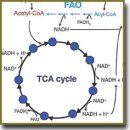
Metabolic Imaging in the Study of Oncological Processes (Review)
There have been reviewed the main approaches to the study of energy metabolism in cancer cells, based on fluorescent imaging of NADH and FAD cofactors. The term “metabolic imaging” covers a range of modern fluorescent techniques for detecting NADH and FAD according to fluorescence intensity and/or lifetime. These cofactors play an important role in energy metabolism reactions acting as electron carriers and, being fluorescent, serve as a basis for metabolic process analysis in living cells and tissues without the use of additional coloring agents. Particular attention is paid to metabolic changes associated with carcinogenesis. Numerous examples of metabolic imaging application in cell cultures in vitro, animal and human tumors in vivo, as well as in patients’ tumor biopsy samples have demonstrated its being highly demanded for biomedical research in the area of oncology.
- Chakraborty S., Nian F.-S., Tsai J.-W., Karmenyan A., Chiou A. Quantification of the metabolic state in cell-model of parkinson’s disease by fluorescence lifetime imaging microscopy. Sci Rep 2016; 6: 19145, https://doi.org/10.1038/srep19145.
- Folmes C.D., Dzeja P.P., Nelson T.J., Terzic A. Metabolic plasticity in stem cell homeostasis and differentiation. Cell Stem Cell 2012; 11(5): 596–606, https://doi.org/10.1016/j.stem.2012.10.002.
- Balu M., Zachary C.B., Harris R.M., Krasieva T.B., König K., Tromberg B.J., Kelly K.M. In vivo multiphoton microscopy of basal cell carcinoma. JAMA Dermatol 2015; 151(10): 1068–1074, https://doi.org/10.1001/jamadermatol.2015.0453.
- Corpas F.J., Barroso J.B. NADPH-generating dehydrogenases: their role in the mechanism of protection against nitro-oxidative stress induced by adverse environmental conditions. Front Environ Sci 2014; 2, https://doi.org/10.3389/fenvs.2014.00055.
- Heikal A.A. Intracellular coenzymes as natural biomarkers for metabolic activities and mitochondrial anomalies. Biomark Med 2010; 4(2): 241–263, https://doi.org/10.2217/bmm.10.1.
- Shi F., Li Y., Li Y., Wang X. Molecular properties, functions, and potential applications of NAD kinases. Acta Biochim Biophys Sin (Shanghai) 2009; 41(5): 352–361, https://doi.org/10.1093/abbs/gmp029.
- Xia W., Wang Z., Wang Q., Han J., Zhao C., Hong Y., Zeng L., Tang L., Ying W. Roles of NAD(+)/NADH and NADP(+)/NADPH in cell death. Curr Pharm Des 2009; 15(1): 12–19, https://doi.org/10.2174/138161209787185832.
- Cairns R.A., Harris I.S., Mak T.W. Regulation of cancer cell metabolism. Nat Rev Cancer 2011; 11(2): 85–95, https://doi.org/10.1038/nrc2981.
- Cantor J.R., Sabatini D.M. Cancer cell metabolism: one hallmark, many faces. Cancer Discov 2012; 2(10): 881–898, https://doi.org/10.1158/2159-8290.cd-12-0345.
- Kunz W.S., Kunz W. Contribution of different enzymes to flavoprotein fluorescence of isolated rat liver mitochondria. Biochim Biophys Acta 1985; 841(3): 237–246, https://doi.org/10.1016/0304-4165(85)90064-9.
- Saks V.A., Veksler V.I., Kuznetsov A.V., Kay L., Sikk P., Tiivel T., Tranqui L., Olivares J., Winkler K., Wiedemann F., Kunz W.S. Permeabilized cell and skinned fiber techniques in studies of mitochondrial function in vivo. In: Bioenergetics of the cell: quantitative aspects. Springer Science + Business Media; 1998; p. 81–100, https://doi.org/10.1007/978-1-4615-5653-4_7.
- Pollak N., Dölle C., Ziegler M. The power to reduce: pyridine nucleotides — small molecules with a multitude of functions. Biochem J 2007; 402(2): 205–218, https://doi.org/10.1042/bj20061638.
