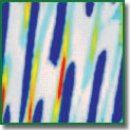
Changes in Theta and Gamma Network Oscillations during the Development of Neurodegenerative Disorders (Review)
Theta and gamma oscillations registered in the hippocampus and neocortex are necessary for cognitive processes in the brain; their alterations are revealed in many neurological and psychiatric diseases. The most common forms of neurodegenerative disorders, Alzheimer’s disease (AD) and temporal lobe epilepsy (TLE) are characterized by the loss of cells and progressive violations of cognitive functions, such as memory. Early diagnosis of diseases is very important for their successful treatment. Many efforts have been done for defining early signs of these diseases. Significant advances have been made in the searching of some AD and TLE reliable biomarkers with using biochemical and imaging approaches. However, there is a great need for the biomarkers that would reflect changes of brain activity within few milliseconds to obtain information about cognitive disturbances.
In the present review, the data of recent literature specifying that the coherent analysis of the theta and gamma oscillations can be used in early diagnostics of TLE and AD are considered. These data show that in a brain with the developing neurodegenerative disorder the specific violations of the theta and gamma interaction are observed. We summarize here the data on the alterations of the theta and gamma coherence based on examples from TLE and AD and from models of these diseases. The specific disturbances in interactions of theta–gamma oscillations in hippocampal, hippocampal–entorhinal, hippocampal–prefrontal, and hippocampal–septal networks were revealed in the epileptic brain. In the AD models, marked changes were observed in the theta–gamma coupling in the subiculum, an output region of the hippocampus. In addition, a decreased theta–gamma interaction between the hippocampus and the parietal cortex as well as between the hippocampus and the prefrontal cortex was also shown.
- Vanderwolf C. Hippocampal electrical activity and voluntary movement in the rat. Electroencephalogr Clin Neurophysiol 1969; 26(4): 407–418, https://doi.org/10.1016/0013-4694(69)90092-3.
- Bland B.H. The physiology and pharmacology of hippocampal formation theta rhythms. Prog Neurobiol 1986; 26(1): 1–54, https://doi.org/10.1016/0301-0082(86)90019-5.
- Buzsáki G. Theta oscillations in the hippocampus. Neuron 2002; 33(3): 325–340, https://doi.org/10.1016/s0896-6273(02)00586-x.
- Klimesch W., Doppelmayr M., Schimke H., Ripper B. Theta synchronization and alpha desynchronization in a memory task. Psychophysiology 1997; 34(2): 169–176, https://doi.org/10.1111/j.1469-8986.1997.tb02128.x.
- Kahana M.J., Sekuler R., Caplan J.B., Kirschen M., Madsen J.R. Human theta oscillations exhibit task dependence during virtual maze navigation. Nature 1999; 399(6738): 781–784, https://doi.org/10.1038/21645.
- Jensen O., Tesche C.D. Frontal theta activity in humans increases with memory load in a working memory task. Eur J Neurosci 2002; 15(8): 1395–1399, https://doi.org/10.1046/j.1460-9568.2002.01975.x.
- Vinogradova O.S. Expression, control, and probable functional significance of the neuronal theta-rhythm. Prog Neurobiol 1995; 45(6): 523–583, https://doi.org/10.1016/0301-0082(94)00051-i.
- Guderian S., Schott B.H., Richardson-Klavehn A., Duzel E. Medial temporal theta state before an event predicts episodic encoding success in humans. Proc Natl Acad Sci U S A 2009; 106(13): 5365–5370, https://doi.org/10.1073/pnas.0900289106.
- Paré D. Amygdala oscillations and the consolidation of emotional memories. Trends Cogn Sci 2002; 6(7): 306–314, https://doi.org/10.1016/s1364-6613(02)01924-1.
- Nerad L., McNaughton N. The septal EEG suggests a distributed organization of the pacemaker of hippocampal theta in the rat. Eur J Neurosci 2006; 24(1): 155–166, https://doi.org/10.1111/j.1460-9568.2006.04902.x.
