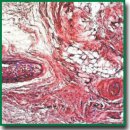
Subcutaneous Implantation of Poly(3-hydroxybutyrate-co-3-hydroxyvalerate) and Poly(ε-caprolactone) Scaffolds Modified with Growth Factors
The aim of the investigation was to assess the tissue response to subcutaneous implantation of nonwoven scaffolds fabricated from biodegradable polymers and further modified by growth factors.
Materials and Methods. Specimens were produced by two-phase electrospinning from poly(ε-caprolactone) and a blend of poly(3-hydroxybutyrate-co-3-hydroxyvalerate) and poly(ε-caprolactone) both unmodified (controls) and modified with growth factors VEGF, bFGF, and SDF-1α. The ratio of a polymer solution in chloroform and a water phase with a bioactive molecule was 20:1. To study the tissue response in vivo, nonwoven scaffolds were subcutaneously implanted in Wistar rats weighing 80–100 g for the periods of either 1, 2, 3, 6, 9, or 12 months.
Results. Incorporating growth factors into the scaffolds during electrospinning provided biofunctionalization, including enhanced vasculogenesis and angiogenesis and increased viability of endothelial cells. Histological examination showed that biomolecules incorporated into the matrix have been functionally active throughout the whole time of the implantation.
The tested specimens did not cause rejection and acute inflammatory reaction. A thin connective tissue capsule was formed around the implants. A full resorption of the scaffolds did not occur, and the polymers remained at the site of implantation for at least 12 months. Growth factors significantly improved performance of the implants during the first 3 months postimplantation: VEGF enhanced angiogenesis, bFGF stimulated a thick connective tissue capsule formation, while SDF-1α facilitated angiogenesis and cellular infiltration. From the 12th month postimplantation, incomplete biodegradation of nonwoven scaffolds caused granulomatous inflammation.
Conclusion. Nonwoven scaffolds fabricated of biodegradable polymers and further modified with VEGF, bFGF, and SDF-1α represent a promising option for the fabrication of cardiovascular implants.
- Catto V., Farè S., Freddi G., Tanzi M.C. Vascular tissue engineering: recent advances in small diameter blood vessel regeneration. ISRN Vascular Medicine 2014; 2014: 923030, https://doi.org/10.1155/2014/923030.
- Hasan A., Memic A., Annabi N., Hossain M., Paul A., Dokmeci M.R., Dehghani F., Khademhosseini A. Electrospun scaffolds for tissue engineering of vascular grafts. Acta Biomater 2014; 10(1): 11–25, https://doi.org/10.1016/j.actbio.2013.08.022.
- Lee Y.-S., Livingston Arinzeh T. Electrospun nanofibrous materials for neural tissue engineering. Polymers 2011; 3(4): 413–426, https://doi.org/10.3390/polym3010413.
- Sankaran K.K., Subramanian A., Krishnan U.M., Sethuraman S. Nanoarchitecture of scaffolds and endothelial cells in engineering small diameter vascular grafts. Biotechnol J 2015; 10(1): 96–108, https://doi.org/10.1002/biot.201400415.
- Antonova L.V., Burago A.Y., Matveeva V.G., Kudryavtseva Y.A., Nasonova M.V., Toropova Y.G., Velikanova E.A., Golovkin A.S. Bioresorption characteristics of plain and cell-loaded polyhydroxyalkanoate and polycaprolactone film scaffolds in chronic long-term experiment. Fundamentalnie issledovania 2013; 7(1): 17–23.
- Antonova L.V., Nasonova M.V., Kudryavtseva Yu.A., Golovkin A.S. Potential for polyhydroxyalkanoates and policaprolactone copolymer use as tissue-engineered scaffolds in cardiovascular surgery. Byulleten sibirskoy meditsiny 2012; 11(1): 128–134.
- Popova I.V., Stepanova A.O., Plotnikova T.A., Sergeevichev D.S., Akulov A.E., Pokushalov A.A., Laktionov P.P., Karpenko A.A. Study of patency of vascular grafts manufactured by means of electrospinning. Angiologiya i sosudistaya khirurgiya 2015; 21(2): 136–141.
- de Valence S., Tille J.-C., Mugnai D., Mrowczynski W., Gurny R., Möller M., Walpoth B.H. Long term performance of polycaprolactone vascular grafts in a rat abdominal aorta replacement model. Biomaterials 2012; 33(1): 38–47, https://doi.org/10.1016/j.biomaterials.2011.09.024.
- Rabkin E., Schoen F.J. Cardiovascular tissue engineering. Cardiovasc Pathol 2002; 11(6): 305–317, https://doi.org/10.1016/s1054-8807(02)00130-8.
- Finlay H.M., Whittaker P., Canham P.B. Collagen organization in the branching region of human brain arteries. Stroke 1998; 29(8): 1595–1601, https://doi.org/10.1161/01.str.29.8.1595.
- Natasha G., Tan A., Gundogan B., Farhatnia Y., Nayyer L., Mahdibeiraghdar S., Rajadas J., De Coppi P., Davies A.H., Seifalian A.M. Tissue engineering vascular grafts a fortiori: looking back and going forward. Expert Opin Biol Ther 2014; 15(2): 231–244, https://doi.org/10.1517/14712598.2015.980234.
- Azimi-Nezhad M. Vascular endothelial growth factor from embryonic status to cardiovascular pathology. Rep Biochem Mol Biol 2014; 2(2): 59–69.
- Harburger D.S., Calderwood D.A. Integrin signalling at a glance. J Cell Sci 2009; 122(9): 1472–1472, https://doi.org/10.1242/jcs.052910.
- GOST ISO 10993-1-2011. Medical devices. Biological evaluation of medical devices. Part 1. Evaluation and testing. 5.2.9 Implantation.
- Nasonova M.V., Antonova I.V., Matveeva V.G., Doronina N.V., Ezhov V.A., Burago A.Y., Glushkova T.V., Kudryavtseva Yu.A. Resorption rate of polyhydroxyalkanoatebased scaffolds and scaffolds with multipotent mesenchymal stromal cells. Complex Issues of Cardiovascular Diseases 2015; 1: 39, https://doi.org/10.17802/2306-1278-2015-1-39-45.