- Ying W. NAD+/NADH and NADP+/NADPH in cellular functions and cell death: regulation and biological consequences. Antioxid Redox Signal 2007; 10(2): 179–206, https://doi.org/10.1089/ars.2007.1672.
- Smeitink J., van den Heuvel L., DiMauro S. The genetics and pathology of oxidative phosphorylation. Nat
Rev Gene 2001; 2(5): 342–352, https://doi.org/10.1038/ 35072063. - Papa S., Martino P.L., Capitanio G., Gaballo A., De Rasmo D., Signorile A., Petruzzella V. The oxidative phosphorylation system in mammalian mitochondria. Adv Exp Med Biol 2012; 942: 3–37, https://doi.org/10.1007/978-94-007-2869-1_1.
- Gautheron D.C. Mitochondrial oxidative phosphorylation and respiratory chain: review. J Inherit Metab Dis 1984; 7(Suppl 1): 57–61, https://doi.org/10.1007/bf03047376.
- Nsiah-Sefaa A., McKenzie M. Combined defects in oxidative phosphorylation and fatty acid β-oxidation in mitochondrial disease. Biosci Rep 2016; 36(2): e00313, https://doi.org/10.1042/BSR20150295.
- Chorvat D., Chorvatova A. Multi-wavelength fluorescence lifetime spectroscopy: a new approach to the study of endogenous fluorescence in living cells and tissues. Laser Phys Lett 2009; 6(3): 175–193, https://doi.org/10.1002/lapl.200810132.
- Patterson G.H., Knobel S.M., Arkhammar P., Thastrup O., Piston D.W. Separation of the glucose-stimulated cytoplasmic and mitochondrial NAD(P)H responses in pancreatic islet beta cells. Proc Natl Acad Sci USA 2000; 97(10): 5203–5207, https://doi.org/10.1073/pnas.090098797.
- Richards-Kortum R., Sevick-Muraca E. Quantitative optical spectroscopy for tissue diagnosis. Annu Rev Phys Chem 1996; 47: 555–606, https://doi.org/10.1146/annurev.physchem.47.1.555.
- Blacker T.S., Mann Z.F., Gale J.E., Ziegler M., Bain A.J., Szabadkai G., Duchen M.R. Separating NADH and NADPH fluorescence in live cells and tissues using FLIM. Nat Commun 2014; 5: 3936, https://doi.org/10.1038/ncomms4936.
- Meleshina A.V., Dudenkova V.V., Shirmanova M.V., Shcheslavskiy V.I., Becker W., Bystrova A.S., Cherkasova E.I., Zagaynova E.V. Probing metabolic states of differentiating stem cells using two-photon FLIM. Sci Rep 2016; 6: 21853, https://doi.org/10.1038/srep21853.
- Klaidman L.K., Leung A.C., Adams J.D. Jr. High-performance liquid chromatography analysis of oxidized and reduced pyridine dinucleotides in specific brain regions. Anal Biochem 1995; 228(2): 312–317, https://doi.org/10.1006/abio.1995.1356.
- Huang S., Heikal A.A., Webb W.W. Two-photon fluorescence spectroscopy and microscopy of NAD(P)H and flavoprotein. Biophys J 2002; 82(5): 2811–2825, https://doi.org/10.1016/s0006-3495(02)75621-x.
- Rocheleau J.V., Head W.S., Piston D.W. Quantitative NAD(P)H/flavoprotein autofluorescence imaging reveals metabolic mechanisms of pancreatic islet pyruvate response. J Biol Chem 2004; 279(30): 31780–31787, https://doi.org/10.1074/jbc.m314005200.
- Georgakoudi I., Quinn K.P. Optical imaging using endogenous contrast to assess metabolic state. Annu Rev Biomed Eng 2012; 14: 351–367, https://doi.org/10.1146/annurev-bioeng-071811-150108.
- Kim H.J., Winge D.R. Emerging concepts in the flavinylation of succinate dehydrogenase. Biochim Biophys Acta 2013; 1827(5): 627–636, https://doi.org/10.1016/j.bbabio.2013.01.012.
- Wouters B.G., Koritzinsky M. Hypoxia signalling through mTOR and the unfolded protein response in cancer. Nat Rev Cancer 2008; 8(11): 851–864, https://doi.org/10.1038/nrc2501.
