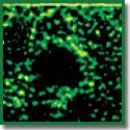
Endocannabinoid System Participates in Neuron-Glial Networks Adaptation to Modeled Ischemia Factors in vitro
The aim of the study was to evaluate the role of a cannabinoid receptor agonist and blockers of key enzymes of endocannabinoid biodegradation in the adaptation of neuron-glial networks to ischemia factors influence.
Materials and Methods. Dissociated hippocampal cells obtained from C57BL/6 murine embryos on the 18th day of gestation were cultivated as primary cultures. Two major factors of ischemia — hypoxia and glucose deprivation — were modeled on day 14 of culture development in vitro. Synthetic endocannabinoid N-arachidonoyl dopamine (NADA) at concentration of 10 μM, irreversible inhibitor of monoacylglycerol lipase JZL184 (1 μM) and inhibitor of two key enzymes of endocannabinoid biodegradation JZL195 (1 μM) were added into the culture medium at the beginning of ischemia factors modeling or immediately after it. The cell viability was assessed on days 1, 3, and 7 after the influence of ischemia factors. On day 7, the spontaneous calcium activity in primary hippocampal cells was analyzed using the multicellular functional calcium imaging. To reveal the molecular neuroprotective mechanisms of the tested compounds, expression of mRNA CB1R receptor and mRNA hypoxia-inducible factor HIF-1α were measured using fluorescent mRNA detection probes (Smart Flare Merck, Germany).
Results. Application of NADA (the agonist of the cannabinoid type 1 and 2 receptors) or JZL195 (the MAGL/FAAH enzymes blocker) into the culture medium has been found to have a neuroprotective effect manifesting itself in a consistent cell death decrease compared with the control cultures. In addition, activation of the endocannabinoid system partially neutralizes the changes induced by hypoxia and glucose deprivation in vitro: the spontaneous calcium activity was detected in a greater number of cells (vs the control group), and the duration and frequency of calcium oscillations were partially normalized.
It was also found that NADA application during hypoxia significantly increased the type 1 cannabinoid receptors expression. When NADA and JZL195 were added the expression level of mRNA HIF-1α factor did not differ from that of the intact cultures.
Conclusion. The synthetic endocannabinoid NADA and the enzyme blocker JZL195 have a pronounced neuroprotective effect on the modeled ischemia factors consisting in the increase of neuronal cell viability and a good functional calcium activity of primary hippocampal cultures in a long-term period after injury.
- Numakawa T. Possible protective action of neurotrophic factors and natural compounds against common neurodegenerative diseases. Neural Regen Res 2014; 9(16): 1506–1508, https://doi.org/10.4103/1673-5374.139474.
- Duarte E.P., Curcio M., Canzoniero L.M., Duarte C.B. Neuroprotection by GDNF in the ischemic brain. Growth Factors 2012; 30(4): 242–257, https://doi.org/10.3109/08977194.2012.691478.
- Picone R.P., Kendall D.A. Minireview: from the bench, toward the clinic: therapeutic opportunities for cannabinoid receptor modulation. Mol Endocrinol 2015; 29(6): 801–813, https://doi.org/10.1210/me.2015-1062.
- Schurman L.D., Lichtman A.H. Endocannabinoids: a promising impact for traumatic brain injury. Front Pharmacol 2017; 8: 69, https://doi.org/10.3389/fphar.2017.00069.
- Pertwee R.G. Cannabinoid pharmacology: the first 66 years. Br J Pharmacol 2006; 147(S1): S163–S171, https://doi.org/10.1038/sj.bjp.0706406.
- Fernández-Ruiz J., Moro M.A., Martínez-Orgado J. Cannabinoids in neurodegenerative disorders and stroke/brain trauma: from preclinical models to clinical applications. Neurotherapeutics 2015; 12(4): 793–806, https://doi.org/10.1007/s13311-015-0381-7.
- Pertwee R.G., Howlett A.C., Abood M.E., Alexander S.P., Di Marzo V., Elphick M.R., Greasley P.J., Hansen H.S., Kunos G., Mackie K., Mechoulam R., Ross R.A. International Union of Basic and Clinical Pharmacology. LXXIX. Cannabinoid receptors and their ligands: beyond CB1 and CB2. Pharmacol Rev 2010; 62(4): 588–631, https://doi.org/10.1124/pr.110.003004.
- Nagayama T., Sinor A.D., Simon R.P., Chen J., Graham S.H., Jin K., Greenberg D.A. Cannabinoids and neuroprotection in global and focal cerebral ischemia and in neuronal cultures. J Neurosci 1999; 19(8): 2987–2995.