- Magill P.J., Sharott A., Bolam J.P., Brown P. Delayed synchronization of activity in cortex and subthalamic nucleus following cortical stimulation in the rat. J Physiol 2006; 574(3): 929–946, https://doi.org/10.1113/jphysiol.2006.110379.
- DeCoteau W.E., Thorn C., Gibson D.J., Courtemanche R., Mitra P., Kubota Y., Graybiel A.M. Learning-related coordination of striatal and hippocampal theta rhythms during acquisition of a procedural maze task. Proc Natl Acad Sci U S A 2007; 104(13): 5644–5649, https://doi.org/10.1073/pnas.0700818104.
- Kabanova I.V., Sinelnikova V.V., Popova I.Y., Kichigina V.F., Aliev R.R. Coherence and phase analysis of theta oscillations in the septohippocampal system during generation of convulsive activity. Neurosci Behav
Physi 2013; 43(2): 214–218, https://doi.org/10.1007/s11055-013-9716-1. - Hasselmo M.E., Bodelón C., Wyble B.P. A proposed function for hippocampal theta rhythm: separate phases of encoding and retrieval enhance reversal of prior learning. Neural Comput 2002; 14(4): 793–817, https://doi.org/10.1162/089976602317318965.
- Bragin A., Jando G., Nadasdy Z., Hetke J., Wise K., Buzsaki G. Gamma (40–100 Hz) oscillation in the hippocampus of the behaving rat. J Neurosci 1995; 15(1): 47–60, https://doi.org/10.1523/jneurosci.15-01-00047.1995.
- Strogatz S.H. Nonlinear dynamics and chaos: with applications to physics, biology, chemistry
and engineering. Cambridge, MA: Perseus Books; 2003. - Buzsáki G., Wang X.-J. Mechanisms of gamma oscillations. Annu Rev Neurosci 2012; 35(1): 203–225, https://doi.org/10.1146/annurev-neuro-062111-150444.
- Bouyer J., Montaron M., Rougeul A. Fast
fronto-parietal rhythms during combined focused attentivebehaviour and immobility incat : cortical and thalamic localizations. Electroencephalogr Clin Neurophysiol 1981; 51(3): 244–252, https://doi.org/10.1016/0013-4694(81)90138-3. - Benchenane K., Peyrache A., Khamassi M., Tierney P.L.,
Gioanni Y., Battaglia F.P., Wiener S.I. Coherenttheta oscillations and reorganization of spike timing in the hippocampal-prefrontal network upon learning. Neuron 2010; 66(6): 921–936, https://doi.org/10.1016/j.neuron.2010.05.013. - Fries P. Neuronal gamma-band synchronization as a fundamental process in cortical computation. Annu Rev Neurosci 2009; 32(1): 209–224, https://doi.org/10.1146/annurev.neuro.051508.135603.
- Jutras M.J., Fries P., Buffalo E.A. Gamma-band synchronization in the macaque hippocampus and memory formation. J Neurosci 2009; 29(40): 12521–12531, https://doi.org/10.1523/jneurosci.0640-09.2009.
- Sauseng P., Klimesch W., Heise K.F., Gruber W.R., Holz E., Karim A.A., Glennon M., Gerloff C., Birbaumer N., Hummel F.C. Brain oscillatory substrates of visual short-term memory capacity. Curr Biol 2009; 19(21): 1846–1852, https://doi.org/10.1016/j.cub.2009.08.062.
- Sridharan D., Knudsen E.I. Gamma oscillations in the midbrain spatial attention network: linking circuits to function. Curr Opin Neurobiol 2015; 31: 189–198, https://doi.org/10.1016/j.conb.2014.11.006.
- Canolty R.T., Knight R.T. The functional role of cross-frequency coupling. Trends Cogn Sci 2010; 14(11): 506–515, https://doi.org/10.1016/j.tics.2010.09.001.