- Marusyk A., Polyak K. Tumor heterogeneity: causes and consequences. Biochim Biophys Acta 2010; 1805(1): 105–117, https://doi.org/10.1016/j.bbcan.2009.11.002.
- DeBerardinis R.J., Lum J.J., Hatzivassiliou G., Thompson C.B. The biology of cancer: metabolic reprogramming fuels cell growth and proliferation. Cell Metab 2008; 7(1): 11–20, https://doi.org/10.1016/j.cmet.2007.10.002.
- Edinger A.L., Linardic C.M., Chiang G.G., Thompson C.B., Abraham R.T. Differential effects of rapamycin on mammalian target of rapamycin signaling functions in mammalian cells. Cancer Res 2003; 63(23): 8451–8460.
- Peng T., Golub T.R., Sabatini D.M. The immunosuppressant rapamycin mimics a starvation-like signal distinct from amino acid and glucose deprivation. Mol Cell Biol 2002; 22(15): 5575–5584, https://doi.org/10.1128/mcb.22.15.5575-5584.2002.
- Masson N., Ratcliffe P.J. Hypoxia signaling pathways in cancer metabolism: the importance of co-selecting interconnected physiological pathways. Cancer Metab 2014; 2(1): 3, https://doi.org/10.1186/2049-3002-2-3.
- Solaini G., Baracca A., Lenaz G., Sgarbi G. Hypoxia and mitochondrial oxidative metabolism. Biochim Biophys Acta 2010; 1797(6–7): 1171–1177, https://doi.org/10.1016/j.bbabio.2010.02.011.
- Zhang H., Bosch-Marce M., Shimoda L.A., Tan Y.S., Baek J.H., Wesley J.B., Gonzalez F.J., Semenza G.L. Mitochondrial autophagy is an HIF-1-dependent adaptive metabolic response to hypoxia. J Biol Chem 2008; 283(16): 10892–10903, https://doi.org/10.1074/jbc.M800102200.
- Zhang H., Gao P., Fukuda R., Kumar G., Krishnamachary B., Zeller K.I., Dang C.V., Semenza G.L. HIF-1 inhibits mitochondrial biogenesis and cellular respiration in VHL-deficient renal cell carcinoma by repression of C-MYC activity. Cancer Cell 2007; 11(5): 407–420, https://doi.org/10.1016/j.ccr.2007.04.001.
- Lopez-Lazaro M. The warburg effect: why and how do cancer cells activate glycolysis in the presence of oxygen? Anticancer Agents Med Chem 2008; 8(3): 305–312, https://doi.org/10.2174/187152008783961932.
- Warburg O., Wind F., Negelein E. The metabolism of tumors in the body. J Gen Physiol 1927; 8(6): 519–530, https://doi.org/10.1085/jgp.8.6.519.
- Pelicano H., Martin D.S., Xu R.H., Huang P. Glycolysis inhibition for anticancer treatment. Oncogene 2006; 25(34): 4633–4646, https://doi.org/10.1038/sj.onc.1209597.
- Zheng J. Energy metabolism of cancer: glycolysis versus oxidative phosphorylation (review). Oncol Lett 2012; 4(6): 1151–1157, https://doi.org/10.3892/ol.2012.928.
- Berridge M.V., Herst P.M., Tan A.S. Metabolic flexibility and cell hierarchy in metastatic cancer. Mitochondrion 2010; 10(6): 584–588, https://doi.org/10.1016/j.mito.2010.08.002.
- Diaz-Ruiz R., Rigoulet M., Devin A. The Warburg and Crabtree effects: on the origin of cancer cell energy metabolism and of yeast glucose repression. Biochim Biophys Acta 2011; 1807(6): 568–576, https://doi.org/10.1016/j.bbabio.2010.08.010.
- Fantin V.R., St-Pierre J., Leder P. Attenuation of LDH-A expression uncovers a link between glycolysis, mitochondrial physiology, and tumor maintenance. Cancer Cell 2006; 9(6): 425–434, https://doi.org/10.1016/j.ccr.2006.04.023.
