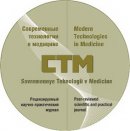
Scaffold- and Cell System-Based Bone Grafts in Tissue Engineering (Review)
The review considers the current trends in tissue engineering including maxillofacial surgery based on the use of scaffolds, autologous stem cells and bioactive substances. The authors have shown the advantages and disadvantages of basic materials used for scaffold synthesis — three-dimensional porous or fiber matrices serving as a mechanical frame for cells; among such materials there are natural polymers (collagen, cellulose, fibronectin, chitosan, alginate and agarose, fibroin), synthetic polymers (polylactide, polyglycolide, polycaprolactone, polyvinyl alcohol) and bioceramics (hydroxyapatite, tricalcium phosphate and bioactive glasses). There have been demonstrated the matrix techniques, special attention being paid to innovative technologies of rapid prototyping — the process of 3D-imaging according to a digital model. The most applicable of these techniques for biopolymers are laser stereolithography, selective laser sintering, fused deposition modeling, and 3D-printing. Great emphasis has been put on the use of bioactive substances in the process of obtaining scaffold-based bioengineered constructions — setting of stem cells on matrices before their transplantation to the defect area. Special attention has been given to a current trend of cellular biology — the application of multipotent mesenchymal stromal cells (most common marrow cells used in bone tissue regeneration), in particular, the available sources of their isolation and the variants of directed osteogenic differentiation have been presented. The review covers the characteristics and aims of bioactive substance inclusion in scaffold structure — not only to induce osteogenic differentiation, but also to attract new stem cells of a carrier, as well as promote angiogenesis.
- Chan B.P., Leong K.W. Scaffolding in tissue engineering: general approaches and tissue-specific considerations. Eur Spine J 2008; 17(Suppl 4): 467–479, http://dx.doi.org/10.1007/s00586-008-0745-3.
- Bauer T.W., Muschler G.F. Bone graft materials. An overview of the basic science. Clin Orthop Relat Res 2000; 10–27.
- Bhumiratana S., Vunjak-Novakovic G. Concise review: personalized human bone grafts for reconstructing head and face. Stem Cells Transl Med 2012; 1(1): 64–69, http://dx.doi.org/10.5966/sctm.2011-0020.
- Eppley B.L., Pietrzak W.S., Blanton M.W. Allograft and alloplastic bone substitutes: a review of science and technology for the craniomaxillofacial surgeon. J Craniofac Surg 2005; 16(6): 981–989, http://dx.doi.org/10.1097/01.scs.0000179662.38172.dd.
- Grayson W.L., Frohlich M., Yeager K., Bhumiratana S., Chan M.E., Cannizzaro C., Wan L.Q., Liu X.S., Guo X.E., Vunjak-Novakovic G. Engineering anatomically shaped human bone grafts. Proc Natl Acad Sci USA 2010; 107(8): 3299–3304, http://dx.doi.org/10.1073/pnas.0905439106.
- Stella J.A., D’Amore A., Wagner W.R., Sacks M.S. On the biomechanical function of scaffolds for engineering load-bearing soft tissues. Acta Biomater 2010; 6(7): 2365–2381, http://dx.doi.org/10.1016/j.actbio.2010.01.001.
- Chen G., Ushida T., Tateish T. Scaffold design for tissue engineering. Macromol Biosci 2002; 2: 67–77.
- Amoabediny Gh., Salehi-Nik N., Heli B. The role of biodegradable engineered scaffold in tissue engineering. In: Biomaterials Science and Engineering. Ed. by Pignatello R. InTech; 2011; p. 153–172.
- Stephanie M. Willerth, Shelly E. Sakiyama-Elbert. Approaches to neural tissue engineering using scaffolds for drug delivery. Adv Drug Deliv Rev 2007; 59(4–5): 325–338, http://dx.doi.org/10.1016/j.addr.2007.03.014.
- Ratner B.D., Bryant S.J. Biomaterials: where we have been and where we are going. Annu Rev Biomed Eng 2004; 6: 41–75.
- Ko H.F., Sfeir C., Kumta P.N. Novel synthesis strategies for natural polymer and composite biomaterials as potential scaffolds for tissue engineering. Philos Trans A Math Phys Eng Sci 2010; 368(1917): 1981–1997, http://dx.doi.org/10.1098/rsta.2010.0009.
- Rosso F., Marino G., Giordano A., Barbarisi M., Parmeggiani D., Barbarisi A. Smart materials as scaffolds for tissue engineering. J Cell Physiol 2005; 203(3): 465–470, http://dx.doi.org/10.1002/jcp.20270.
- O’Brien F.J. Biomaterials and scaffolds for tissue engineering. Materials Today 2011; 14(3): 88–95.