- Tort A.B.L., Komorowski R.W., Manns J.R., Kopell N.J., Eichenbaum H.
Theta–gamma coupling increases during the learning of item-context associations. Proc Natl Acad Sci 2009; 106(49): 20942–20947, https://doi.org/10.1073/pnas.0911331106. - Lisman J.E., Jensen O. The theta–gamma neural code. Neuron 2013; 77(6): 1002–1016, https://doi.org/10.1016/j.neuron.2013.03.007.
- Livanov M.N., Krylov V.Yu., Ostrjakova T.V., Shulgina G.I. Slow field potential oscillations as one of the basic mechanisms of integrative activity of neurons [proceedings]. Act Nerv Super (Praha) 1977; 19(1): 43–44.
- Vinogradova O.S. Hippocampus as comparator:
role of the two input and two output systems of the hippocampus in selection and registration of information. Hippocampus 2001; 11(5): 578–598, https://doi.org/10.1002/hipo.1073.abs. - Engel A.K., Fries P., Singer W. Dynamic predictions: oscillations and synchrony in
processing. Nat Rev Neurosci 2001; 2(10): 704–716, https://doi.org/10.1038/35094565.top –down - Igarashi K.M., Lu L., Colgin L.L., Moser M.-B., Moser E.I. Coordination of entorhinal–hippocampal ensemble activity during associative learning. Nature 2014; 510(7503): 143–147, https://doi.org/10.1038/nature13162.
- Fell J., Klaver P., Lehnertz K., Grunwald T., Schaller C., Elger C.E., Fernández G. Human memory formation is accompanied by
rhinal–hippocampal coupling and decoupling. Nat Neurosci 2001; 4(12): 1259–1264, https://doi.org/10.1038/nn759. - Varela F., Lachaux J.-P., Rodriguez E., Martinerie J. The
brainweb : phase synchronization and large-scale integration. Nat Rev Neurosci 2001; 2(4): 229–239, https://doi.org/10.1038/35067550. - Buzsáki G. Large-scale recording of neuronal ensembles. Nat Neurosci 2004; 7(5): 446–451, https://doi.org/10.1038/nn1233.
- Womelsdorf T., Fries P., Mitra P.P., Desimone R. Gamma-band synchronization in visual cortex predicts
speed of change detection. Nature 2005; 439(7077): 733–736, https://doi.org/10.1038/nature04258. - Vinck M., Bos J.J., Van Mourik-Donga L.A., Oplaat K.T., Klein G.A., Jackson J.C., Gentet L.J., Pennartz C.M. Cell-type and state-dependent synchronization among rodent somatosensory, visual, perirhinal cortex, and hippocampus CA1. Front Syst Neurosci 2016; 9: 187, https://doi.org/10.3389/fnsys.2015.00187.
- Astasheva E.V., Kitchigina V.F. Activation of the glutamatergic system of the medial septal area accelerates epileptogenesis. Neurosci Behav
Physi 2011; 41(3): 316–320, https://doi.org/10.1007/s11055-011-9418-5. - Bott J.B., Muller M.A., Jackson J., Aubert J., Cassel J.C., Mathis C., Goutagny R. Spatial reference memory is associated with modulation of
theta–gamma coupling in the dentate gyrus. Cereb Cortex 2015; 26(9): 3744–3753, https://doi.org/10.1093/cercor/bhv177. - Tabuchi E.T., Mulder A.B., Wiener S.I. Position and behavioral modulation of synchronization of hippocampal and
accumbens neuronal discharges in freely moving rats. Hippocampus 2000; 10(6): 717–728, https://doi.org/10.1002/1098-1063(2000)10:6717::aid-hipo10093.0.co;2-3. - Harris K.D., Csicsvari J., Hirase H., Dragoi G., Buzsáki G. Organization of cell assemblies in the hippocampus. Nature 2003; 424(6948): 552–556, https://doi.org/10.1038/nature01834.