- Moreno-Sánchez R., Rodríguez-Enríquez S., Marín-Hernández A., Saavedra E. Energy metabolism in tumor cells. FEBS J 2007; 274(6): 1393–1418, https://doi.org/10.1111/j.1742-4658.2007.05686.x.
- Brand K.A., Hermfisse U. Aerobic glycolysis by proliferating cells: a protective strategy against reactive oxygen species. Faseb J 1997; 11(5): 388–395.
- Lunt S.Y., Vander Heiden M.G. Aerobic glycolysis: meeting the metabolic requirements of cell proliferation. Annu Rev Cell Dev Biol 2011; 27: 441–464, https://doi.org/10.1146/annurev-cellbio-092910-154237.
- Fogal V., Richardson A.D., Karmali P.P., Scheffler I.E., Smith J.W., Ruoslahti E. Mitochondrial p32 protein is a critical regulator of tumor metabolism via maintenance of oxidative phosphorylation. Mol Cell Biol 2010; 30(6): 1303–1318, https://doi.org/10.1128/MCB.01101-09.
- Zu X.L., Guppy M. Cancer metabolism: facts, fantasy, and fiction. Biochem Biophys Res Commu 2004; 313(3): 459–465, https://doi.org/10.1016/j.bbrc.2003.11.136.
- Robertson-Tessi M., Gillies R.J., Gatenby R.A., Anderson A.R. Impact of metabolic heterogeneity on tumor growth, invasion, and treatment outcomes. Cancer Res 2015; 75(8): 1567–1579, https://doi.org/10.1158/0008-5472.CAN-14-1428.
- Sengupta D., Pratx G. Imaging metabolic heterogeneity in cancer. Mol Cancer 2016; 15: 4, https://doi.org/10.1186/s12943-015-0481-3.
- Xu H.N., Feng M., Moon L., Dolloff N., El-Deiry W., Li L.Z. Redox imaging of the p53-dependent mitochondrial redox state in colon cancer ex vivo. J Innov Opt Health Sci 2013; 6(3): 1350016, https://doi.org/10.1142/s1793545813500168.
- Chance B., Schoener B., Oshino R., Itshak F., Nakase Y. Oxidation-reduction ratio studies of mitochondria in freeze-trapped samples. NADH and flavoprotein fluorescence signals. J Biol Chem 1979; 254(11): 4764–4771.
- Levitt J.M., Baldwin A., Papadakis A., Puri S., Xylas J., Munger K., Georgakoudi I. Intrinsic fluorescence and redox changes associated with apoptosis of primary human epithelial cells. J Biomed Opt 2006; 11(6): 064012, https://doi.org/10.1117/1.2401149.
- Natal R. de A., Pelegati V.B., Bondarik C., Mendonça G.R., Derchain S.F., Lima C.P., Cesar C.L., Sarian L.O., Vassalloet J. Increased metabolic activity detected by FLIM in human breast cancer cells with desmoplastic reaction: a pilot study. Proc. SPIE 9536, Advanced Microscopy Techniques IV; and Neurophotonics II, 95360L (July 14, 2015), https://doi.org/10.1117/12.2183442.
- Quinn K.P., Sridharan G.V., Hayden R.S., Kaplan D.L., Lee K., Georgakoudi I. Quantitative metabolic imaging using endogenous fluorescence to detect stem cell differentiation. Sci Rep 2013; 3: 3432, https://doi.org/10.1038/srep03432.
- Zhang Z., Blessington D., Li H., Busch T.M., Glickson J., Luo Q., Chance B., Zheng G. Redox ratio of mitochondria as an indicator for the response of photodynamic therapy. J Biomed Opt 2004; 9(4): 772–778, https://doi.org/10.1117/1.1760759.
- Druzhkova I.N., Shirmanova M.V., Lukina M.M., Dudenkova V.V., Mishina N.M., Zagaynova E.V. The metabolic interaction of cancer cells and fibroblasts — coupling between NAD(P)H and FAD, intracellular pH and hydrogen peroxide. Cell Cycle 2016; 15(9): 1257–1266, https://doi.org/10.1080/15384101.2016.1160974.
- Skala M., Ramanujam N. Multiphoton redox ratio imaging for metabolic monitoring in vivo. Methods Mol Biol 2010; 594: 155–162, https://doi.org/10.1007/978-1-60761-411-1_11.