- Panikashvili D., Shein N.A., Mechoulam R., Trembovler V., Kohen R., Alexandrovich A., Shohami E. The endocannabinoid 2-AG protects the
blood–brain barrier after closed head injury and inhibits mRNA expression of proinflammatory cytokines. Neurobiol Dis 2006; 22(2): 257–264, https://doi.org/10.1016/j.nbd.2005.11.004. - Shohami E., Cohen-Yeshurun A., Magid L., Algali M., Mechoulam R. Endocannabinoids and traumatic brain injury. Br J Pharmacol 2011; 163(7): 1402–1410, https://doi.org/10.1111/j.1476-5381.2011.01343.x.
- Koch M., Kreutz S., Böttger C., Benz A., Maronde E., Ghadban C., Korf H.W., Dehghani F. Palmitoylethanolamide protects dentate gyrus granule cells via peroxisome proliferator-activated receptor-alpha. Neurotox Res 2011; 19(2): 330–340, https://doi.org/10.1007/s12640-010-9166-2.
- Pazos M.R., Mohammed N., Lafuente H., Santos M., Martínez-Pinilla E., Moreno E., Valdizan E., Romero J., Pazos A., Franco R., Hillard C.J., Alvarez F.J., Martínez-Orgado J. Mechanisms of cannabidiol neuroprotection in hypoxic-ischemic newborn pigs: role of 5HT(1A) and CB2 receptors. Neuropharmacology 2013; 71: 282–291, https://doi.org/10.1016/j.neuropharm.2013.03.027.
- Rosenberg E.C., Patra P.H., Whalley B.J. Therapeutic effects of cannabinoids in animal models of seizures, epilepsy, epileptogenesis, and epilepsy-related neuroprotection. Epilepsy Behav 2017; 70(Pt B): 319–327, https://doi.org/10.1016/j.yebeh.2016.11.006.
- Mori M.A., Meyer E., Soares L.M., Milani H., Guimarães F.S., de Oliveira R.M. Cannabidiol reduces neuroinflammation and promotes neuroplasticity and functional recovery after brain ischemia. Prog Neuropsychopharmacol Biol Psychiatry 2017; 75: 94–105, https://doi.org/10.1016/j.pnpbp.2016.11.005.
- Pihlaja R., Takkinen J., Eskola O., Vasara J., López-Picón F.R., Haaparanta-Solin M., Rinne J.O. Monoacylglycerol lipase inhibitor JZL184 reduces neuroinflammatory response in APdE9 mice and in adult mouse glial cells. J Neuroinflammation 2015; 12: 81, https://doi.org/10.1186/s12974-015-0305-9.
- Carloni S., Alonso-Alconada D., Girelli S., Duranti A., Tontini A., Piomelli D., Hilario E., Alvarez A., Balduini W. Pretreatment with the monoacylglycerol lipase inhibitor URB602 protects from the long-term consequences of neonatal hypoxic-ischemic brain injury in rats. Pediatr Res 2012; 72(4): 400–406, https://doi.org/10.1038/pr.2012.91.
- Valdeolivas S., Pazos M.R., Bisogno T., Piscitelli F., Iannotti F.A., Allarà M., Sagredo O., Di Marzo V., Fernández-Ruiz J. The inhibition of 2-arachidonoyl-glycerol (2-AG) biosynthesis, rather than enhancing striatal damage, protects striatal neurons from malonate-induced death: a potential role of cyclooxygenase-2-dependent metabolism of 2-AG. Cell Death Dis 2013; 4(10): e862, https://doi.org/10.1038/cddis.2013.387.
- Celorrio M., Fernández-Suárez D., Rojo-Bustamante E., Echeverry-Alzate V., Ramírez M.J., Hillard C.J., López-Moreno J.A., Maldonado R., Oyarzábal J., Franco R., Aymerich M.S. Fatty acid amide hydrolase inhibition for the symptomatic relief of Parkinson’s disease. Brain Behav Immun 2016; 57: 94–105, https://doi.org/10.1016/j.bbi.2016.06.010.
- Tchantchou F., Tucker L.B., Fu A.H., Bluett R.J., McCabe J.T., Patel S., Zhang Y. The fatty acid amide hydrolase inhibitor PF-3845 promotes neuronal survival, attenuates inflammation and improves functional recovery in mice with traumatic brain injury. Neuropharmacology 2014; 85: 427–39, https://doi.org/10.1016/j.neuropharm.2014.06.006.
