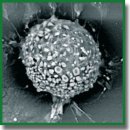
Features of Primary Hippocampal Cultures Formation on Scaffolds Based on Hyaluronic Acid Glycidyl Methacrylate
The aim of the study was to investigate the morphological and metabolic features of primary hippocampal cultures formation on hydrogel films and scaffolds based on hyaluronic acid glycidyl methacrylate.
Materials and Methods. Hydrogel films and scaffolds with certain architectonics were developed by micromolding technique on the basis of hyaluronic acid glycidyl methacrylate. Primary hippocampal cells obtained from С57ВL/6 mouse embryos (E18) were cultured on the created constructs more than 14 days. Testing cell viability, morphometric assessment, and analysis of spontaneous calcium activity of primary hippocampal cultures were performed on day 14 of cultures development in vitro.
Results. This study revealed that the material for the development of scaffolds with given architectonics is non-toxic for the nervous system cells. Dissociated hippocampal cells were actively attached to the scaffold surface and were assembled into cell conglomerates, which exhibited spontaneous calcium activity.
Conclusion. Scaffolds designed on the basis hyaluronic acid glycidyl methacrylate have a high biocompatibility with the nervous system cells. Architectonics and adhesive properties of scaffold contribute to the formation of functionally active cell conglomerates.
- Steinhoff G. Regenerative medicine: from protocol to
patient . Springer Science+Business Media B.V.; 2011; 1055 p, https://doi.org/10.1007/978-90-481-9075-1. Maghdouri -White Y., Bowlin G.L., Lemmon C.A., Dréau D. Mammary epithelial cell adhesion, viability, and infiltrationon blended or coated silk fibroin-collagen type I electrospun scaffolds. Mater Sci Eng C Mater Biol Appl 2014; 43: 37–44, https://doi.org/10.1016/j.msec.2014.06.037.- Kaneko A., Matsushita A., Sankai Y. A 3D nanofibrous hydrogel and collagen sponge scaffold
promotes locomotor functional recovery, spinal repair, and neuronal regeneration after complete transection of the spinal cord inadult rat. Biomed Mater 2015; 10(1): 015008, https://doi.org/10.1088/1748-6041/10/1/015008. - Hosseinkhani M., Mehrabani D.,
Karimfar M.H., Bakhtiyari S., Manafi A., Shirazi R. Tissue engineered scaffolds in regenerative medicine. World J Plast Surg 2014; 3(1): 3–7. - Kim Y.B., Kim G.H. PCL/alginate composite scaffolds for hard tissue engineering: fabrication, characterization, and cellular activities. ACS Comb Sci 2015; 17(2): 87–99, https://doi.org/10.1021/co500033h.
- Murphy S.V., Atala A. 3D bioprinting of tissues and organs. Nat Biotechnol 2014; 32(8): 773–785, https://doi.org/10.1038/nbt.2958.
Levingstone T.J., Thompson E., Matsiko A., Schepens A., Gleeson J.P., O’Brien F.J. Multi-layered collagen-based scaffolds for osteochondral defect repair in rabbits. Acta Biomater 2016; 32: 149–160, https://doi.org/10.1016/j.actbio.2015.12.034.- Lotfi M., Bagherzadeh R., Naderi-Meshkin H., Mahdipour E., Mafinezhad A., Sadeghnia H.R., Esmaily H., Maleki M., Hasssanzadeh H., Ghayaour-Mobarhan M., Bidkhori H.R., Bahrami A.R. Hybrid chitosan-ß-glycerol phosphate-gelatin nano-/micro fibrous scaffolds with suitable mechanical and biological properties for tissue engineering. Biopolymers 2016; 105(3): 163–175, https://doi.org/10.1002/bip.22764.
- Miranda D.G., Malmonge S.M., Campos D.M., Attik N.G., Grosgogeat B., Gritsch K. A chitosan-hyaluronic acid hydrogel scaffold for periodontal tissue engineering. J Biomed Mater Res B Appl Biomater 2016; 104(8): 1691–1702, https://doi.org/10.1002/jbm.b.33516.
- Cui N., Qian J., Liu T., Zhao N., Wang H. Hyaluronic acid hydrogel scaffolds with a triple degradation behavior for bone tissue engineering. Carbohydr Polym 2015; 126: 192–198, https://doi.org/10.1016/j.carbpol.2015.03.013.