- Skala M.C., Fontanella A., Lan L., Izatt J.A., Dewhirst M.W. Longitudinal optical imaging of tumor metabolism and hemodynamics. J Biomed Opt 2010; 15(1): 011112, https://doi.org/10.1117/1.3285584.
- Skala M.C., Riching K.M., Gendron-Fitzpatrick A., Eickhoff J., Eliceiri K.W., White J.G., Ramanujam N. In vivo multiphoton microscopy of NADH and FAD redox states, fluorescence lifetimes, and cellular morphology in precancerous epithelia. Proc Natl Acad Sci USA 2007; 104(49): 19494–19499, https://doi.org/10.1073/pnas.0708425104.
- Cannon T.M., Shah A.T., Walsh A.J., Skala M.C. High-throughput measurements of the optical redox ratio using a commercial microplate reader. J Biomed Opt 2015; 20(1): 010503, https://doi.org/10.1117/1.jbo.20.1.010503.
- Ostrander J.H., McMahon C.M., Lem S., Millon S.R., Brown J.Q., Seewaldt V.L., Ramanujam N. Optical redox ratio differentiates breast cancer cell lines based on estrogen receptor status. Cancer Res 2010; 70(11): 4759–4766, https://doi.org/10.1158/0008-5472.can-09-2572.
- Staniszewski K., Audi S.H., Sepehr R., Jacobs E.R., Ranji M. Surface fluorescence studies of tissue mitochondrial redox state in isolated perfused rat lungs. Ann Biomed Eng 2013; 41(4): 827–836, https://doi.org/10.1007/s10439-012-0716-z.
- Sepehr R., Staniszewski K., Maleki S., Jacobs E.R., Audi S., Ranji M. Optical imaging of tissue mitochondrial redox state in intact rat lungs in two models of pulmonary oxidative stress. J Biomed Opt 2012; 17(4): 046010, https://doi.org/10.1117/1.JBO.17.4.046010.
- Currie E., Schulze A., Zechner R., Walther T.C., Farese R.V. Jr. Cellular fatty acid metabolism and cancer. Cell Metab 2013; 18(2): 153–161, https://doi.org/10.1016/j.cmet.2013.05.017.
- Fox C.J., Hammerman P.S., Thompson C.B. Fuel feeds function: energy metabolism and the T-cell response. Nat Rev Immunol 2005; 5(11): 844–852, https://doi.org/10.1038/nri1710.
- Chance B. Optical method. Annu Rev Biophys Biophys Chem 1991; 20(1): 1–30, https://doi.org/10.1146/annurev.bb.20.060191.000245.
- Palmer S., Litvinova K., Rafailov E.U., Nabi G. Detection of urinary bladder cancer cells using redox ratio and double excitation wavelengths autofluorescence. Biomed Opt Express 2015; 6(3): 977–986, https://doi.org/10.1364/BOE.6.000977.
- Cannon T.M., Shah A.T., Skala M.C. Validation and characterization of optical redox ratio measurements with a microplate reader in breast cancer cells. Proc. SPIE 9303, Photonic Therapeutics and Diagnostics XI, 93032S (February 26, 2015), https://doi.org/10.1117/12.2077941.
- Hou J., Wright H.J., Chan N., Tran R., Razorenova O.V., Potma E.O., Tromberg B.J. Correlating two-photon excited fluorescence imaging of breast cancer cellular redox state with seahorse flux analysis of normalized cellular oxygen consumption. J Biomed Opt 2016; 21(6): 60503, https://doi.org/10.1117/1.JBO.21.6.060503.
- Walsh A., Cook R.S., Rexer B., Arteaga C.L., Skala M.C. Optical imaging of metabolism in HER2 overexpressing breast cancer cells. Biomed Opt Express 2012; 3(1): 75–85, https://doi.org/10.1364/boe.3.000075.
- Walsh A.J., Cook R.S., Manning H.C., Hicks D.J., Lafontant A., Arteaga C.L., Skala M.C. Optical metabolic imaging identifies glycolytic levels, sub-types and early treatment response in breast cancer. Cancer Res 2013; 73(20): 6164–6174, https://doi.org/10.1158/0008-5472.can-13-0527.