- Su S.H., Wang Y.Q., Wu Y.F., Wang D.P., Lin Q., Hai J. Cannabinoid receptor agonist WIN55,212-2 and fatty acid amide hydrolase inhibitor URB597 may protect against cognitive impairment in rats of chronic cerebral hypoperfusion via PI3K/AKT signaling. Behav Brain Res 2016; 313: 334–344, https://doi.org/10.1016/j.bbr.2016.07.009.
- England T.J., Hind W.H., Rasid N.A., O’Sullivan S.E. Cannabinoids in experimental stroke: a systematic review and meta-analysis. J Cereb Blood Flow Metab 2015; 35(3): 348–358, https://doi.org/10.1038/jcbfm.2014.218.
- Hind W.H., England T.J., O’Sullivan S.E. Cannabidiol protects an in vitro model of the blood-brain barrier from oxygen-glucose deprivation via PPARγ and 5-HT1A receptors. Br J Pharmacol 2016; 173(5): 815–825, https://doi.org/10.1111/bph.13368.
- Mukhina I.V., Kazantsev V.B., Khaspeckov L.G., Zakharov Yu.N., Vedunova M.V., Mitroshina E.V., Korotchenko S.A., Koryagina E.A. Multielectrode matrices — new possibilities in investigation of the neuronal network plasticity. Sovremennye tehnologii v medicine 2009; 1: 8–15.
- Vedunova M.V., Mishchenko T.A., Mitroshina E.V., Mukhina I.V. TrkB-mediated neuroprotective and antihypoxic properties of brain-derived neurotrophic factor. Oxid Med Cell Longev 2015; 2015: 453901, https://doi.org/10.1155/2015/453901.
- Paredes M., Etzler J.C., Watts L.T., Zheng W., Lechleiter J.D. Chemical calcium indicators. Methods 2008; 46(3): 143–151, https://doi.org/10.1016/j.ymeth.2008.09.025.
- Zakharov Yu.N., Korotchenko S.A., Kalintseva Ya.I., Potanina A.V., Mitroshina E.V., Vedunova M.V., Mukhina I.V. Fluorescence analysis of the metabolic activity patterns of a neuronal-glial network. Journal of Optical Technology 2012; 79(6): 348–351, https://doi.org/10.1364/jot.79.000348.
- Rosi N.L., Giljohann D.A., Thaxton C.S., Lytton-Jean A.K., Han M.S., Mirkin C.A. Oligonucleotide-modified gold nanoparticles for intracellular gene regulation. Science 2006; 12(5776): 1027–1030, https://doi.org/10.1126/science.1125559.
- Yuste R. From the neuron doctrine to neural networks. Nat Rev Neurosci 2015; 16(8): 487–497, https://doi.org/10.1038/nrn3962.
- Grabiec U., Koch M., Kallendrusch S., Kraft R., Hill K., Merkwitz C., Ghadban C., Lutz B., Straiker A., Dehghani F. The endocannabinoid N-
arachidonoyldopamine (NADA) exerts neuroprotective effects after excitotoxic neuronal damage via cannabinoid receptor 1 (CB1). Neuropharmacology 2012; 62(4): 1797–1807, https://doi.org/10.1016/j.neuropharm.2011.11.023. - Panikashvili D., Mechoulam R., Beni S.M., Alexandrovich A., Shohami E. CB1 cannabinoid receptors are involved in neuroprotection via NF-κB inhibition. J Cereb Blood Flow Metab 2005; 25(4): 477–484, https://doi.org/10.1038/sj.jcbfm.9600047.
- Marsicano G., Moosmann B., Hermann H., Lutz B., Behl C. Neuroprotective properties of cannabinoids against oxidative stress:
role of the cannabinoid receptor CB1. J Neurochem 2002; 80(3): 448–456, https://doi.org/10.1046/j.0022-3042.2001.00716.x. - Ma L., Jia J., Niu W., Jiang T., Zhai Q., Yang L., Bai F., Wang Q., Xiong L. Mitochondrial CB1 receptor is involved in ACEA-induced protective effects on neurons and mitochondrial functions. Sci Rep 2015; 5(1): 12440, https://doi.org/10.1038/srep12440.
- Xu C., Hermes D.J., Nwanguma B., Jacobs I.R., Mackie K., Mukhopadhyay S., Lichtman A.H., Ignatowska-Jankowska B., Fitting S. Endocannabinoids exert CB1 receptor-mediated neuroprotective effects in models of neuronal damage induced by HIV-1 Tat protein. Mol Cell Neurosci 2017; 83: 92–102, https://doi.org/10.1016/j.mcn.2017.07.003.