- Brodsky B., Persikov A.V. Molecular structure of the collagen triple helix. Adv Protein Chem 2005; 70: 301–339, http://dx.doi.org/10.1016/S0065-3233(05)70009-7.
- Persikov A.V., Ramshaw J.A.M., Brodsky B. Collagen model peptides: sequence dependence of triple-helix stability. Biopolymers 2000; 55(6): 436–450, http://dx.doi.org/10.1002/1097-0282(2000)55:6436::AID-BIP10193.0.CO;2-D.
- Bhattacharjee A., Bansa M. Collagen structure: the madras triple helix and the current scenario. IUBMB Life 2005; 57(3): 161–172, http://dx.doi.org/10.1080/15216540500090710.
- Berisio R., Vitagliano L., Mazzarella L., Zagari A. Recent progress on collagen triple helix structure, stability and assembly. Protein Pept Lett 2002; 9(2): 107–116, http://dx.doi.org/10.2174/0929866023408922.
- Jenkins C.L., Raines R.T. Insights on the conformational stability of collagen. Nat Prod Rep 2002; 19(1): 49–59.
- Gelse K., Pöschl E., Aigner T. Collagens — structure, function, and biosynthesis. Adv Drug Deliv Rev 2003; 55(12): 1531–1546, http://dx.doi.org/10.1016/j.addr.2003.08.002.
- Lee C.H., Singla A., Lee Y. Biomedical applications of collagen. Int J Pharm 2001; 221(1–2): 1–22, http://dx.doi.org/10.1016/S0378-5173(01)00691-3.
- Glowacki J., Mizuno S. Collagen scaffolds for tissue engineering. Biopolymers 2008; 89(5): 338–344, http://dx.doi.org/10.1002/bip.20871.
- Cooperman L., Michaeli D. The immunogenicity of injectable collagen. I. A 1-year prospective study. J Am Acad Dermatol 1984; 10(4): 638–646.
- DeLustro F., Condell R.A., Nguyen M.A., McPherson J.M. A comparative study of the biologic and immunologic response to medical devices derived from dermal collagen. J Biomed Mater Res 1986; 20(1): 109–120.
- Chunlin Y., Hillas P.J., Báez J.A., Nokelainen M., Balan J., Tang J., Spiro R., Polarek J.W. The application of recombinant human collagen in tissue engineering. Bio Drugs 2004; 18(2): 103–119.
- Chevallay B., Herbage D. Collagen-based biomaterials as 3D scaffold for cell cultures: applications for tissue engineering and gene therapy. Med Biol Eng Comput 2000; 38(2): 211–218.
- Orgel J.P., Wess T.J., Miller A. The in situ conformation and axial location of the intermolecular cross-linked non-helical telopeptides of type I collagen. Structure 2000; 8(2): 137–142.
- Eyre D.R., Wu J.J. Collagen cross-links. Top Curr Chem 2005; 247: 207–209.
- Entcheva E., Bien H., Yin L., Chung C.-Y., Farrell M., Kostov Y. Functional cardiac cell constructs on cellulose-base scaffolding. Biomaterials 2004; 25(26): 5753–5762, http://dx.doi.org/10.1016/j.biomaterials.2004.01.024.
- Shi Q., Li Y., Sun J., Zhang H., Chen L., Chen B., Yang H., Wang Z. The osteogenesis of bacterial cellulose scaffold loaded with bone morphogenetic protein-2. Biomaterials 2012; 33(28): 6644–6649, http://dx.doi.org/10.1016/j.biomaterials.2012.05.071.
- Zaborowska M., Bodin A., Bäckdahl H., Popp J., Goldstein A., Gatenholm P. Microporous bacterial cellulose as a potential scaffold for bone regeneration. Acta Biomater 2010; 6(7): 2540–2547, http://dx.doi.org/10.1016/j.actbio.2010.01.004.
- Müller F.A., Müller L., Hofmann I., Greil P., Wenzel M.M., Staudenmaier R. Cellulose-based scaffold materials for cartilage tissue engineering. Biomaterials 2006; 27(21): 3955–3963, http://dx.doi.org/10.1016/j.biomaterials.2006.02.031.
- Backdahl H., Helenius G., Bodin A., Nannmark U., Johansson B.R., Risberg B. Mechanical properties of bacterial cellulose and interactions with smooth muscle cells. Biomaterials 2006; 27: 2141–2149.
- Helenius G., Bäckdahl H., Bodin A., Nannmark U., Gatenholm P., Risberg B. In vivo biocompatibility of bacterial cellulose. J Biomed Mater Res A 2006; 76A(2): 431–438, http://dx.doi.org/10.1002/jbm.a.30570.