- Colgin L.L., Moser E.I. Gamma oscillations in the hippocampus. Physiology 2010; 25(5): 319–329, https://doi.org/10.1152/physiol.00021.2010.
- Buzsáki G. Neural syntax: cell assemblies, synapsembles, and readers. Neuron 2010; 68(3): 362–385, https://doi.org/10.1016/j.neuron.2010.09.023.
- Buzsáki G., Watson B.O. Brain rhythms and neural syntax: implications for efficient coding of cognitive content and neuropsychiatric disease. Dialogues Clin Neurosci 2012; 14(4): 345–367.
- Volgushev M., Chistiakova M., Singer W. Modification of discharge patterns of neocortical neurons by induced oscillations of the membrane potential. Neuroscience 1998; 83(1): 15–25, https://doi.org/10.1016/s0306-4522(97)00380-1.
- Fries P. A mechanism for cognitive dynamics: neuronal communication through neuronal coherence. Trends Cogn Sci 2005; 9(10): 474–480, https://doi.org/10.1016/j.tics.2005.08.011.
- Jensen O., Kaiser J., Lachaux J.-P. Human gamma-frequency oscillations associated with attention and memory. Trends Neurosci 2007; 30(7): 317–324, https://doi.org/10.1016/j.tins.2007.05.001.
- Mitchell D.J., McNaughton N., Flanagan D., Kirk I.J. Frontal-midline theta from the perspective of hippocampal “theta”. Prog Neurobiol 2008; 86(3): 156–185, https://doi.org/10.1016/j.pneurobio.2008.09.005.
- Rutishauser U., Ross I.B., Mamelak A.N., Schuman E.M. Human memory strength is predicted by theta-frequency phase-locking of single neurons. Nature 2010; 464(7290): 903–907, https://doi.org/10.1038/nature08860.
- Wang X.-J. Neurophysiological and computational principles of cortical rhythms in cognition. Physiol Rev 2010; 90(3): 1195–1268, https://doi.org/10.1152/physrev.00035.2008.
- Colgin L.L., Denninger T., Fyhn M., Hafting T., Bonnevie T., Jensen O., Moser M.B., Moser E.I. Frequency of gamma oscillations routes flow of information in the hippocampus. Nature 2009; 462(7271): 353–357, https://doi.org/10.1038/nature08573.
- Colgin L.L. Oscillations and hippocampal–prefrontal synchrony. Curr Opin Neurobiol 2011; 21(3): 467–474, https://doi.org/10.1016/j.conb.2011.04.006.
- Colgin L.L.
Theta–gamma coupling in theentorhinal–hippocampal system. Curr Opin Neurobiol 2015; 31: 45–50, https://doi.org/10.1016/j.conb.2014.08.001. - Bastos A.M., Vezoli J., Fries P. Communication through coherence with inter-areal delays. Curr Opin Neurobiol 2015; 31: 173–180, https://doi.org/10.1016/j.conb.2014.11.001.
- Kirihara K., Rissling A.J., Swerdlow N.R., Braff D.L., Light G.A. Hierarchical organization of gamma and theta oscillatory dynamics in schizophrenia. Biol Psychiatry 2012; 71(10): 873–880, https://doi.org/10.1016/j.biopsych.2012.01.016.
- Froriep U.P., Kumar A., Cosandier-Rimélé D., Häussler U., Kilias A., Haas C.A., Egert U. Altered theta coupling between medial entorhinal cortex and dentate gyrus in temporal lobe epilepsy. Epilepsia 2012; 53(11): 1937–1947, https://doi.org/10.1111/j.1528-1167.2012.03662.x.
- Bakker A., Krauss G.L., Albert M.S., Speck C.L., Jones L.R., Stark C.E., Yassa M.A., Bassett S.S., Shelton A.L., Gallagher M. Reduction of hippocampal hyperactivity improves cognition in amnestic mild cognitive impairment. Neuron 2012; 74(3): 467–474, https://doi.org/10.1016/j.neuron.2012.03.023.
