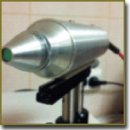
Multimodal Optical Coherence Tomography in Visualization of Brain Tissue Structure at Glioblastoma (Experimental Study)
The aim of the investigation was to evaluate the performance of multimodal OCT (MM OCT) for differential diagnostics of normal and diseased brain tissue using an experimental model of glioblastoma.
Materials and Methods. The spectral domain MM OCT device developed at the Institute of Applied Physics of the Russian Academy of Sciences (Nizhny Novgorod, Russia) was used for the study. It provides two modes of investigation: cross-polarization OCT (CP OCT) and microangiographic OCT (MA OCT). The instrument features the following characteristics: rate of information gathering — 20,000 A-scans per second; wavelength — 1.3 µm; shot size — ~4×2 mm; lateral resolution — 20 µm; axial resolution — 10–15 µm. The OCT investigation was performed on an experimental 101.8 rat brain glioblastoma tumor model inoculated and maintained in the Research Institute of Human Morphology. To evaluate the signal parameters typical of the tumor and of normal brain tissue, CP OCT and MA OCT images were compared with histological specimens (stained with hematoxylin and eosin). Analysis of the MA OCT images was performed on the basis of comparison with the findings of ZOOM-microscopy.
Results. The model of the rat 101.8 glioblastoma helped to identify links between CP OCT images of areas of brain tissue and their morphological structure. We performed a comparative evaluation of the signals from the glial tumor and from normal brain tissue. MA OCT allowed the visualization of the blood vessels both in the tumor and in the normal brain tissues, revealing changes in the form and sizes typical of the tumor vessels.
Conclusion. ММ OCT is an innovative technology with potential for use in intraoperational diagnoses of glial tumors of the brain. The ability to combine several modes of investigation enables information to be obtained simultaneously about the structure of the tissues and about any peculiarities of the structure of the different elements of their microvascular network.
- Witiw C.D., Nathan V., Bernstein M. Economics, innovation, and quality improvement in neurosurgery. Neurosurg Clin N Am 2015; 26(2): 197–205, http://dx.doi.org/10.1016/j.nec.2014.11.003.
- Moiseev A.A., Gelikonov G.V., Terpelov D.A., Shilyagin P.A., Gelikonov V.M. Improvement of lateral resolution of spectral domain optical coherence tomography images in out-of-focus regions with holographic data processing techniques. Quantum Electronics 2014; 44(8): 732–739, http://dx.doi.org/10.1070/QE2014v044n08ABEH015492.
- Gelikonov V.M., Gelikonov G.V., Shilyagin P.A. Linear-wavenumber spectrometer for high-speed spectral-domain optical coherence tomography. Optics and Spectroscopy 2009; 106(3): 459–465, http://dx.doi.org/10.1134/s0030400x09030242.
- Gelikonov V.M., Gelikonov G.V., Kasatkina I.V., Terpelov D.A., Shilyagin P.A. Coherent noise compensation in spectral-domain optical coherence tomography. Optics and Spectroscopy 2009; 106(6): 895–900, http://dx.doi.org/10.1134/s0030400x09060174.
- Gelikonov V.M., Gelikonov G.V., Terpelov D.A., Shabanov D.V., Shilyagin P.A. Suppression of image autocorrelation artefacts in spectral domain optical coherence tomography and multiwave digital holography. Quantum Electronics 2012; 42(5): 390–393, http://dx.doi.org/10.1070/QE2012v042n05ABEH014852.
- Shilyagin P.A., Gelikonov G.V., Gelikonov V.M., Moiseev A.A., Terpelov D.A. Achromatic registration of quadrature components of the optical spectrum in spectral domain optical coherence tomography. Quantum Electronics 2014; 44(7): 664–669, http://dx.doi.org/10.1070/QE2014v044n07ABEH015465.
- Gelikonov V.M., Gelikonov G.V. New approach to cross-polarized optical coherence tomography based on orthogonal arbitrarily polarized modes. Laser Physics Letters 2006; 3(9): 445–451, http://dx.doi.org/10.1002/lapl.200610030.
- Matveev L.A., Zaitsev V.Y., Gelikonov G.V., Matveyev A.L., Moiseev A.A., Ksenofontov S.Y., Gelikonov V.M., Sirotkina M.A., Gladkova N.D., Demidov V., Vitkin A. Hybrid M-mode-like OCT imaging of three-dimensional microvasculature in vivo using reference-free processing of complex valued B-scans. Optics Letters 2015; 40(7): 1472–1475, http://dx.doi.org/10.1364/ol.40.001472.
- Khalansky A.S., Kondakova L.I. Transplanted rat glioma 101.8.I. Biological characteristics. Klinicheskaya i eksperimental’naya morfologiya 2013; 4(8): 63–68.
- Barth R.F., Kaur B. Rat brain tumor models in experimental neuro-oncology: the C6, 9L, T9, RG2, F98, BT4C, RT-2 and CNS-1 gliomas. J Neurooncol 2009; 94(3): 299–312, http://dx.doi.org/10.1007/s11060-009-9875-7.