- Liu S., Tao D., Zhang L. Cellulose scaffold: a green template for the controlling synthesis of magnetic inorganic nanoparticles. Powder Technology 2012; 217: 502–509, http://dx.doi.org/10.1016/j.powtec.2011.11.010.
- Biokhimiya [Biochemistry]. Pod red. Severina E.S. [Severin E.S. (editor)]. Moscow: GEOTAR-Media; 2003; 715 p.
- Ebner R., Lackner J.M., Waldhauser W., Major R., Czarnowska E., Kustosz R., Lacki P., Major B. Biocompatible TiN-based novel nanocrystalline films. Bulletin of the Polish Academy of Science 2006; 2: 167–173.
- Khor E., Lim L.Y. Implantable applications of chitin and chitosan. Biomaterials 2003; 24(13): 2339–2349, http://dx.doi.org/10.1016/S0142-9612(03)00026-7.
- Venkatesan J., Kim S.K. Chitosan composites for bone tissue engineering — an overview. Mar Drugs 2010; 8(8): 2252–2266, http://dx.doi.org/10.3390/md8082252.
- No H.K., Park N.Y., Lee S.H., Meyers S.P. Antibacterial activity of chitosans and chitosan oligomers with different molecular weights. Int J Food Microbiol 2002; 74(1–2): 65–72.
- Costa-Pinto A.R., Reis R.L., Neves N.M. Scaffolds based bone tissue engineering: the role of chitosan. Tissue Eng Part B Rev 2011; 17(5): 331–347, http://dx.doi.org/10.1089/ten.teb.2010.0704.
- Rabea E.I., Badawy M.E.T., Stevens C.V., Smagghe G., Steurbaut W. Chitosan as antimicrobial agent: applications and mode of action. Biomacromolecules 2003; 4(6): 1457–1465, http://dx.doi.org/10.1021/bm034130m.
- Sun J., Tan H. Alginate-based biomaterials for regenerative medicine applications. Materials 2013, 6(4): 1285–1309.
- Mano J.F., Silva G.A., Azevedo H.S., Malafaya P.B., Sousa R.A., Silva S.S., Boesel L.F., Oliveira J.M., Santos T.C., Marques A.P., Neves N.M., Reis R.L. Natural origin biodegradable systems in tissue engineering and regenerative medicine: present status and some moving trends. J R Soc Interface 2007; 4(17): 999–1030, http://dx.doi.org/10.1098/rsif.2007.0220.
- Awad H.A., Wickham M.Q., Leddy H.A., Gimble J.M., Guilak F. Chondrogenic differentiation of adipose-derived adult stem cells in agarose, alginate, and gelatin scaffolds. Biomaterials 2004; 25(16): 3211–3222, http://dx.doi.org/10.1016/j.biomaterials.2003.10.045.
- Willerth S.M., Sakiyama-Elbert S.E. Combining stem cells and biomaterial scaffolds for constructing tissues and cell delivery. StemBook. Cambridge (MA): Harvard Stem Cell Institute; 2008.
- Kim H.J., Kim U.J., Kim H.S., Li C., Wada M., Leisk G.G., Kaplan D.L. Bone tissue engineering with premineralized silk scaffolds. Bone 2008; 42(6): 1226–1234, http://dx.doi.org/10.1016/j.bone.2008.02.007.
- Correia C., Bhumiratana S., Yan L.P., Oliveira A.L., Gimble J.M., Rockwood D., Kaplan D.L., Sousa R.A., Reis R.L., Vunjak-Novakovic G. Development of silk-based scaffolds for tissue engineering of bone from human adipose-derived stem cells. Acta Biomater 2012; 8(7): 2483–2492, http://dx.doi.org/10.1016/j.actbio.2012.03.019.
- Mandal B.B., Grinberg A., Gil E.S., Panilaitis B., Kaplan D.L. High-strength silk protein scaffolds for bone repair. Proc Natl Acad Sci USA 2012; 109(20): 7699–7704, http://dx.doi.org/10.1073/pnas.1119474109.
- Garg T., Singh O., Arora S., Murthy R. Scaffold: a novel carrier for cell and drug delivery. Crit Rev Ther Drug Carrier Syst 2012; 29(1): 1–63, http://dx.doi.org/10.1615/CritRevTherDrugCarrierSyst.v29.i1.10.
- Pavia F.C., Rigogliuso S., La Carrubba V., Mannella G.A., Ghersi G., Brucato V. Poly lactic acid based scaffolds for vascular tissue engineering. Chemical Engineering Transactions 2012; 27: 409–414.