- Levitt J.M., McLaughlin-Drubin M.E., Münger K., Georgakoudi I. Automated biochemical, morphological, and organizational assessment of precancerous changes from endogenous two-photon fluorescence images. PLoS ONE 2011; 6(9): e24765, https://doi.org/10.1371/journal.pone.0024765.
- Shah A.T., Demory Beckler M., Walsh A.J., Jones W.P., Pohlmann P.R., Skala M.C. Optical metabolic imaging of treatment response in human head and neck squamous cell carcinoma. PLoS ONE 2014; 9(3): e90746, https://doi.org/10.1371/journal.pone.0090746.
- Kirkpatrick N.D., Zou C., Brewer M.A., Brands W.R., Drezek R.A., Utzinger U. Endogenous fluorescence spectroscopy of cell suspensions for chemopreventive drug monitoring. Photochem Photobiol 2005; 81(1): 125–134, https://doi.org/10.1562/2004-08-09-ra-267.1.
- Gurushankar K., Nazeer S.S., Jayasree R.S., Krishnakumar N. Evaluation of antitumor activity of hesperetin-loaded nanoparticles against dmba-induced oral carcinogenesis based on tissue autofluorescence spectroscopy and multivariate analysis. J Fluoresc 2015; 25(4): 931–939, https://doi.org/10.1007/s10895-015-1575-4.
- Gohulkumar M., Nazeer S.S., Jayasree R.S., Gurushankar K., Krishnakumar N. Monitoring the metabolic response to nanoencapsulated silibinin treatment in DMBA-induced oral carcinogenesis using endogenous fluorescence. Anal Methods 2014; 6(24): 9744–9753, https://doi.org/10.1039/c4ay01877j.
- Xu H.N., Nioka S., Glickson J.D., Chance B., Li L.Z. Quantitative mitochondrial redox imaging of breast cancer metastatic potential. J Biomed Opt 2010; 15(3): 036010, https://doi.org/10.1117/1.3431714.
- Shah A.T., Diggins K.E., Walsh A.J., Irish J.M., Skala M.C. In vivo autofluorescence imaging of tumor heterogeneity in response to treatment. Neoplasia 2015; 17(12): 862–870, https://doi.org/10.1016/j.neo.2015.11.006.
- Zhang M., Zhang Z., Blessington D., Li H., Busch T.M., Madrak V., Miles J., Chance B., Glickson J.D., Zheng G. Pyropheophorbide 2-deoxyglucosamide: a new photosensitizer targeting glucose transporters. Bioconjug Chem 2003; 14(4): 709–714, https://doi.org/10.1021/bc034038n.
- Shah A.T., Skala M.C. Ex vivo label-free microscopy of head and neck cancer patient tissues. Proc. SPIE 9329, Multiphoton Microscopy in the Biomedical Sciences XV, 93292B (March 5, 2015), https://doi.org/10.1117/12.2075583.
- Ramanujam N., Richards-Kortum R., Thomsen S., Mahadevan-Jansen A., Follen M., Chance B. Low temperature fluorescence imaging of freeze-trapped human cervical tissues. Opt Express 2001; 8(6): 335–343, https://doi.org/10.1364/oe.8.000335.
- Liu Q., Grant G., Li J., Zhang Y., Hu F., Li S., Wilson C., Chen K., Bigner D., Vo-Dinh T. Compact point-detection fluorescence spectroscopy system for quantifying intrinsic fluorescence redox ratio in brain cancer diagnostics. J Biomed Opt 2011; 16(3): 037004, https://doi.org/10.1117/1.3558840.
- Georgakoudi I., Jacobson B.C., Müller M.G., Sheets E.E., Badizadegan K., Carr-Locke D.L., Crum C.P., Boone C.W., Dasari R.R., Van Dam J., Feld M.S. NAD(P)H and collagen as in vivo quantitative fluorescent biomarkers of epithelial precancerous changes. Cancer Res 2002; 62(3): 682–687.