- Hsieh F.Y., Lin H.H., Hsu S.H. 3D bioprinting of neural stem cell-laden thermoresponsive biodegradable polyurethane hydrogel and potential
in central nervous system repair. Biomaterials 2015; 71: 48–57, https://doi.org/10.1016/j.biomaterials.2015.08.028. - Raspa A., Marchini A., Pugliese R., Mauri M., Maleki M., Vasita R.,
Gelain F. A biocompatibility study of new nanofibrous scaffolds for nervous system regeneration. Nanoscale 2016; 8(1): 253–265, https://doi.org/10.1039/c5nr03698d. - Kufelt O., El-Tamer A., Sehring C., Schlie-Wolter S., Chichkov B.N. Hyaluronic
acid based materials for scaffolding via two-photon polymerization. Biomacromolecules 2014; 15(2): 650–659, https://doi.org/10.1021/bm401712q. - Drobnik J., Pietrucha K., Piera L., Szymański J., Szczepanowska A. Collagenous scaffolds supplemented with hyaluronic acid and chondroitin sulfate used for wound fibroblast and embryonic nerve cell culture. Adv Clin Exp Med 2017; 26(2): 223–230.
- Kushchayev S.V., Giers M.B., Hom Eng D., Martirosyan N.L., Eschbacher J.M., Mortazavi M.M., Theodore N., Panitch A.,
Preul M.C. Hyaluronic acid scaffold has a neuroprotective effect in hemisection spinal cord injury. J Neurosurg Spine 2016; 25(1): 114–124, https://doi.org/10.3171/2015.9.spine15628. - Wang T.W., Spector M. Development of hyaluronic acid-based scaffolds for brain tissue engineering. Acta Biomater 2009; 5(7): 2371–2384, https://doi.org/10.1016/j.actbio.2009.03.033.
- Rusakov D.A., Dityatev A.E. Brain circuitry outside the synaptic cleft. Philos Trans R Soc Lond B Biol Sci 2014; 369(1654): 20130591, https://doi.org/10.1098/rstb.2013.0591.
- Kochlamazashvili G., Henneberger C., Bukalo O., Dvoretskova E., Senkov O., Lievens P.M., Westenbroek R., Engel A.K., Catterall W.A., Rusakov D.A., Schachner M., Dityatev A. The extracellular matrix molecule hyaluronic acid regulates hippocampal synaptic plasticity by modulating postsynaptic L-type Ca(2+) channels. Neuron 2010; 67(1): 116–128, https://doi.org/10.1016/j.neuron.2010.05.030.
- Savelyev A.G., Bardakova K.N., Khaydukov E.V., Generalova A.N., Popov V.K., Chichkov B.N., Semchishen V.A. Flavin mononucleotide photoinitiated cross-linking of hydrogels: polymer concentration threshold of strengthening. J Photochem Photobiol A Chem 2017; 341: 108–114, https://doi.org/10.1016/j.jphotochem.2017.03.026.
- Vedunova M.V., Mishchenko T.A., Mitroshina E.V., Mukhina I.V. TrkB-mediated neuroprotective and antihypoxic properties of brain-derived neurotrophic factor. Oxid Med Cell Longev 2015; 2015: 453901, https://doi.org/10.1155/2015/453901.
- Zakharov Yu.N., Korotchenko S.A., Kalintseva Ya.I., Potanina A.V., Mitroshina E.V., Vedunova M.V., Mukhina I.V. Fluorescence analysis of the metabolic activity patterns of a neuronal-glial network. Journal of Optical Technology 2012; 79(6): 348–351, https://doi.org/10.1364/jot.79.000348.
- Shirokova О.М., Frumkina L.Е., Vedunova М.V., Mitroshina Е.V., Zakharov Y.N., Khaspekov L.G., Mukhina I.V. Morphofunctional patterns of neuronal network developing in dissociated hippocampal cell cultures. Sovremennye technologii v medicine 2013; 5(2): 6–13.
- Dityatev A. Remodeling of extracellular matrix and epileptogenesis. Epilepsia 2010; 51: 61–65, https://doi.org/10.1111/j.1528-1167.2010.02612.x.