- Xiao L., Wang B., Yang G., Gauthier M. Poly(lactic acid)-based biomaterials: synthesis, modification and applications. In: Biomedical science, engineering and technology. Ed. by Dhanjoo N. Ghista. InTech; 2012; p. 247–282, http://dx.doi.org/10.5772/23927.
- Kallela I., Tulamo R.-M., Hietanen J., Pohjonen T., Suuronen R., Lindqvist C. Fixation of mandibular body osteotomies using biodegradable amorphous self-reinforced (70L:30DL) polylactide or metal lag screws: an experimental study in sheep. J Craniomaxillofac Surg 1999; 27(2): 124–133.
- Lyons F., Partap S., O’Brien F.J. Part 1: scaffolds and surfaces. Technol Health Care 2008; 16(4): 305–317.
- Kerimoğlu O., Alarçin E. Poly(lactic-co-glycolic acid) based drug delivery devices for tissue engineering and regenerative medicine. ANKEM Derg 2012; 26(2): 86–98, http://dx.doi.org/10.5222/ankem.2012.086.
- Gloria A., Causa F., Russo T., Battista E., Della Moglie R., Zeppetelli S., De Santis R., Netti P.A., Ambrosio L. Three-dimensional poly(ε-caprolactone) bioactive scaffolds with controlled structural and surface properties. Biomacromolecules 2012; 13(11): 3510–3521, http://dx.doi.org/10.1021/bm300818y.
- Mei N., Chen G., Zhou P., Chen X., Shao Z.Z., Pan L.F., Wu C.G. Biocompatibility of poly(ε-caprolactone) scaffold modified by chitosan — the fibroblasts proliferation in vitro. J Biomater Appl 2005; 19(4): 323–339, http://dx.doi.org/10.1177/0885328205048630.
- Zhu Y., Gao C.Y., Shen J.Y. Surface modification of polycaprolactone with poly(methacrylic acid) and gelatin covalent immobilization for promoting its cytocompatibility. Biomaterials 2002; 23(24): 4889–4895, http://dx.doi.org/10.1016/S0142-9612(02)00247-8.
- Ng K.W., Wanivenhaus F., Chen T., Hsu H.C., Allon A.A., Abrams V.D., Torzilli P.A., Warren R.F., Maher S.A. A novel macroporous polyvinyl alcohol scaffold promotes chondrocyte migration and interface formation in an in vitro cartilage defect model. Tissue Eng Part A 2012; 18(11–12): 1273–1281, http://dx.doi.org/10.1089/ten.TEA.2011.0276.
- Shuai C., Mao Z., Lu H., Nie Y., Hu H., Peng S. Fabrication of porous polyvinyl alcohol scaffold for bone tissue engineering via selective laser sintering. Biofabrication 2013; 5(1): 015014, http://dx.doi.org/10.1088/1758-5082/5/1/015014.
- Bellucci D., Sola A., Cannillo V. A revised replication method for bioceramic scaffolds. Bioceram Dev Appl 2011; 1: 110401.
- Leukers B., Gülkan H., Irsen S.H., Milz S., Tille C., Schieker M., Seitz H. Hydroxyapatite scaffolds for bone tissue engineering made by 3D printing. J Mater Sci Mater Med 2005; 16(12): 1121–1124, http://dx.doi.org/10.1007/s10856-005-4716-5.
- Marra K.G., Szem J.W., Kumta P.N., DiMilla P.A., Weiss L.E. In vitro analysis of biodegradable polymer blend/hydroxyapatite composites for bone tissue engineering. J Biomed Mater Res 1999; 47(3): 324–335, http://dx.doi.org/10.1002/(SICI)1097-4636(19991205)47:3324::AID-JBM63.0.CO;2-Y.
- Zhao H., Wang G., Hu S., Cui J., Ren N., Liu D., Liu H., Cao C., Wang J., Wang Z. In vitro biomimetic construction of hydroxyapatite-porcine acellular dermal matrix composite scaffold for MC3T3-E1 preosteoblast culture. Tissue Eng Part A 2011; 17(5–6): 765–776, http://dx.doi.org/10.1089/ten.TEA.2010.0196.
- Sulaiman S.B., Keong T.K., Cheng C.H., Saim A.B., Idrus R.B. Tricalcium phosphate/hydroxyapatite (TCP-HA) bone scaffold as potential candidate for the formation of tissue engineered bone. Indian J Med Res 2013; 137(6): 1093–1101.
- Tarafder S., Balla V.K., Davies N.M., Bandyopadhyay A., Bose S. Microwave-sintered 3D printed tricalcium phosphate scaffolds for bone tissue engineering. J Tissue Eng Regen Med 2013; 7(8): 631–641, http://dx.doi.org/10.1002/term.555.