- Müller M.G., Valdez T.A., Georgakoudi I., Backman V., Fuentes C., Kabani S., Laver N., Wang Z., Boone C.W., Dasari R.R., Shapshay S.M., Feld M.S. Spectroscopic detection and evaluation of morphologic and biochemical changes in early human oral carcinoma. Cancer 2003; 97(7): 1681–1692, https://doi.org/10.1002/cncr.11255.
- Yu Q., Heikal A.A. Two-photon autofluorescence dynamics imaging reveals sensitivity of intracellular NADH concentration and conformation to cell physiology at the single-cell level. J Photochem Photobiol B 2009; 95(1): 46–57, https://doi.org/10.1016/j.jphotobiol.2008.12.010.
- Tregidgo C., Levitt J.A., Suhling K. Effect of refractive index on the fluorescence lifetime of green fluorescent protein. J Biomed Opt 2008; 13(3): 031218, https://doi.org/10.1117/1.2937212.
- van Manen H.J., Verkuijlen P., Wittendorp P., Subramaniam V., van den Berg T.K., Roos D., Otto C. Refractive index sensing of green fluorescent proteins in living cells using fluorescence lifetime imaging microscopy. Biophys J 2008; 94(8): L67–L69, https://doi.org/10.1529/biophysj.107.127837.
- Lakowicz J.R., Szmacinski H., Nowaczyk K., Johnson M.L. Fluorescence lifetime imaging of free and protein-bound NADH. Proc Natl Acad Sci USA 1992; 89(4): 1271–1275, https://doi.org/10.1073/pnas.89.4.1271.
- Duncan R.R. Fluorescence lifetime imaging microscopy (FLIM) to quantify protein–protein interactions inside cells. Biochem Soc Trans 2006; 34(Pt 5): 679–682, https://doi.org/10.1042/bst0340679.
- Elson D., Webb S., Siegel J., Suhling K., Davis D., Lever J., Phillips D., Wallace A., French P. Biomedical applications of fluorescence lifetime imaging. Opt Photon News 2002; 13(11): 26–32, https://doi.org/10.1364/opn.13.11.000026.
- Campos-Delgado D.U., Navarro O.G., Arce-Santana E.R., Walsh A.J., Skala M.C., Jo J.A. Deconvolution of fluorescence lifetime imaging microscopy by a library of exponentials. Opt Express 2015; 23(18): 23748–23767, https://doi.org/10.1364/oe.23.023748.
- Becker W. Fluorescence lifetime imaging — techniques and applications. J Microsc 2012; 247(2): 119–136, https://doi.org/10.1111/j.1365-2818.2012.03618.x.
- Becker W., Bergmann A., Hink M.A., Konig K., Benndorf K., Biskup C. Fluorescence lifetime imaging by time-correlated single-photon counting. Microsc Res Tech 2004; 63(1): 58–66, https://doi.org/10.1002/jemt.10421.
- Skala M.C., Riching K.M., Bird D.K., Gendron-Fitzpatrick A., Eickhoff J., Eliceiri K.W., Keely P.J., Ramanujam N. In vivo multiphoton fluorescence lifetime imaging of protein-bound and free nadh in normal and pre-cancerous epithelia. J Biomed Opt 2007; 12(2): 024014, https://doi.org/10.1117/1.2717503.
- Kantelhardt S.R., Kalasauskas D., Konig K., Kim E., Weinigel M., Uchugonova A., Giese A. In vivo multiphoton tomography and fluorescence lifetime imaging of human brain tumor tissue. J Neurooncol 2016; 127(3): 473–482, https://doi.org/10.1007/s11060-016-2062-8.
- Seidenari S., Arginelli F., Dunsby C., French P.M., Konig K., Magnoni C., Talbot C., Ponti G. Multiphoton laser tomography and fluorescence lifetime imaging of melanoma: morphologic features and quantitative data for sensitive and specific non-invasive diagnostics. PLoS ONE 2013; 8(7): e70682, https://doi.org/10.1371/journal.pone.0070682.
- Ruck A., Hauser C., Mosch S., Kalinina S. Spectrally resolved fluorescence lifetime imaging to investigate cell metabolism in malignant and nonmalignant oral mucosa cells. J Biomed Opt 2014; 19(9): 096005, https://doi.org/10.1117/1.jbo.19.9.096005.
