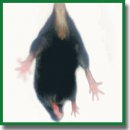
Phenotypic Variations in the Behavior of Sip1 Knockout Mice
The aim of the study was the behavioral phenotyping of mice homo- and heterozygous for the Sip1 gene, which plays an important role in the development of the mammalian cerebral cortex.
Materials and Methods. The study was performed on mice hetero- and homozygous for the Sip1 gene; these animal models were developed using the Cre recombination method. At an age of 20–30 days, all animals were exposed to a high-intensity sound to identify predisposition to audiogenic epilepsy. At an age of two months, the males were tested for their general physical health and behavioral phenotypes. The tests included a neurological and sensorimotor assessment, an evaluation of anxiety using the light-dark test, a study on locomotion and general exploration in the open field test, the acoustic startle response and prepulse inhibition, social activity in the Crawley’s test and the learning ability as scored by the conditioned reflex of passive avoidance.
Results. Mice homozygous for the Sip1 gene never reached the age of two months. In heterozygous mice, a higher occurrence of hind limb extension reflex abnormalities, an increased level of anxiety in the light-dark test, and a decrease in social activity in the Crawley’s test were found.
Conclusion. The presence of a mutant allele of the Sip1 gene leads to neurologic disorders, an increase in anxiety and a decrease in the social activity of the animals.
- Fernández V., Llinares-Benadero C., Borrell V. Cerebral cortex expansion and folding: what have we learned? EMBO J 2016; 35(10): 1021–1044, https://doi.org/10.15252/embj.201593701.
- Elsen G.E., Hodge R.D., Bedogni F., Daza R.A.M., Nelson B.R., Shiba N., Reiner S.L., Hevner R.F. The protomap is propagated to cortical plate neurons through an Eomes-dependent intermediate map. Proc Natl Acad Sci USA 2013; 110(10): 4081–4086, https://doi.org/10.1073/pnas.1209076110.
- De Juan Romero C., Borrell V. Coevolution of radial glial cells and the cerebral cortex. Glia 2015; 63(8): 1303–1319, https://doi.org/10.1002/glia.22827.
- Goossens S., Janzen V., Bartunkova S., Yokomizo T., Drogat B., Crisan M., Haigh K., Seuntjens E., Umans L., Riedt T., Bogaert P., Haenebalcke L., Berx G., Dzierzak E., Huylebroeck D., Haigh J.J. The EMT regulator Zeb2/Sip1 is essential for murine embryonic hematopoietic stem/progenitor cell differentiation and mobilization. Blood 2011; 117(21): 5620–5630, https://doi.org/10.1182/blood-2010-08-300236.
- Manthey A.L., Lachke S.A., FitzGerald P.G., Mason R.W., Scheiblin D.A., McDonald J.H., Duncan M.K. Loss of Sip1 leads to migration defects and retention of ectodermal markers during lens development. Mech Dev 2014; 131: 86–110, https://doi.org/10.1016/j.mod.2013.09.005.
- Teraishi M., Takaishi M., Nakajima K., Ikeda M., Higashi Y., Shimoda S., Asada Y., Hijikata A., Ohara O., Hiraki Y., Mizuno S., Fukada T., Furukawa T., Wakamatsu N., Sano S. Critical involvement of ZEB2 in collagen fibrillogenesis: the molecular similarity between Mowat-Wilson syndrome and Ehlers-Danlos syndrome. Sci Rep 2017; 7: 46565, https://doi.org/10.1038/srep46565.
- Garavelli L., Mainardi P. Mowat-Wilson syndrome. Orphanet J Rare Dis 2007; 2(1): 42, https://doi.org/10.1186/1750-1172-2-42.
- Srivatsa S., Parthasarathy S., Molnár Z., Tarabykin V. Sip1 downstream Effector ninein controls neocortical axonal growth, ipsilateral branching, and microtubule growth and stability. Neuron 2015; 5(5): 998–1012, https://doi.org/10.1016/j.neuron.2015.01.018.
- Turovskaya M.V., Babaev A.A., Zinchenko V.P., Epifanova E.A., Borisova E.V., Tarabykin V.S., Turovsky E.A. Sip-1 mutations cause disturbances in the activity of NMDA- and AMPA-, but not kainate receptors of neurons in the cerebral cortex. Neurosci Lett 2017; 650: 180–186, https://doi.org/10.1016/j.neulet.2017.04.048.