- Farooq I., Imran Z., Farooq U., Leghari A., Ali H. Bioactive glass: a material for the future. World J Dent 2012; 3(2): 199–201.
- Fu Q., Saiz E., Rahaman M.N., Tomsia A.P. Bioactive glass scaffolds for bone tissue engineering: state of the art and future perspectives. Mater Sci Eng C 2011; 31(7): 1245–1256, http://dx.doi.org/10.1016/j.msec.2011.04.022.
- Boccaccini A.R., Blaker J.J. Bioactive composite materials for tissue engineering scaffolds. Expert Rev Med Devices 2005; 2(3): 303–317, http://dx.doi.org/10.1586/17434440.2.3.303.
- Gleeson J.P., O’Brien F.J. Composite scaffolds for orthopaedic regenerative medicine. Advances in Composite Materials for Medicine and Nanotechnology 2011; 10: 33–59.
- Lu T., Li Y., Chen T. Techniques for fabrication and construction of three-dimensional scaffolds for tissue engineering. Int J Nanomedicine 2013; 8: 337–350, http://dx.doi.org/10.2147/IJN.S38635.
- Woods I., Flanagan T.C. Electrospinning of biomimetic scaffolds for tissue-engineered vascular grafts: threading the path. Expert Rev Cardiovasc Ther 2014; 12(7): 815–832, http://dx.doi.org/10.1586/14779072.2014.925397.
- Liang D., Hsiao B.S., Chu B. Functional electrospunnanofibrous scaffolds for biomedical applications. Adv Drug Deliv Rev 2007; 59(14): 1392–1412, http://dx.doi.org/10.1016/j.addr.2007.04.021.
- Akbarzadeh R., Yousefi A.M. Effects of processing parameters in thermally induced phase separation technique on porous architecture of scaffolds for bone tissue engineering. J Biomed Mater Res B Appl Biomater 2014; 102(6): 1304–1315, http://dx.doi.org/10.1002/jbm.b.33101.
- Smith L.A., Beck J.A., Ma P.X. Nano fibrous scaffolds and their biologicaleffects. In: Tissue cell and organ engineering. Ed. by Kumar C. Wiley-VCH, Weinheim; 2006; р. 188–215.
- Li Q., Reed D.A., Min L., Gopinathan G., Li S., Dangaria S.J., Li L., Geng Y., Galang M.T., Gajendrareddy P., Zhou Y., Luan X., Diekwisch T.G. Lyophilized platelet-rich fibrin (PRF) promotes craniofacial bone regeneration through Runx2. Int J Mol Sci 2014; 15(5): 8509–8525, http://dx.doi.org/10.3390/ijms15058509.
- Boland E.D., Espy P.G., Bowlin G.L. Tissue engineering scaffolds. In: Encyclopaedia of biomaterials and biomedical engineering. Eds. by Wenk G.E., Bowlin G.L. USA; 2004; p. 1633–1635.
- Thein-Han W., Xu H.H. Prevascularization of a gas-foaming macroporous calcium phosphate cement scaffold via coculture of human umbilical vein endothelial cells and osteoblasts. Tissue Eng Part A 2013; 19(15–16): 1675–1685, http://dx.doi.org/10.1089/ten.TEA.2012.0631.
- Ikada Y. Scope of tissue engineering. In: Tissue engineering: fundamental and applications. Ed. by Ikada Y. USA: Academic press. 2006; p. 29.
- Ma L. X., Peter X. Polymeric scaffolds for bone tissue engineering. Ann Biomed Eng 2004; 32(3): 477–486.
- Subia B., Kundu J., Kundu S.C. Biomaterial scaffold fabrication techniques for potential tissue engineering applications. In: Tissue Engineering. Ed. by Eberli D. InTech; 2010; p. 141–159.
- Gelinsky M., Welzel P.B., Simon P., Bernhardt A., König U. Porous three-dimensional scaffolds made of mineralized collagen: preparation and properties of a biomimetic nanocomposite material for tissue engineering of bone. Chemical Engineering Journal 2008; 137: 84–96.
- Bose S., Vahabzadeh S., Bandyopadhyay A. Bone tissue engineering using 3D printing. Mater Today 2013; 16(12): 496–504, http://dx.doi.org/10.1016/j.mattod.2013.11.017.
- Li X., Cui R., Sun L., Aifantis K.E., Fan Y., Feng Q., Cui F., Watari F. 3D-printed biopolymers for tissue engineering application. International Journal of Polymer Science 2014; Article ID 829145.