- Zhuang Q., Dai C., Yang L., Wen H., Wang H., Jiang X., Zhang Y. Stimulated CB1 cannabinoid receptor inducing ischemic tolerance and protecting neuron from cerebral ischemia. Cent Nerv Syst Agents Med Chem 2017; 17(2): 141–150, https://doi.org/10.2174/1871524916666160504104624.
- Smith T.G.,
Roblins P.A.,Ratelife P.J. The human side of hypoxia-inducible factor. Br J Haematol 2008; 141(3): 325–334, https://doi.org/10.1111/j.1365-2141.2008.07029.x. - Semenza G.L. Hydroxylation of HIF-1: oxygen sensing at the molecular level. Physiology (Bethesda) 2004; 19(4): 176–182, https://doi.org/10.1152/physiol.00001.2004.
- Semenza G.L. Regulation of physiological responses to continuous and intermittent hypoxia by hypoxia-inducible factor 1. Exp Physiol 2006; 91(5): 803–806, https://doi.org/10.1113/expphysiol.2006.033498.
- Singh N., Sharma G., Mishra V., Raghubir R.
Hypoxia inducible factor-1: its potential role in cerebral ischemia. Cell Mol Neurobiol 2012; 32(4): 491–507, https://doi.org/10.1007/s10571-012-9803-9. - Soler-Torronteras R., Lara-Chica M., García V., Calzado M.A., Muñoz E. Hypoximimetic activity of N-acyl-dopamines. N-arachidonoyl-dopamine stabilizes HIF-1α protein through a SIAH2-dependent pathway. Biochim Biophys Acta 2014; 1843(11): 2730–2743, https://doi.org/10.1016/j.bbamcr.2014.07.005.
- Abán C., Martinez N., Carou C., Albamonte I., Toro A., Seyahian A., Franchi A., Leguizamón G., Trigubo D., Damiano A., Farina M. Endocannabinoids participate in placental apoptosis induced by hypoxia inducible factor-1. Apoptosis 2016; 21(10): 1094–1105, https://doi.org/10.1007/s10495-016-1274-x.
- Adamcio B., Sperling S., Hagemeyer N., Walkinshaw G., Ehrenreich H. Hypoxia inducible factor stabilization leads to lasting improvement of hippocampal memory in healthy mice. Behav Brain Res 2010; 208(1): 80–44, https://doi.org/10.1016/j.bbr.2009.11.010.
- Liu X.L., Lu J., Xing J. Stabilization of HIF-1α modulates VEGF and Caspase-3 in the hippocampus of rats following transient global ischemia induced by asphyxial cardiac arrest. Life Sci 2016; 151: 243–249, https://doi.org/10.1016/j.lfs.2016.03.005.
- Zhu T., Zhan L., Liang D., Hu J., Lu Z., Zhu X., Sun W., Liu L., Xu E. Hypoxia-inducible factor 1α mediates neuroprotection of hypoxic postconditioning against global cerebral ischemia. J Neuropathol Exp Neurol 2014; 73(10): 975–986, https://doi.org/10.1097/nen.0000000000000118.
- Reischl S., Li L., Walkinshaw G., Flippin L.A., Marti H.H., Kunze R. Inhibition of HIF prolyl-4-hydroxylases by FG-4497 reduces brain tissue injury and edema formation during ischemic stroke. PLoS One 2014; 9(1): e84767, https://doi.org/10.1371/journal.pone.0084767.
- Yang Y., Ju J., Deng M., Wang J., Liu H., Xiong L., Zhang J. Hypoxia inducible factor 1α promotes endogenous adaptive response in rat model of chronic cerebral hypoperfusion. Int J Mol Sci 2017; 18(1): 3, https://doi.org/10.3390/ijms18010003.
- Yan J., Huang Y., Lu Y., Chen J., Jiang H. Repeated administration of ketamine can induce hippocampal neurodegeneration and long-term cognitive impairment via the ROS/HIF-1α pathway in developing rats. Cell Physiol Biochem 2014; 33(6): 1715–1732, https://doi.org/10.1159/000362953.
- Yin R., Yuan L., Ping L., Hu L. Neonatal bronchopulmonary dysplasia increases neuronal apoptosis in the hippocampus through the HIF-1α and p53 pathways. Respir Physiol Neurobiol 2016; 220: 81–87, https://doi.org/10.1016/j.resp.2015.09.011.
- Li A., Sun X., Ni Y., Chen X., Guo A. HIF-1α involves in neuronal apoptosis after traumatic brain injury in adult rats. J Mol Neurosci 2013; 51(3): 1052–1062, https://doi.org/10.1007/s12031-013-0084-7.