- Higashi Y., Maruhashi M., Nelles L., Van de Putte T., Verschueren K., Miyoshi T., Yoshimoto A., Kondoh H., Huylebroeck D. Generation of the floxed allele of the SIP1 (Smad-interacting protein 1) gene for Cre-mediated conditional knockout in the mouse. Genesis 2002; 32(2): 82–84, https://doi.org/10.1002/gene.10048.
- Goebbels S., Bormuth I., Bode U., Hermanson O., Schwab M.H., Nave K.-A. Genetic targeting of principal neurons in neocortex and hippocampus of NEX-Cre mice. Genesis 2006; 44(12): 611–621, https://doi.org/10.1002/dvg.20256.
- Seuntjens E., Nityanandam A., Miquelajauregui A., Debruyn J., Stryjewska A., Goebbels S., Nave K.A., Huylebroeck D., Tarabykin V. Sip1 regulates sequential fate decisions by feedback signaling from postmitotic neurons to progenitors. Nat Neurosci 2009; 12(11): 1373–1380, https://doi.org/10.1038/nn.2409.
- Gorski J.A., Talley T., Qiu M., Puelles L., Rubenstein J.L., Jones K.R. Cortical excitatory neurons and glia, but not GABAergic neurons, are produced in the Emx1-expressing lineage. J Neurosci 2002; 22(15): 6309–6314.
- Chabrol E., Navarro V., Provenzano G., Cohen I., Dinocourt C., Rivaud-Péchoux S., Fricker D., Baulac M., Miles R., Leguern E., Baulac S. Electroclinical characterization of epileptic seizures in leucine-rich, glioma-inactivated 1-deficient mice. Brain 2010; 133(9): 2749–2762, https://doi.org/10.1093/brain/awq171.
- Semiokhina A.F., Fedotova I.B., Poletaeva I.I. Rats of Krushinsky-Molodkina strain: studies of audiogenic epilepsy, vascular pathology and behavior. Zhurnal vysshei nervnoi deyatelnosti imeni I.P. Pavlova 2006; 56(3): 298–316.
- Silva A.F., Sousa D.S., Medeiros A.M., Macêdo P.T., Leão A.H., Ribeiro A.M., Izídio G.S., Silva R.H. Sex and estrous cycle influence diazepam effects on anxiety and memory: Possible role of progesterone. Prog Neuropsychopharmacol Biol Psychiatry 2016; 70: 68–76, https://doi.org/10.1016/j.pnpbp.2016.05.003.
- Kiryk A., Aida T., Tanaka K., Banerjee P., Wilczynski G.M., Meyza K., Knapska E., Filipkowski R.K., Kaczmarek L., Danysz W. Behavioral characterization of GLT1 (+/–) mice as a model of mild glutamatergic hyperfunction. Neurotox Res 2008; 13(1): 19–30, https://doi.org/10.1007/bf03033364.
- Rosen J.B., Schulkin J. From normal fear to pathological anxiety. Psychol Rev 1998; 105(2): 325–350, https://doi.org/10.1037/0033-295x.105.2.325.
- Crawley J.N. Designing mouse behavioral tasks relevant to autistic-like behaviors. Ment Retard Dev Disabil Res Rev 2004; 10(4): 248–258, https://doi.org/10.1002/mrdd.20039.
- Kaidanovich-Beilin O., Lipina T., Vukobradovic I., Roder J., Woodgett J.R. Assessment of social interaction behaviors. J Vis Exp 2011; 48: 2473, https://doi.org/10.3791/2473.
- Crawley J.N. Behavioral phenotyping strategies for mutant mice. Neuron 2008; 57(6): 809–818, https://doi.org/10.1016/j.neuron.2008.03.001.
- Lalonde R., Strazielle C. Motor performance and regional brain metabolism of spontaneous murine mutations with cerebellar atrophy. Behav Brain Res 2001; 125(1–2): 103–108, https://doi.org/10.1016/s0166-4328(01)00276-5.
- Komatsu M., Waguri S., Chiba T., Murata S., Iwata J., Tanida I., Ueno T., Koike M., Uchiyama Y., Kominami E., Tanaka K. Loss of autophagy in the central nervous system causes neurodegeneration in mice. Nature 2006; 441(7095): 880–884, https://doi.org/10.1038/nature04723.