- Hollister S.J. Porous scaffold design for tissue engineering. Nat Mater 2005; 4(7): 518–524, http://dx.doi.org/10.1038/nmat1421.
- Melchels F.P., Feijen J., Grijpma D.W. A review on stereolithography and its applications in biomedical engineering. Biomaterials 2010; 31(24): 6121–6130, http://dx.doi.org/10.1016/j.biomaterials.2010.04.050.
- Skoog S.A., Goering P.L., Narayan R.J. Stereolithography in tissue engineering. J Mater Sci Mater Med 2014; 25(3): 845–856, http://dx.doi.org/10.1007/s10856-013-5107-y.
- Kang H.W., Cho D.W. Development of an indirect stereolithography technology for scaffold fabrication with a wide range of biomaterial selectivity. Tissue Eng Part C Methods 2012; 18(9): 719–29, http://dx.doi.org/10.1089/ten.TEC.2011.0621.
- Leach J.B., Bivens K.A., Collins C.N., Schmidt C.E. Development of photocrosslinkable hyaluronic acidpolyethylene glycol-peptide composite hydrogels for soft tissue engineering. J Biomed Mater Res A 2004; 70(1): 74-82, http://dx.doi.org/10.1002/jbm.a.30063.
- Narayan R.J., Doraiswamy A., Chrisey D.B., Chichkov B.N. Medical prototyping using two photon polymerization. Mater Today 2010; 13(12): 42–48, http://dx.doi.org/10.1016/S1369-7021(10)70223-6.
- Kurselis K., Kiyan R., Bagratashvili V.N., Popov V.K., Chichkov B.N. 3D fabrication of all-polymer conductive microstructures by two photon polymerization. Opt Express 2013; 21(25): 31029–3135, http://dx.doi.org/10.1364/OE.21.031029.
- Obata K., El-Tamer A., Koch L., Hinze U., Chichkov B.N. High-aspect 3D two-photon polymerization structuring with widened objective working range (WOW-2PP). Light: Science & Applications 2013; 2: e116.
- Mazzoli A. Selective laser sintering in biomedical engineering. Med Biol Eng Comput 2013; 51(3): 245–256, http://dx.doi.org/10.1007/s11517-012-1001-x.
- Capel A.J., Edmondson S., Christie S.D., Goodridge R.D., Bibb R.J., Thurstans M. Design and additive manufacture for flow chemistry. Lab Chip 2013; 13(23): 4583–4590, http://dx.doi.org/10.1039/c3lc50844g.
- Antonov E.N., Bagratashvili V.N., Whitaker M.J., Barry J.J., Shakesheff K.M., Konovalov A.N., Popov V.K., Howdle S.M. Three-dimensional bioactive and biodegradable scaffolds fabricated by surface-selective laser sintering. Adv Mater 2005; 17(3): 327–330, http://dx.doi.org/10.1002/adma.200400838.
- Bukharova T.B., Antonov E.N., Popov V.K., Fatkhudinov T.Kh., Popova A.V., Volkov A.V., Bochkova S.A., Bagratashvili V.N., Gol’dshtein D.V. Biocompatibility of tissue engineering constructions from porous polylactide carriers obtained by the method of selective laser sintering and bone marrow-derived multipotent stromal cells. Bull Exp Biol Med 2010; 149(1): 148–153, http://dx.doi.org/10.1007/s10517-010-0895-2.
- Schantz J.T., Hutmacher D.W., Chim H., Ng K.W., Lim T.C., Teoh S.H. Induction of ectopic bone formation by using human periosteal cells in combination with a novel scaffold technology. Cell Transplant 2002; 11(2): 125–138.
- Yeong W.Y., Chua C.K., Leong K.F., Chandrasekaran M. Rapid prototyping in tissue engineering: challenges and potential. Trends Biotechnol 2004; 22(12): 643–652, http://dx.doi.org/10.1016/j.tibtech.2004.10.004.
- Zein I., Hutmacher D.W., Tan K.C., Teoh S.H. Fused deposition modeling of novel scaffold architectures for tissue engineering applications. Biomaterials 2002; 23(4): 1169–1185.
- Samar J.K., Susmita B., Howard L.H., Amit B. Development of controlled porosity polymerceramic composite scaffolds via fused deposition modeling. Mater Sci Eng C 2003; 23: 611–620, http://dx.doi.org/10.1016/S0928-4931(03)00052-3.
- Endres M., Hutmacher D.W., Salgado A.J., Kaps C., Ringe J., Reis R.L., Sittinger M., Brandwood A., Schantz J.T. Osteogenic induction of human bone marrow-derived mesenchymal progenitor cells in novel synthetic polymer-hydrogel matrices. Tissue Eng 2003; 9(4): 689–702, http://dx.doi.org/10.1089/107632703768247386.
