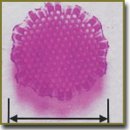
Long-Term Neurological and Behavioral Results of Biodegradable Scaffold Implantation in Mice Brain
The aim of the study was to assess the integral outcomes of implantation biocompatible scaffold as the carrier of neural stem cells in the reconstructive surgery of open traumatic brain injury (TBI) by parameters of
Materials and Methods. Adult male C57BL/6 mice were injured with open-skull weight-drop method. 3D hydrogel scaffold based on modified chitosan in complex with hyaluronic acid was transplanted into the lesion cavity 1 week after TBI. Using a variety of behavioral and cognitive tests (modified neurological severity scores (mNSS), open field test as well as novel object recognition and passive avoidance tests) the short- and long-term neurological and memory functions sequelae induced by TBI were assessed. Magnetic resonance imaging was used to visualize the injury site.
Results. Significant functional recovery was observed on both the mNSS and open field tests in the scaffold transplantation group compared to the control TBI group. In addition, enhanced improvement of short and long-term memory functions was found 5 months post injury. Magnetic resonance imaging data revealed that the scaffold transplantation
Conclusion. Taken together, our findings indicated that transplantation of 3D biodegradable scaffold into injury cavity contributed to the preservation of volume in the damaged region in the first months after TBI which in turn led to a better functional recovery in the remote period.
- Gusev E.I., Konovalov A.N., Skvortsova V.I., Hecht A.B.
Nevrologiya [Nevrologiya]. Moscow:GEOTAR -Media; 2009; 1040 p. - Bullock M.R., Chesnut R., Ghajar J., Gordon D., Hartl R., Newell D.W., Servadei F., Walters B.C., Wilberger J.E. Guidelines for the surgical management of traumatic brain injury. Neurosurgery 2006; 58(3), https://doi.org/10.1097/00006123-200603001-00006.
- Feigin V.L., Barker-
Collo S., Krishnamurthi R., Theadom A., Starkey N. Epidemiology of ischaemic stroke and traumatic brain injury. Best Pract Res Clin Anaesthesiol 2010; 24(4): 485–494, https://doi.org/10.1016/j.bpa.2010.10.006. - Valadka A.B., Robertson C.S. Surgery of cerebral trauma and associated critical care. Neurosurgery 2007; 61(1): 203–221, https://doi.org/10.1227/01.neu.0000255497.26440.01.
- Greenberg M.S. Handbook of neurosurgery. New York: Thieme Publishers; 2010.
- Cheng G., Kong R., Zhang L., Zhang J. Mitochondria in traumatic brain injury and mitochondrial-targeted multipotential therapeutic strategies. Br J Pharmacol 2012; 167(4): 699–719, https://doi.org/10.1111/j.1476-5381.2012.02025.x.
- Liu S.-J., Zou Y., Belegu V., Lv L.-Y., Lin N., Wang T.-Y., McDonald J.-W., Zhou X., Xia Q.-J., Wang T.-H. Co-grafting of neural stem cells with olfactory
en sheathing cells promotes neuronal restoration in traumatic brain injury with an anti-inflammatory mechanism. J Neuroinflammation 2014; 11(1): 66, https://doi.org/10.1186/1742-2094-11-66. Blaya M.O., Tsoulfas P., Bramlett H.M., Dietrich W.D. Neural progenitor cell transplantation promotes neuroprotection, enhances hippocampal neurogenesis, and improves cognitive outcomes after traumatic brain injury. Exp Neurol 2015; 264: 67–81, https://doi.org/10.1016/j.expneurol.2014.11.014.- Dobrowolski S.,
Lepski G. Stem cells in traumatic brain injury. American Journal of Neuroscience 2013; 4(1): 13–24, https://doi.org/10.3844/amjnsp.2013.13.24. - Lima C., Pratas-Vital J., Escada P., Hasse-Ferreira A.,
Capucho C., Peduzzi J.D. Olfactory mucosa autografts in human spinal cord injury: a pilot clinical study. J Spinal Cord Med 2006; 29(3): 191–203, https://doi.org/10.1080/10790268.2006.11753874. - Toft A., Scott D.T., Barnett S.C., Riddell J.S. Electrophysiological evidence that olfactory cell transplants improve function after spinal cord injury. Brain 2006; 130(4): 970–984, https://doi.org/10.1093/brain/awm040.
- Ao Q., Wang A.J., Chen G.Q., Wang S.J., Zuo H.C., Zhang X.F. Combined transplantation of neural stem cells and olfactory ensheathing cells for the repair of spinal cord injuries. Med Hypotheses 2007; 69(6): 1234–1237, https://doi.org/10.1016/j.m ehy.2007.04.011.