- Goldey G.J., Roumis D.K., Glickfeld L.L., Kerlin A.M., Reid R.C., Bonin V., Schafer D.P., Andermann M.L. Removable cranial windows for long-term imaging in awake mice. Nat Protoc 2014; 9(11): 2515–2538, http://dx.doi.org/10.1038/nprot.2014.165.
- Mostany R., Portera-Cailliau C. A craniotomy surgery procedure for chronic brain imaging. J Vis Exp 2008; 12, http://dx.doi.org/10.3791/680.
- Park K., You J., Du C., Pan Y. Cranial window implantation on mouse cortex to study microvascular change induced by cocaine. Quant Imaging Med Surg 2015; 5(1): 97–107, http://dx.doi.org/10.3978/j.issn.2223-4292.2014.11.31.
- Keiner D., Heimann A., Kronfeld A., Sommer C., Mueller-Forell W., Kempski O., Oertel J. Towards a glioma model for surgical technique evaluation in the rat. Br J Neurosurg 2014; 28(1): 86–92, http://dx.doi.org/10.3109/02688697.2013.804489.
- Prikaz Minzdravsotsrazvitiya RF ot 23.08.2010 №708n “Ob utverzhdenii Pravil laboratornoy praktiki” [Ministry of Health and Social Development of the Russian Federation Decree No.708n as of Aug 23, 2010 “On Good Laboratory Practices”].
- Mezhdunarodnye rekomendatsii (eticheskiy kodeks) po provedeniyu mediko-biologicheskikh issledovaniy s ispol’zovaniem zhivotnykh [International guiding principles (ethical code) for biomedical research involving animals]. 1985.
- Sanai N., Berger M.S. Glioma extent of resection and its impact on patient outcome. Neurosurgery 2008; 62(4): 753–766, http://dx.doi.org/10.1227/01.neu.0000318159.21731.cf.
- Sanai N., Polley M.Y., McDermott M.W., Parsa A.T., Berger M.S. An extent of resection threshold for newly diagnosed glioblastomas. J Neurosurg 2011; 115(1): 3–8, http://dx.doi.org/10.3171/2011.2.jns10998.
- Anokhina Yu.E., Gaidar B.V., Martynov B.V., Svistov D.V., Papayan G.V., Grigorievsky D.I. Prognostic significance of surgery volume under fluorescent intraoperative diagnostic applications in patients with malignant brain gliomas. Vestnik rossiyskoy voenno-meditsinskoy akademii 2014; 1: 19–24.
- Stummer W., Reulen H.J., Meinel T., Pichlmeier U., Schumacher W., Tonn J.C., Rohde V., Oppel F., Turowski B., Woiciechowsky C., Franz K., Pietsch T. Extent of resection and survival in glioblastoma multiforme: identification of and adjustment for bias. Neurosurgery 2008; 62(3): 564–576, http://dx.doi.org/10.1227/01.neu.0000317304.31579.17.
- McGirt M.J., Chaichana K.L., Gathinji M., Attenello F.J., Than K., Olivi A., Weingart J.D., Brem H., Quiñones-Hinojosa A.R. Independent association of extent of resection with survival in patients with malignant brain astrocytoma. J Neurosurg 2009; 110(1): 156–162, http://dx.doi.org/10.3171/2008.4.17536.
- Kuhnt D., Becker A., Ganslandt O., Bauer M., Buchfelder M., Nimsky C. Correlation of the extent of tumor volume resection and patient survival in surgery of glioblastoma multiforme with high-field intraoperative MRI guidance. Neuro Oncol 2011; 13(12): 1339–1348, http://dx.doi.org/10.1093/neuonc/nor133.
- Sanai N., Polley M.Y., McDermott M.W., Parsa A.T., Berger M.S. An extent of resection threshold for newly diagnosed glioblastomas. J Neurosurg 2011; 115(1): 3–8, http://dx.doi.org/10.3171/2011.2.JNS10998.
- Böhringer H.J., Boller D., Leppert J., Knopp U., Lankenau E., Reusche E., Hüttmann G., Giese A. Time-domain and spectral-domain optical coherence tomography in the analysis of brain tumor tissue. Lasers Surg Med 2006; 38(6): 588–597, http://dx.doi.org/10.1002/lsm.20353.
- Böhringer H.J., Lankenau E., Stellmacher F., Reusche E., Hüttmann G., Giese A. Imaging of human brain tumor tissue by near-infrared laser coherence tomography. Acta Neurochir (Wien) 2009; 151(5): 507–517, http://dx.doi.org/10.1007/s00701-009-0248-y.
- Kut C., Chaichana K.L., Xi J., Raza S.M., Ye X., McVeigh E.R., Rodriguez F.J., Quiñones-Hinojosa A., Li X. Detection of human brain cancer infiltration ex vivo and in vivo using quantitative optical coherence tomography. Sci Transl Med 2015; 7(292): 292ra100, http://dx.doi.org/10.1126/scitranslmed.3010611.