- Inostroza M., Brotons-Mas J.R., Laurent F., Cid E., de la Prida L.M. Specific impairment of “what-where-when” episodic-like memory in experimental models of temporal lobe epilepsy. J Neurosci 2013; 33(45): 17749–17762, https://doi.org/10.1523/jneurosci.0957-13.2013.
- Laurent F., Brotons-Mas J.R., Cid E., Lopez-Pigozzi D., Valero M., Gal B., de la Prida L.M. Proximodistal structure of theta coordination in the dorsal hippocampus of epileptic rats. J Neurosci 2015; 35(11): 4760–4775, https://doi.org/10.1523/jneurosci.4297-14.2015.
- Kitchigina V.F., Butuzova M.V. Theta activity of septal neurons during different epileptic phases: the same frequency but different significance? Exp Neurol 2009; 216(2): 449–458, https://doi.org/10.1016/j.expneurol.2009.01.001.
- Rodriguez E., George N., Lachaux J.P., Martinerie J., Renault B., Varela F.J. Perception’s shadow: long-distance synchronization of human brain activity. Nature 1999; 397(6718): 430–433, https://doi.org/10.1038/17120.
- Hurtado J.M., Rubchinsky L.L., Sigvardt K.A. Statistical method for detection of phase-locking episodes in neural oscillations. J Neurophysiol 2004; 91(4): 1883–1898, https://doi.org/10.1152/jn.00853.2003.
- Canolty R.T., Edwards E., Dalal S.S., Soltani M., Nagarajan S.S., Kirsch H.E., Berger M.S., Barbaro N.M., Knight R.T. High gamma power is phase-locked to theta oscillations in human neocortex. Science 2006; 313(5793): 1626–1628, https://doi.org/10.1126/science.1128115.
- Axmacher N., Henseler M.M., Jensen O., Weinreich I., Elger C.E., Fell J. Cross-frequency coupling supports multi-item working memory in the human hippocampus. Proc Natl Acad Sci U S A 2010; 107(7): 3228–3233, https://doi.org/10.1073/pnas.0911531107.
- Montgomery S.M., Buzsaki G. Gamma oscillations dynamically couple hippocampal CA3 and CA1 regions during memory task performance. Proc Natl Acad Sci U S A 2007; 104(36): 14495–14500, https://doi.org/10.1073/pnas.0701826104.
- Tort A.B., Kramer M.A., Thorn C., Gibson D.J., Kubota Y., Graybiel A.M., Kopell N.J. Dynamic cross-frequency couplings of local field potential oscillations in rat striatum and hippocampus during performance of a T-maze task. Proc Natl Acad Sci U S A 2008; 105(51): 20517–20522, https://doi.org/10.1073/pnas.0810524105.
Nacher V., Ledberg A., Deco G., Romo R. Coherent delta-band oscillations between cortical areas correlate with decision making. Proc Natl Acad Sci U S A 2013; 110(37): 15085–15090, https://doi.org/10.1073/pnas.1314681110.- Wulff P., Ponomarenko A.A., Bartos M., Korotkova T.M., Fuchs E.C., Bähner F., Both M., Tort A.B., Kopell N.J., Wisden W., Monyer H. Hippocampal theta rhythm and its coupling with gamma oscillations require fast inhibition onto parvalbumin-positive interneurons. Proc Natl Acad Sci U S A 2009; 106(9): 3561–3566, https://doi.org/10.1073/pnas.0813176106.
- Siegel M., Warden M.R., Miller E.K. Phase-dependent neuronal coding of objects in short-term memory. Proc Natl Acad Sci U S A 2009; 106(50): 21341–21346, https://doi.org/10.1073/pnas.0908193106.