- Utela B., Storti D., Anderson R., Ganter M. A review of process development steps for new material systems in three dimensional printing (3DP). Journal of Manufacturing Processes 2008; 96–104.
- Caplan A.I. Adult mesenchymal stem cells for tissue engineering versus regenerative medicine. J Cell Physiol 2007; 213(2): 341–347, http://dx.doi.org/10.1002/jcp.21200.
- Makino S., Fukuda K., Miyoshi S., Konishi F., Kodama H., Pan J., Sano M., Takahashi T., Hori S., Abe H., Hata J., Umezawa A., Ogawa S. Cardiomyocytes can be generated from marrow stromal cells in vitro. J Clin Invest 1999; 103: 697–705, http://dx.doi.org/10.1172/JCI5298.
- Kopen G.C., Prockop D.J., Phinney D.G. Marrow stromal cells migrate throughout forebrain and cerebellum, and they differentiate into astrocytes after injection into neonatal mouse brains. Proc Natl Acad Sci USA 1999; 96(19): 10711–10716, http://dx.doi.org/10.1073/pnas.96.19.10711.
- Strioga M., Viswanathan S., Darinskas A., Slaby O., Michalek J. Same or not the same? Comparison of adipose tissue-derived versus bone marrow-derived mesenchymal stem and stromal cells. Stem Cells Dev 2012; 21(14): 2724–2752, http://dx.doi.org/10.1089/scd.2011.0722.
- Gronthos S., Mankani M., Brahim J., Robey P.G., Shi S. Postnatal human dental pulp stem cells (DPSCs) in vitro and in vivo. Proc Natl Acad Sci USA 2000; 97(25): 13625–13630, http://dx.doi.org/10.1073/pnas.240309797.
- Izadpanah R., Trygg C., Patel B., Kriedt C., Dufour J. Biologic properties of mesenchymal stem cells derived from bone marrow and adipose tissue. J Cell Biochem 2006; 99: 1285–1297, http://dx.doi.org/10.1002/jcb.20904.
- Dominici M., Le Blanc K., Mueller I., Slaper-Cortenbach I., Marini F., Krause D., Deans R., Keating A., Prockop Dj., Horwitz E. Minimal criteria for defining multipotent mesenchymal stromal cells. The International Society for Cellular Therapy position statement. Cytotherapy 2006; 8(4): 315–317.
- Peng L., Jia Z., Yin X., Zhang X., Liu Y., Chen P., Ma K., Zhou C. Comparative analysis of mesenchymal stem cells from bone marrow, cartilage, and adipose tissue. Stem Cells Dev 2008; 17(4): 761–773, http://dx.doi.org/10.1089/scd.2007.0217.
- Roemeling-van Rhijn M., Khairoun M., Korevaar S.S., Lievers E., Leuning D.G., Ijzermans J.N., Betjes M.G., Gene¬ver P.G., van Kooten C., de Fijter H.J., Rabelink T.J., Baan C.C., Weimar W., Roelofs H., Hoogduijn M.J., Reinders M.E. Human bone marrow- and adipose tissue-derived mesenchymal stromal cells are immunosuppressive in vitro and in a humanized allograft rejection model. J Stem Cell Res Ther 2013; 6(1): 20780, http://dx.doi.org/10.4172/2157-7633.S6-001.
- Rath S.N., Nooeaid P., Arkudas A., Beier J.P., Strobel L.A., Brandl A., Roether J.A., Horch R.E., Boccaccini A.R., Kneser U. Adipose- and bone marrow-derived mesenchymal stem cells display different osteogenic differentiation patterns in 3D bioactive glass-based scaffolds. J Tissue Eng Regen Med 2013, http://dx.doi.org/10.1002/term.1849. [Epub ahead of print].
- Lee R.H., Kim B., Choi I., Kim H., Choi H.S., Suh K., Bae Y.C., Jung J.S. Characterization and expression analysis of mesenchymal stem cells from human bone marrow and adipose tissue. Cell Physiol Biochem 2004; 14(4–6): 311–324, http://dx.doi.org/10.1159/000080341.
- Im G.I., Shin Y.W., Lee K.B. Do adipose tissue-derived mesenchymal stem cells have the same osteogenic and chondrogenic potential as bone marrow-derived cells? Osteoarthritis Cartilage 2005; 13(10): 845–853, http://dx.doi.org/10.1016/j.joca.2005.05.005.