- Kantelhardt S.R., Finke M., Schweikard A., Giese A. Evaluation of a completely robotized neurosurgical operating microscope. Neurosurgery 2013; 72(Suppl 1): A19–A26, http://dx.doi.org/10.1227/NEU.0b013e31827235f8.
- Finke M., Kantelhardt S., Schlaefer A., Bruder R., Lankenau E., Giese A., Schweikard A. Automatic scanning of large tissue areas in neurosurgery using optical coherence tomography. Int J Med Robot 2012; 8(3): 327–336, http://dx.doi.org/10.1002/rcs.1425.
- Lankenau E., Klinger D., Winter C., Malik A., Müller H., Oelckers S., Pau H.-W., Just T., Hüttmann G. Combining optical coherence tomography (OCT) with an operating microscope. In: Advances in medical engineering. Springer Berlin Heidelberg; 2007, p. 343–348, http://dx.doi.org/10.1007/978-3-540-68764-1_57.
- Schmitt J.M., Xiang S.H. Cross-polarized backscatter in optical coherence tomography of biological tissue. Opt Lett 1998; 23(13): 1060–1062, http://dx.doi.org/10.1364/ol.23.001060.
- Gladkova N., Kiseleva E., Streltsova O., Prodanets N., Snopova L., Karabut M., Gubarkova E., Zagaynova E. Combined use of fluorescence cystoscopy and cross-polarization OCT for diagnosis of bladder cancer and correlation with immunohistochemical markers. J Biophotonics 2013; 6(9): 687–698, http://dx.doi.org/10.1002/jbio.201200105.
- Gladkova N., Streltsova O., Zagaynova E., Kiseleva E., Gelikonov V., Gelikonov G., Karabut M., Yunusova K., Evdokimova O. Cross-polarization optical coherence tomography for early bladder-cancer detection: statistical study. J Biophotonics 2011; 4(7–8): 519–532, http://dx.doi.org/10.1002/jbio.201000088.
- Gladkova N., Kiseleva E., Robakidze N., Balalaeva I., Karabut M., Gubarkova E., Feldchtein F. Evaluation of oral mucosa collagen condition with cross-polarization optical coherence tomography. J Biophotonics 2013; 6(4): 321–329, http://dx.doi.org/10.1002/jbio.201200059.
- Kiseleva E., Kirillin M., Feldchtein F., Vitkin A., Sergeeva E., Zagaynova E., Streltzova O., Shakhov B., Gubarkova E., Gladkova N. Differential diagnosis of human bladder mucosa pathologies in vivo with cross-polarization optical coherence tomography. Biomed Opt Express 2015; 6(4):1464–1476, http://dx.doi.org/10.1364/boe.6.001464.
- Leitgeb R.A., Werkmeister R.M., Blatter C., Schmetterer L. Doppler optical coherence tomography. Prog Retin Eye Res 2014; 41: 26–43, http://dx.doi.org/10.1016/j.preteyeres.2014.03.004.
- Chong S.P., Merkle C.W., Leahy C., Srinivasan V.J. Cerebral metabolic rate of oxygen (CMRO2) assessed by combined Doppler and spectroscopic OCT. Biomed Opt Express 2015; 6(10): 3941–3951, http://dx.doi.org/10.1364/BOE.6.003941.
- Devor A., Sakadzic S., Srinivasan V.J., Yaseen M.A., Nizar K., Saisan P.A., Tian P., Dale A.M., Vinogradov S.A., Franceschini M.A., Boas D.A. Frontiers in optical imaging of cerebral blood flow and metabolism. J Cereb Blood Flow Metab 2012; 32(7): 1259–1276, http://dx.doi.org/10.1038/jcbfm.2011.195.
- Srinivasan V.J., Sakadžić S., Gorczynska I., Ruvinskaya S., Wu W., Fujimoto J.G., Boas D.A. Quantitative cerebral blood flow with optical coherence tomography. Opt Express 2010; 18(3): 2477–2494, http://dx.doi.org/10.1364/OE.18.002477.
- Lee J., Wu W., Lesage F., Boas D.A. Multiple-capillary measurement of RBC speed, flux, and density with optical coherence tomography. J Cereb Blood Flow Metab 2013; 33(11): 1707–1710, http://dx.doi.org/10.1038/jcbfm.2013.158.
- Srinivasan V.J., Atochin D.N., Radhakrishnan H., Jiang J.Y., Ruvinskaya S., Wu W., Barry S., Cable A.E., Ayata C., Huang P.L., Boas D.A. Optical coherence tomography for the quantitative study of cerebrovascular physiology. J Cereb Blood Flow Metab 2011; 31(6): 1339–1345, http://dx.doi.org/10.1038/jcbfm.2011.19.
- Ren H., Du C., Yuan Z., Park K., Volkow N.D., Pan Y. Cocaine-induced cortical microischemia in the rodent brain: clinical implications. Mol Psychiatry 2012; 17(10): 1017–1025, http://dx.doi.org/10.1038/mp.2011.160.