- Gregoriou G.G., Gotts S.J., Zhou H., Desimone R. High-frequency, long-range coupling between prefrontal and visual cortex during attention. Science 2009; 324(5931): 1207–1210, https://doi.org/10.1126/science.1171402.
- Palva J.M. Phase synchrony among neuronal oscillations in the human cortex. J Neurosci 2005; 25(15): 3962–3972, https://doi.org/10.1523/jneurosci.4250-04.2005.
- Palva J.M., Monto S., Kulashekhar S., Palva S. Neuronal synchrony reveals working memory networks and predicts individual memory capacity. Proc Natl Acad Sci U S A 2010; 107(16): 7580–7585, https://doi.org/10.1073/pnas.0913113107.
- Holz E.M., Glennon M., Prendergast K., Sauseng P. Theta–gamma phase synchronization during memory matching in visual working memory. NeuroImage 2010; 52(1): 326–335, https://doi.org/10.1016/j.neuroimage.2010.04.003.
- Shirvalkar P.R., Rapp P.R., Shapiro M.L. Bidirectional changes to hippocampal theta-gamma
comodulation predict memory for recent spatial episodes. Proc Natl Acad Sci U S A 2010; 107(15): 7054–7059, https://doi.org/10.1073/pnas.0911184107. - Tanninen S.E., Nouriziabari B., Morrissey M.D., Bakir R., Dayton R.D., Klein R.L., Takehara-Nishiuchi K. Entorhinal tau pathology disrupts hippocampal-prefrontal oscillatory coupling during associative learning. Neurobiol Aging 2017; 58: 151–162, https://doi.org/10.1016/j.neurobiolaging.2017.06.024.
- Friston K.J. Another neural code? NeuroImage 1997; 5(3): 213–220, https://doi.org/10.1006/nimg.1997.0260.
- Buzsáki G., Lai-Wo S.L., Vanderwolf C.H. Cellular bases of hippocampal EEG in the behaving rat. Brain Res 1983; 6(2): 139–171, https://doi.org/10.1016/0165-0173(83)90037-1.
- Buzsáki G., Buhl D.L., Harris K.D., Csicsvari J., Czéh B., Morozov A. Hippocampal network patterns of activity in the mouse. Neuroscience 2003; 116(1): 201–211, https://doi.org/10.1016/s0306-4522(02)00669-3.
- Soltesz I., Deschenes M. Low- and high-frequency membrane potential oscillations during theta activity in CA1 and CA3 pyramidal neurons of the rat hippocampus under ketamine-xylazine anesthesia. J Neurophysiol 1993; 70(1): 97–116, https://doi.org/10.1152/jn.1993.70.1.97.
- Lisman J., Idiart M. Storage of 7 +/– 2 short-term memories in oscillatory subcycles. Science 1995; 267(5203): 1512–1515, https://doi.org/10.1126/science.7878473.
- Mormann F., Fell J., Axmacher N., Weber B., Lehnertz K., Elger C.E., Fernández G. Phase/amplitude reset and theta-gamma interaction in the human medial temporal lobe during a continuous word recognition memory task. Hippocampus 2005; 15(7): 890–900, https://doi.org/10.1002/hipo.20117.
- Sirota A., Montgomery S., Fujisawa S., Isomura Y., Zugaro M., Buzsáki G. Entrainment of neocortical neurons and gamma oscillations by the hippocampal theta rhythm. Neuron 2008; 60(4): 683–697, https://doi.org/10.1016/j.neuron.2008.09.014.
- Tort A.B.L., Komorowski R., Eichenbaum H., Kopell N. Measuring phase-amplitude coupling between neuronal oscillations of different frequencies. J Neurophysiol 2010; 104(2): 1195–1210, https://doi.org/10.1152/jn.00106.2010.
- Scheffer-Teixeira R., Belchior H., Caixeta F.V., Souza B.C., Ribeiro S., Tort A.B.L. Theta phase modulates multiple layer-specific oscillations in the CA1 region. Cereb Cortex 2011; 22(10): 2404–2414, https://doi.org/10.1093/cercor/bhr319.