- Andrews M.R., Stelzner D.J. Evaluation of olfactory ensheathing and Schwann cells after implantation into a dorsal injury of adult rat spinal cord. J
Neurotraum 2007; 24(11): 1773–1792, https://doi.org/10.1089/neu.2007.0353. Skop N.B., Calderon F., Cho C.H., Gandhi C.D., Levison S.W. Improvements in biomaterial matrices for neural precursor cell transplantation. Mol Cell Ther 2014; 2(1): 19, https://doi.org/10.1186/2052-8426-2-19.- Crompton K.E., Goud J.D., Bellamkonda R.V., Gengenbach T.R., Finkelstein D.I., Horne M.K., Forsythe J.S. Polylysine-functionalised thermoresponsive chitosan hydrogel for neural tissue engineering. Biomaterials 2007; 28(3): 441–449, https://doi.org/10.1016/j.biomaterials.2006.08.044.
- Tate C.C., Shear D.A., Tate M.C., Archer D.R., Stein D.G., LaPlaca M.C. Laminin and fibronectin scaffolds enhance neural stem cell transplantation into the injured brain. J Tissue Eng Regen Med 2009; 3(3): 208–217, https://doi.org/10.1002/term.154.
- Mo L., Yang Z., Zhang A., Li X. The repair of the injured adult
rat hippocampus with NT-3-chitosan carriers. Biomaterials 2010; 31(8): 2184–2192, https://doi.org/10.1016/j.biomaterials.2009.11.078. - Timashev P.S., Demina T.S., Minaev N.V., Bardakova K.N., Koroleva A.V.,
Kufelt O.A., Chichkov B.N., Panchenko V.Ya., Akopova T.A., Bagratashvili V.N. Fabrication of microstructured materials based on chitosan and its derivatives using two-photon polymerization. High Energy Chemistry 2015; 49(4): 300–303, https://doi.org/10.1134/s0018143915040177. Kufelt O., El-Tamer A.,Sehring C., Schlie-Wolter S., Chichkov B.N. Hyaluronic acid based materials for scaffolding via two-photon polymerization. Biomacromolecules 2014; 15(2): 650–659, https://doi.org/10.1021/bm401712q.- Timashev P.S., Bardakova K.N, Minaev N.V., Demina T.S., Mishchenko T.A., Mitroshina E.V., Akovantseva A.A., Koroleva A.V.,
Asyutin D.S., Pimenova L.F., Konovalov N.A., Akopova T.A., Solov’eva A.B., Mukhina I.V., Vedunova M.V., Chichkov B.N., Bagratashvilі V.N. Compatibility of cells of the nervous system with structured biodegradable chitosan-based hydrogel matrices. Applied Biochemistry and Microbiology 2016; 52(5): 508–514, https://doi.org/10.1134/s0003683816050161. Khalin I., Jamari N.L., Razak N.B.,Hasain Z.B., Nor M.A., Zainudin M.H., Omar A.B., Alyautdin R. A mouse model of weight-drop closed head injury: emphasis on cognitive and neurological deficiency. Neural Regen Res 2016; 11(4): 630, https://doi.org/10.4103/1673-5374.180749.- Feeney D.M., Boyeson M.G., Linn R.T., Murray H.M., Dail W.G. Responses to cortical injury: I. Methodology and local effects of contusions in the rat. Brain Res 1981; 211(1): 67–77, https://doi.org/10.1016/0006-8993(81)90067-6.
- Zhang Y.P., Cai J., Shields L.B., Liu N., Xu X.-M., Shields C.B. Traumatic brain injury using mouse models. Transl Stroke Res 2014; 5(4): 454–471, https://doi.org/10.1007/s12975-014-0327-0.
- Flierl M.A., Stahel P.F., Beauchamp K.M., Morgan S.J., Smith W.R., Shohami E. Mouse closed head injury model induced by a weight-drop device. Nat Protoc 2009; 4(9): 1328–1337, https://doi.org/10.1038/nprot.2009.148.
Tsenter J., Beni-Adani L., Assaf Y., Alexandrovich A.G., Trembovler V., Shohami E. Dynamic changes in the recovery after traumatic brain injury in mice: effect of injury severity on T2-weighted MRI abnormalities, and motor and cognitive functions. J Neurotrauma 2008; 25(4): 324–333, https://doi.org/10.1089/neu.2007.0452.- Rolls E.T. The mechanisms for pattern completion and pattern separation in the hippocampus. Front Syst Neurosci 2013; 7, https://doi.org/10.3389/fnsys.2013.00074.
- Huang T.-N., Chuang H.-C., Chou W.-H., Chen C.-Y., Wang H.-F., Chou S.-J., Hsueh Y.P. Tbr1 haploinsufficiency impairs amygdalar axonal projections and results in cognitive abnormality. Nat Neurosci 2014; 17(2): 240–247, https://doi.org/10.1038/nn.3626.