- Noёl D., Caton D., Roche S., Bony C., Lehmann S., Casteilla L., Jorgensen C., Cousin B. Cell specific differences between human adipose-derived and mesenchymal-stromal cells despite similar differentiation potentials. Exp Cell Res 2008; 314(7): 1575–1584, http://dx.doi.org/10.1016/j.yexcr.2007.12.022.
- Sakaguchi Y., Sekiya I., Yagishita K., Muneta T. Comparison of human stem cells derived from various mesenchymal tissues: superiority of synovium as a cell source. Arthritis Rheum 2005; 52(8): 2521–2529, http://dx.doi.org/10.1002/art.21212.
- Kern S., Eichler H., Stoeve J., Klüter H., Bieback K. Comparative analysis of mesenchymal stem cells from bone marrow, umbilical cord blood, or adipose tissue. Stem Cells 2006; 24(5): 1294–1301, http://dx.doi.org/10.1634/stemcells.2005-0342.
- De Ugarte D.A., Morizono K., Elbarbary A., Alfonso Z., Zuk P.A., Zhu M., Dragoo J.L., Ashjian P., Thomas B., Benhaim P., Chen I., Fraser J., Hedrick M.H. Comparison of multi-lineage cells from human adipose tissue and bone marrow. Cells, Tissues, Organs 2003; 174(3): 101–109, http://dx.doi.org/10.1159/000071150.
- Tuan R.S., Boland G., Tuli R. Adult mesenchymal stem cells and cell-based tissue engineering. Arthritis Res Ther 2003; 5(1): 32–45.
- Birmingham E., Niebur G.L., McHugh P.E., Shaw G., Barry F.P., McNamara L.M. Osteogenic differentiation of mesenchymal stem cells is regulated by osteocyte and osteoblast cells in a simplified bone niche. Eur Cell Mater 2012; 23: 13–27.
- Mikami Y., Lee M., Irie S., Honda M.J. Dexamethasone modulates osteogenesis and adipogenesis with regulation of osterix expression in rat calvaria-derived cells. J Cell Physiol 2011; 226(3): 739–748, http://dx.doi.org/10.1002/jcp.22392.
- Kaveh K., Ibrahim R., Bakar M.Z.A., Ibrahim T.A. Mesenchymal stem cells, osteogenic lineage and bone tissue engineering: a review. Journal of Animal and Veterinary Advances 2011; 10(17): 2317–2330, http://dx.doi.org/10.3923/javaa.2011.2317.2330.
- Qing W., Guang-Xing C., Lin G., Liu Y. The osteogenic study of tissue engineering bone with BMP2 and BMP7 gene-modified rat adipose-derived stem cell. J Biomed Biotechnol 2012; 2012: 410879, http://dx.doi.org/10.1155/2012/410879.
- Hofmann S., Garcia-Fuentes M. Bioactive scaffolds for the controlled formation of complex skeletal tissues. In: Regenerative medicine and tissue engineering: cells and biomaterials. Ed. by Eberli D. Rijeka: InTech; 2011; p. 393–432, http://dx.doi.org/10.5772/22061.
- Sundelacruz S., Kaplan D.L. Stem cell-and scaffold-based tissue engineering approaches to osteochondral regenerative medicine. Semin Cell Dev Biol 2009; 20(6): 646–655, http://dx.doi.org/10.1016/j.semcdb.2009.03.017.
- Luginbuehl V., Meinel L., Merkle H.P., Gander B. Localized delivery of growth factors for bone repair. Eur J Pharm Biopharm 2004; 58(2): 197–208, http://dx.doi.org/10.1016/j.ejpb.2004.03.004.
- Liu H., Zhang L., Shi P., Zou Q., Zuo Y., Li Y. Hydroxyapatite/polyurethane scaffold incorporated with drug-loaded ethyl cellulose microspheres for bone regeneration. J Biomed Mater Res B Appl Biomater 2010; 95(1): 36–46, http://dx.doi.org/10.1002/jbm.b.31680.
- Porter J.R., Ruckh T.T., Popat K.C. Bone tissue engineering: a review in bone biomimetics and drug delivery strategies. Biotechnol Prog 2009; 25(6): 1539–1560, http://dx.doi.org/10.1002/btpr.246.
- Zhu X.H., Tabata Y., Wang C.H., Tong Y.W. Delivery of basic fibroblast growth factor from gelatin microsphere scaffold for the growth of human umbilical vein endothelial cells. Tissue Eng Part A 2008; 14(12): 1939–1947, http://dx.doi.org/10.1089/ten.tea.2007.0346.
- Royce S.M., Askari M., Marra K.G. Incorporation of polymer microspheres within fibrin scaffolds for the controlled delivery of FGF-1. J Biomater Sci Polym Ed 2004; 15(10): 1327–1336.