- Schomburg E.W., Fernández-Ruiz A., Mizuseki K., Berényi A., Anastassiou C.A., Koch C., Buzsáki G. Theta phase segregation of input-specific gamma patterns in entorhinal-hippocampal networks. Neuron 2014; 84(2): 470–485, https://doi.org/10.1016/j.neuron.2014.08.051.
- Tass P., Rosenblum M.G., Weule J., Kurths J., Pikovsky A., Volkmann J., Schnitzler A., Freund H.-J. Detection of n:m phase locking from noisy data: application to magnetoencephalography. Phys Rev Lett 1998; 81(15): 3291–3294.
- Belluscio M.A., Mizuseki K., Schmidt R., Kempter R., Buzsaki G. Cross-frequency phase-phase coupling between theta and gamma oscillations in the hippocampus. J Neurosci 2012; 32(2): 423–435, https://doi.org/10.1523/jneurosci.4122-11.2012.
- Zheng C., Zhang T. Alteration of phase–phase coupling between theta and gamma rhythms in a depression-model of rats. Cogn Neurodyn 2012; 7(2): 167–172, https://doi.org/10.1007/s11571-012-9225-x.
- Xu X., Liu C., Li Z., Zhang T. Effects of hydrogen sulfide on modulation of
theta–gamma coupling inhippocampus in vascular dementia rats. Brain Topogr 2015; 28(6): 879–894, https://doi.org/10.1007/s10548-015-0430-x. - Zheng C., Zhang T. Synaptic plasticity-related neural oscillations on
hippocampus –prefrontal cortex pathway in depression. Neuroscience 2015; 292: 170–180, https://doi.org/10.1016/j.neuroscience.2015.01.071. - Ittner A.A., Gladbach A., Bertz J., Suh L.S., Ittner L.M. p38 MAP kinase-mediated NMDA receptor-dependent suppression of hippocampal
hypersynchronicity in a mouse model of Alzheimer’s disease. Acta Neuropathol Commun 2014; 2(1): 149, https://doi.org/10.1186/s40478-014-0149-z. - Quilichini P., Sirota A., Buzsaki G. Intrinsic circuit organization and theta-gamma oscillation dynamics in the entorhinal cortex of the rat. J Neurosci 2010; 30(33): 11128–11142, https://doi.org/10.1523/jneurosci.1327-10.2010.
- Szczepanski S.M., Crone N.E., Kuperman R.A., Auguste K.I., Parvizi J., Knight R.T. Dynamic changes in phase-amplitude coupling facilitate spatial attention control in
cortex. PLoS Biology 2014; 12(8): e1001936, https://doi.org/10.1371/journal.pbio.1001936.fronto-parietal - Buzsáki G. Rhythms of the brain. Oxford University Press; 2006, https://doi.org/10.1093/acprof:oso/9780195301069.001.0001.
- Buzsáki G., Mizuseki K. The log-dynamic brain: how skewed distributions affect network operations. Nat Rev Neurosci 2014; 15(4): 264–278, https://doi.org/10.1038/nrn3687.
- Knight R.T. Neuroscience: neural networks debunk phrenology. Science 2007; 316(5831): 1578–1579, https://doi.org/10.1126/science.1144677.
- Haider B., McCormick D.A. Rapid neocortical dynamics: cellular and network mechanisms. Neuron 2009; 62(2): 171–189, https://doi.org/10.1016/j.neuron.2009.04.008.
- Voytek B., D’Esposito M., Crone N., Knight R.T. A method for event-related phase/amplitude coupling. NeuroImage 2013; 64: 416–424, https://doi.org/10.1016/j.neuroimage.2012.09.023.
- Lega B.C., Jacobs J., Kahana M. Human hippocampal theta oscillations and the formation of episodic memories. Hippocampus 2011; 22(4): 748–761, https://doi.org/10.1002/hipo.20937.