- Wang X., Bowers S.L., Wang F., Pu X., Nelson R.J., Ma J. Cytoplasmic prion protein induces forebrain neurotoxicity. Biochim Biophys Acta 2009; 1792(6): 555–563, https://doi.org/10.1016/j.bbadis.2009.02.014.
- Lee L.-J., Chen W.-J., Chuang Y.-W., Wang Y.-C. Neonatal whisker trimming causes long-lasting changes in structure and function of the somatosensory system. Exp Neurol 2009; 219(2): 524–532, https://doi.org/10.1016/j.expneurol.2009.07.012.
- Shishelova A.Yu., Aliev R.R., Raevsky V.V. Early sensory experience determines variety of exploratory behavior in adult age. Eksperimentalnaya psikhologiya 2015; 8(1): 73–84.
- Shishelova A.Y., Raevskii V.V., Aliev R.R. Effect of early sensory experience on the exploratory activity in adult animals. Doklady Biological Sciences 2016; 468(1): 101–103, https://doi.org/10.1134/s0012496616030029.
- Krushinskiy L.V. The new in the study of experimental epilepsy and its underlying physiological mechanisms. Uspekhi sovremennoi biologii 1949; 28: 108–133.
- Hascoët M., Bourin M., Nic Dhonnchadha B.Á. The mouse ligth-dark paradigm: a review. Prog Neuropsychopharmacol Biol Psychiatry 2001; 25(1): 141–166, https://doi.org/10.1016/s0278-5846(00)00151-2.
- Denenberg V.H. Open-field behavior in the rat: what does it mean? Ann N Y Acad Sci 1969; 159(3): 852–859, https://doi.org/10.1111/j.1749-6632.1969.tb12983.x.
- Bailey K.R., Rustay N.R., Crawley J.N. Behavioral phenotyping of transgenic and knockout mice: practical concerns and potential pitfalls. ILAR J 2006; 47(2): 124–131, https://doi.org/10.1093/ilar.47.2.124.
- Popova N.K., Barykina N.N., Pliusnina I.Z., Alekhina T.A., Kolpakov V.G. Manifestation of fear response in rats genetically predisposed to various kinds of defense behavior. Rossiiskii fiziologicheskii zhurnal imeni I.M. Sechenova 1999; 1: 99–104.
- Tibeykina M.A. Genetiko-fiziologicheskie osobennosti akusticheskoy reaktsii vzdragivaniya: vzaimosvyaz’ s drugimi formami povedeniya. Dis. … kand. biol. nauk [Genetic and physiological properties of acoustic startle response: relationship with other forms of behavior. PhD dissertation]. Novosibirsk; 2008.
- Amikishieva A.V. Behavioral phenotyping: up-to-date methods and equipment. Informatsionnyy vestnik VOGiS 2009; 13(3): 529–542.
- Thor D.H., Holloway W.R. Anosmia and play fighting behavior in prepubescent male and female rats. Physiol Behav 1982; 29(2): 281–285, https://doi.org/10.1016/0031-9384(82)90016-6.
- Takagi T., Nishizaki Y., Matsui F., Wakamatsu N., Higashi Y. De novo inbred heterozygous Zeb2/Sip1 mutant mice uniquely generated by germ-line conditional knockout exhibit craniofacial, callosal and behavioral defects associated with Mowat-Wilson syndrome. Hum Mol Genet 2015; 24(22): 6390–6402, https://doi.org/10.1093/hmg/ddv350.
- Nishizaki Y., Takagi T., Matsui F., Higashi Y. SIP1 expression patterns in brain investigated by generating a SIP1-EGFP reporter knock-in mouse. Genesis 2013; 52(1): 56–67, https://doi.org/10.1002/dvg.22726.
- Overstreet D.H., Commissaris R.C., De La Garza R., File S.E., Knapp D.J., Seiden L.S. Involvement of 5-HT1A receptors in animal tests of anxiety and depression: evidence from genetic models. Stress 2003; 6(2): 101–110, https://doi.org/10.1080/1025389031000111311.
- Naumenko V.S., Ponimaskin E.G., Popova N.K. 5-HT1A receptor: its role in the regulation of different kinds of behavior. Vavilovskii zhurnal genetiki i selektsii 2016; 20(2): 180–190.