- Ramanujan V.K., Zhang J.H., Biener E., Herman B. Multiphoton fluorescence lifetime contrast in deep tissue imaging: prospects in redox imaging and disease diagnosis. J Biomed Opt 2005; 10(5): 051407, https://doi.org/10.1117/1.2098753.
- Vergen J., Hecht C., Zholudeva L.V., Marquardt M.M., Hallworth R., Nichols M.G. Metabolic imaging using two-photon excited NADH intensity and fluorescence lifetime imaging. Microsc Microanal 2012; 18(4): 761–770, https://doi.org/10.1017/S1431927612000529.
- Bird D.K., Yan L., Vrotsos K.M., Eliceiri K.W., Vaughan E.M., Keely P.J., White J.G., Ramanujam N. Metabolic mapping of MCF10A human breast cells via multiphoton fluorescence lifetime imaging of the coenzyme NADH. Cancer Res 2005; 65(19): 8766–8773, https://doi.org/10.1158/0008-5472.can-04-3922.
- Islam M.S., Honma M., Nakabayashi T., Kinjo M., Ohta N. pH dependence of the fluorescence lifetime of FAD in solution and in cells. Int J Mol Sci 2013; 14(1): 1952–1963, https://doi.org/10.3390/ijms14011952.
- Horilova J., Cunderlikova B., Marcek Chorvatova A. Time- and spectrally resolved characteristics of flavin fluorescence in U87MG cancer cells in culture. J Biomed Opt 2015; 20(5): 051017, https://doi.org/10.1117/1.jbo.20.5.051017.
- Fatakdawala H., Poti S., Zhou F., Sun Y., Bec J., Liu J., Yankelevich D.R., Tinling S.P., Gandour-Edwards R.F., Farwell D.G., Marcu L. Multimodal in vivo imaging of oral cancer using fluorescence lifetime, photoacoustic and ultrasound techniques. Biomed Opt Express 2013; 4(9): 1724–1741, https://doi.org/10.1364/boe.4.001724.
- Adur J., Pelegati V.B., de Thomaz A.A., Baratti M.O., Almeida D.B., Andrade L.A., Bottcher-Luiz F., Carvalho H.F., Cesar C.L. Optical biomarkers of serous and mucinous human ovarian tumor assessed with nonlinear optics microscopies. PLoS ONE 2012; 7(10): e47007, https://doi.org/10.1371/journal.pone.0047007.
- Galletly N.P., McGinty J., Dunsby C., Teixeira F., Requejo-Isidro J., Munro I., Elson D.S., Neil M.A., Chu A.C., French P.M., Stamp G.W. Fluorescence lifetime imaging distinguishes basal cell carcinoma from surrounding uninvolved skin. Br J Dermatol 2008; 159(1): 152–161, https://doi.org/10.1111/j.1365-2133.2008.08577.x.
- McGinty J., Galletly N.P., Dunsby C., Munro I., Elson D.S., Requejo-Isidro J., Cohen P., Ahmad R., Forsyth A., Thillainayagam A.V., Neil M.A.A., French P.M.W., Stamp G.W. Wide-field fluorescence lifetime imaging of cancer. Biomed Opt Express 2010; 1(2): 627–640, https://doi.org/10.1364/boe.1.000627.
- Sun Y., Hatami N., Yee M., Phipps J., Elson D.S., Gorin F., Schrot R.J., Marcu L. Fluorescence lifetime imaging microscopy for brain tumor image-guided surgery. J Biomed Opt 2010; 15(5): 056022, https://doi.org/10.1117/1.3486612.
- Sun Y., Phipps J.E., Meier J., Hatami N., Poirier B., Elson D.S., Farwell D.G., Marcu L. Endoscopic fluorescence lifetime imaging for in vivo intraoperative diagnosis of oral carcinoma. Microscopy and Microanalysis 2013; 19(4): 791–798, https://doi.org/10.1017/s1431927613001530.
- Butte P.V., Mamelak A.N., Nuno M., Bannykh S.I., Black K.L., Marcu L. Fluorescence lifetime spectroscopy for guided therapy of brain tumors. Neuroimage 2011; 54(Suppl 1): S125–S135, https://doi.org/10.1016/j.neuroimage.2010.11.001.