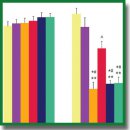
Transplantation of Neural Progenitor Cells in Hyaluronic Acid Hydrogel in Traumatic Brain Injury in Experiment
The aim of the study was to evaluate the possibilities of transplantation of autologous neural progenitor cells from C57BL/6 mouse nasal olfactory lamina propria in the hydrogel based on low-, medium-, and high-molecular hyaluronic acid during a reconstructive operation after an open traumatic brain injury (TBI) in experiment.
Materials and Methods. A reconstructive therapy was performed on the model of an open TBI using the autologous neural progenitor cells from C57BL/6 mouse nasal olfactory lamina propria in a hydrogel based on high, medium and low molecular hyaluronic acid 7 days after injury. Short-term and long-term impairments of neurological functions and memory due to TBI were assessed using a series of behavioral and cognitive tests (modified neurological severity score (mNSS)), open field test, novel object recognition test and passive avoidance behavior). High-field magnetic resonance tomography was used for visualization of a lesion cavity.
Results. Implantation of autologous neural progenitor cells in the hydrogel based on high-molecular weight hyaluronic acid in contrast to low- and medium-molecular weight had a protective effect reducing neurological deficit and restoring motor functions, short-term and long-term memory in the posttraumatic period. The effect of hydrogel molecular weight on the morphological characteristics of the injury cavity was less effective during the autologous neural progenitor cells transplantation after TBI.
Conclusion. Transplantation of autologous neural progenitor cells from C57BL/6 mouse nasal olfactory lamina propria in the hydrogel based on high-molecular weight hyaluronic acid into the injury focus after open brain trauma promotes functional recovery of reflex and cognitive behavior of animals in the posttraumatic period.
- Gusev E.I., Konovalov A.N., Skvortsova V.I., Gekht A.B. Nevrologiya [Neurology]. Moscow: GEOTAR-Media; 2009; 1040 p.
- Bullock M.R., Chesnut R., Ghajar J., Gordon D., Hartl R., Newell D.W., Servadei F., Walters B.C., Wilberger J.E. Guidelines for the surgical management of traumatic brain injury author group. Neurosurgery 2006; 58(3): S2–Vi, https://doi.org/10.1093/neurosurgery/58.3.vi.
- Mayo Clinic Staff. Treatments and drugs. In: Traumatic brain injury. Mayo Clinic; 2014. URL: https://www.mayoclinic.org/diseases-conditions/traumatic-brain-injury/basics/treatment/con-20029302.
- Dobrowolski S., Lepski G. Stem cells in traumatic brain injury. Am J Neurosci 2013; 4(1): 13–24, https://doi.org/10.3844/amjnsp.2013.13.24.
- Crompton K.E., Goud J.D., Bellamkonda R.V., Gengenbach T.R., Finkelstein D.I., Horne M.K., Forsythe J.S. Polylysine-functionalised thermoresponsive chitosan hydrogel for neural tissue engineering. Biomaterials 2007; 28(3): 441–449, https://doi.org/10.1016/j.biomaterials.2006.08.044.
- Tate C.C., Shear D.A., Tate M.C., Archer D.R., Stein D.G., LaPlaca M.C. Laminin and fibronectin scaffolds enhance neural stem cell transplantation into the injured brain. J Tissue Eng Regen Med 2009; 3(3): 208–217, https://doi.org/10.1002/term.154.
- Mo L., Yang Z., Zhang A., Li X. The repair of the injured adult rat hippocampus with NT-3-chitosan carriers. Biomaterials 2010; 31(8): 2184–2192, https://doi.org/10.1016/j.biomaterials.2009.11.078.
- Cao Z. Developing chitosan-based biomaterials for brain repair and neuroprosthetics. Master’s Thesis. University of Tennessee; 2010. URL: http://trace.tennessee.edu/utk_gradthes/609/.
- Engler A.J., Sen S., Sweeney H.L., Discher D.E. Matrix elasticity directs stem cell lineage specification. Cell 2006; 126(4): 677–689, https://doi.org/10.1016/j.cell.2006.06.044.