- Moscardo E., Salvetti B.,
Becchi S., Bertini G.,Fabene P.F. The novel object recognition test in rodents: which are the essential methodological aspects? Proceedings of Measuring Behavior 2012; 8: 476–478. - Levy A., Bercovich-Kinori A., Alexandrovich A.G.,
Tsenter J., Trembovler V., Lund F.E., Shohami E., Stein R., Mayo L. cd38 facilitates recovery from traumatic brain injury. J Neurotrauma 2009; 26(9):1521–1533, https://doi.org/10.1089/neu.2008-0746. - Chen S.T., Chen H.H., Chiang Y.C., Yuan Z.F., Kuo C.C., Lai M.D., Hung T.W., Ho I.K., Chen S.T. Buprenorphine, methadone, and morphine treatment during pregnancy: behavioral effects on the offspring
in rats. Neuropsychiatr Dis Treat 2015; 609–618, https://doi.org/10.2147/ndt.s70585. - Buresh J., Bureshova O., Houston D.P. Metodiki i osnovnye eksperimenty po izucheniyu mozga i povedeniya [Methods and basic experiments for the study of brain and behavior]. Moscow: Vysshaya shkola; 1991; 398 p.
- Zohar O., Rubovitch V., Milman A., Schreiber S., Pick C.G. Behavioral consequences of minimal traumatic brain injury in mice. Acta Neurobiol Exp (Wars) 2011; 71(1): 36–45.
- Cao Z. Developing chitosan-based biomaterials for brain repair and neuroprosthetics. Master of Science Degree Thesis. University of Tennessee; 2010. URL:http://trace.tennessee.edu/utk_gradthes/609.
- Engler A.J., Sen S., Sweeney H.L., Discher D.E. Matrix elasticity directs stem cell lineage specification. Cell 2006; 126(4): 677–689, https://doi.org/10.1016/j.cell.2006.06.044.
- Kaster T., Sack I., Samani A. Measurement of the hyperelastic properties of ex vivo brain tissue slices. J Biomech 2011; 44(6): 1158–1163, https://doi.org/10.1016/j.jbiomech.2011.01.019.
- Liao H., Munoz-Pinto D., Qu X., Hou Y., Grunlan M.A., Hahn M.S. Influence of hydrogel mechanical properties and mesh size on vocal fold fibroblast extracellular matrix production and phenotype. Acta Biomaterialia 2008; 4(5): 1161–1171, https://doi.org/10.1016/j.actbio.2008.04.013.
Khaing Z.Z., Thomas R.C., Geissler S.A., Schmidt C.E. Advanced biomaterials for repairing the nervous system: what can hydrogels do for the brain? Materials Today 2014; 17(7): 332–340, https://doi.org/10.1016/j.mattod.2014.05.011.- Carballo-Molina O.A., Velasco I. Hydrogels as scaffolds and delivery systems to enhance axonal regeneration after injuries. Front Cell Neurosci 2015; 9: 13, https://doi.org/10.3389/fncel.2015.00013.
- Shi W., Nie D., Jin G., Chen W., Xia L., Wu X., Su X., Xu X., Ni L., Zhang X., Zhang X., Chen J. BDNF blended chitosan scaffolds for human umbilical
cord MSC transplants in traumatic brain injury therapy. Biomaterials Biomaterials 2012; 33(11): 3119–3126, https://doi.org/10.1016/j.biomaterials.2012.01.009. - Miranda D.G., Malmonge S.M., Campos D.M.,
Attik N.G., Grosgogeat B., Gritsch K. A chitosan-hyaluronic acid hydrogel scaffold for periodontal tissue engineering. J Biomed Mater Res B Appl Biomater 2016; 104(8): 1691–1702, https://doi.org/10.1002/jbm.b.33516. - Timashev P.S., Vedunova M.V.,
Guseva D., Ponimaskin E., Deiwick A., Mishchenko T.A., Mitroshina E.V., Koroleva A.V., Pimashkin A.S., Panchenko V.Ya., Bagratashvili V.N., Mukhina I.V., Chichkov B.N. 3D in vitro platform produced by two-photon polymerization for the analysis of neural network formation and function. Biomed Phys Eng Express 2016; 2(3): 035001, https://doi.org/10.1088/2057-1976/2/3/035001. - Pettikiriarachchi J.T.S., Parish C.L., Shoichet M.S., Forsythe J.S., Nisbet D.R. Biomaterials for brain tissue engineering. Australian Journal of Chemistry 2010; 63(8):1143–1154, https://doi.org/10.1071/ch10159.