P.L., Bliss T.V., Stewart M.G. Remodelling of synaptic morphology but unchanged synaptic density during late phase long-term potentiation (LTP): a serial section electron micrograph study in the dentate gyrus in the anaesthetized rat. Neuroscience 2004; 128(2): 251–262, http://dx.doi.org/10.1016/j.neuroscience.2004.06.029.
- Verma A., Stellacci F. Effect of surface properties on nanoparticle–cell interactions. Small 2010; 6(1): 12–21, http://dx.doi.org/10.1002/smll.200901158.
- Osaki F., Kanamori T., Sando S., Sera T., Aoyama Y. A quantum dot conjugated sugar ball and its cellular uptake. On the size effects of endocytosis in the subviral region. J Am Chem Soc 2004; 126(21): 6520–6521, http://dx.doi.org/10.1021/ja048792a.
- Chithrani B.D., Chan W.C. Elucidating the mechanism of cellular uptake and removal of protein-coated gold nanoparticles of different sizes and shapes. Nano Lett 2007; 7(6): 1542–1550, http://dx.doi.org/10.1021/nl070363y.
- Albanese A., Chan W.C. Effect of gold nanoparticle aggregation on cell uptake and toxicity. ACS Nano 2011; 5(7): 5478–5489, http://dx.doi.org/10.1021/nn2007496.
- Carroll J.B., Frankamp B.L., Srivastava S., Rotello V.M. Electrostatic self-assembly of structured gold nanoparticle/polyhedral oligomeric silsesquioxane (POSS) nanocomposites. J Mater Chem 2004; 14: 690–694, http://dx.doi.org/10.1039/B311423F.
- Alkilany A.M., Nagaria P.K., Hexel C.R., Shaw T.J., Murphy C.J., Wyatt M.D. Cellular uptake and cytotoxicity of gold nanorods: molecular origin of cytotoxicity and surface effects. Small 2009; 5(6): 701–708, http://dx.doi.org/10.1002/smll.200801546.
- Vaidyanathan S., Orr B.G., Banaszak Holl M.M. Detergent induction of HEK 293A cell membrane permeability measured under quiescent and superfusion conditions using whole cell patch clamp. J Phys Chem B 2014; 118(8): 2112–2123, http://dx.doi.org/10.1021/jp4124315.
- Arnida, Malugin A., Ghandehari H. Cellular uptake and toxicity of gold nanoparticles in prostate cancer cells: a comparative study of rods and spheres. J Appl Toxicol 2010; 30(2): 212–217, http://dx.doi.org/10.1002/jat.1486.
- Alkilany A.M., Murphy C.J. Toxicity and cellular uptake of gold nanoparticles: what we have learned so far? J Nanopart Res 2010; 12: 2313–2333, http://dx.doi.org/10.1007/s11051-010-9911-8.
- Conner S.D., Schmid S.L. Regulated portals of entry into the cell. Nature 2003; 422: 37–44, http://dx.doi.org/10.1038/nature01451.
- Fang C., Bhattarai N., Sun C., Zhang M. Functionalized nanoparticles with long-term stability in biological media. Small 2009; 5(14): 1637–1641, http://dx.doi.org/10.1002/smll.200801647.
- Guduru R. In situ AFM imaging of nanoparticle- cellular membrane interaction for a drug delivery study. Miami-Florida: FIU Electronic Thesis and Dissertations; 2011.