- Liao H., Munoz-Pinto D., Qu X., Hou Y., Grunlan M.A., Hahn M.S. Influence of hydrogel mechanical properties and mesh size on vocal fold fibroblast extracellular matrix production and phenotype. Acta Biomater 2008; 4(5): 1161–1171, https://doi.org/10.1016/j.actbio.2008.04.013.
- Wlodarczyk J., Mukhina I., Kaczmarek L., Dityatev A. Extracellular matrix molecules, their receptors, and secreted proteases in synaptic plasticity. Dev Neurobiol 2011; 71(11): 1040–1053, https://doi.org/10.1002/dneu.20958.
- Vedunova M., Sakharnova T., Mitroshina E., Perminova M., Pimashkin A., Zakharov Y., Dityatev A., Mukhina I. Seizure-like activity in hyaluronidase-treated dissociated hippocampal cultures. Front Cell Neurosci 2013; 7: 149, https://doi.org/10.3389/fncel.2013.00149.
- Balyabin A.V., Tikhobrazova O.P., Muravyeva M.S., Klyuev E.A., Ponyatovskaya A.V., Shirokova O.M., Bardakova K.N., Minaev N.V., Koroleva A.V., Mitaeva Y.I., Mitroshina E.V., Vedunova M.V., Rochev Y.A., Chichkov B.N., Timashev P.S., Bagratashvili V.N., Mukhina I.V. Long-term neurological and behavioral results of biodegradable scaffold implantation in mice brain. Sovremennye tehnologii v medicine 2016; 8(4): 198–211, https://doi.org/10.17691/stm2016.8.4.25.
- Feeney D.M., Boyeson M.G., Linn R.T., Murray H.M., Dail W.G. Responses to cortical injury: I. Methodology and local effects of contusions in the rat. Brain Res 1981; 211(1): 67–77, https://doi.org/10.1016/0006-8993(81)90067-6.
- Beni-Adani L., Gozes I., Cohen Y., Assaf Y., Steingart R.A., Brenneman D.E., Eizenberg O., Trembolver V., Shohami E. A peptide derived from activity-dependent neuroprotective protein (ADNP) ameliorates injury response in closed head injury in mice. J Pharmacol Exp Ther 2001; 296(1): 57–63.
- Levy A., Bercovich-Kinori A., Alexandrovich A.G., Tsenter J., Trembovler V., Lund F.E., Shohami E., Stein R., Mayo L. CD38 facilitates recovery from traumatic brain injury. J Neurotrauma 2009; 26(9): 1521–1533, https://doi.org/10.1089/neu.2008.0746.
- Beni-Adani L., Eizenberg O., Cohen Y. Correlation between neurological severity score and T2-weighted MRI in head injured mice. Rest Neurol Neurosci 2000; 16(3–4): 242.
- Tsenter J., Beni-Adani L., Assaf Y., Alexandrovich A.G., Trembovler V., Shohami E. Dynamic changes in the recovery after traumatic brain injury in mice: effect of injury severity on T2-weighted MRI abnormalities, and motor and cognitive functions. J Neurotrauma 2008; 25(4): 324–333, https://doi.org/10.1089/neu.2007.0452.
- Bures J., Bureshova O., Huston J.P. Metodiki i osnovnye eksperimenty po izucheniyu mozga i povedeniya [Techniques and basic experiments on the study of the brain and behavior]. Moscow: Vysshaya shkola; 1991; 399 p.
- Huang T.N., Chuang H.C., Chou W.H., Chen C.Y., Wang H.F., Chou S.J., Hsueh Y.P. Tbr1 haploinsufficiency impairs amygdalar axonal projections and results in cognitive abnormality. Nat Neurosci 2014; 17(2): 240–247, https://doi.org/10.1038/nn.3626.
- Moscardo E., Salvetti B., Becchi S., Bertini G., Fabene P.F. The novel object recognition test in rodents: which are the essential methodological aspects? In: Proceedings of Measuring Behavior 2012, 8 th International Conference on Methods and Techniques in Behavioral Research. Spink A.J., Grieco F., Krips O.E., Loijens L.W.S., Noldus L.P.J.J., Zimmerman P.H. (editors). The Netherlands; 2012; p. 476–478.