- Newman E.L., Gillet S.N., Climer J.R., Hasselmo M.E. Cholinergic blockade reduces theta–gamma
phase amplitude coupling and speed modulation of theta frequency consistent with behavioral effects on encoding. J Neurosci 2013; 33(50): 19635–19646, https://doi.org/10.1523/jneurosci.2586-13.2013. - Schack B., Weiss S. Quantification of phase synchronization phenomena and their importance for verbal memory processes. Biol Cybern 2005; 92(4): 275–287, https://doi.org/10.1007/s00422-005-0555-1.
- Fell J., Axmacher N. The role of phase synchronization in memory processes. Nat Rev Neurosci 2011; 12(2): 105–118, https://doi.org/10.1038/nrn2979.
- Scheffer-Teixeira R., Tort A.B. On cross-frequency phase-phase coupling between theta and gamma oscillations in the hippocampus. Elife 2016; 5: e20515, https://doi.org/10.7554/elife.20515.
- Markram H. Regulation of synaptic efficacy by coincidence of postsynaptic APs and EPSPs. Science 1997; 275(5297): 213–215, https://doi.org/10.1126/science.275.5297.213.
- Morimoto K., Fahnestock M., Racine R.J. Kindling and status epilepticus models of epilepsy: rewiring the brain. Prog Neurobiol 2004; 73(1): 1–60, https://doi.org/10.1016/j.pneurobio.2004.03.009.
- Dupont S., Van de Moortele P.F., Samson S., Hasboun D., Poline J.B., Adam C., Lehéricy S., Le Bihan D., Samson Y., Baulac M. Episodic memory in left temporal lobe epilepsy: a functional MRI study. Brain 2000; 123(Pt 8): 1722–1732, https://doi.org/10.1093/brain/123.8.1722.
- Helmstaedter C. Effects of chronic epilepsy on declarative memory systems. Prog Brain Res 2002; 135: 439–453, https://doi.org/10.1016/s0079-6123(02)35041-6.
- Burgess N., Maguire E.A., O’Keefe J. The human hippocampus and spatial and episodic memory. Neuron 2002; 35(4): 625–641, https://doi.org/10.1016/s0896-6273(02)00830-9.
- Tulving E. Episodic memory: from mind to brain. Annu Rev Psychol 2002; 53(1): 1–25, https://doi.org/10.1146/annurev.psych.53.100901.135114.
- Lega B., Dionisio S., Bingaman W., Najm I., Gonzalez-Martinez J. The gamma band effect for episodic memory encoding is absent in epileptogenic hippocampi. Clin Neurophysiol 2015; 126(5): 866–872, https://doi.org/10.1016/j.clinph.2014.07.035.
- O’Keefe J., Nadel L. The hippocampus as a cognitive map. Oxford, UK: Oxford University Press; 1978.
- Komorowski R.W., Manns J.R., Eichenbaum H. Robust conjunctive item-place coding by hippocampal neurons parallels learning what happens where. J Neurosci 2009; 29(31): 9918–9929, https://doi.org/10.1523/jneurosci.1378-09.2009.
- Mankin E.A., Sparks F.T., Slayyeh B., Sutherland R.J., Leutgeb S., Leutgeb J.K. Neuronal code for
extended time in the hippocampus. Proc Natl Acad Sci U S A 2012; 109(47): 19462–19467, https://doi.org/10.1073/pnas.1214107109. - Kraus B.J., Robinson R.J., White J.A., Eichenbaum H., Hasselmo M.E. Hippocampal “time cells”: time versus path integration. Neuron 2013; 78(6): 1090–1101, https://doi.org/10.1016/j.neuron.2013.04.015.
- Kitamura T., Pignatelli M., Suh J., Kohara K., Yoshiki A., Abe K., Tonegawa S. Island cells control temporal association memory. Science 2014; 343(6173): 896–901, https://doi.org/10.1126/science.1244634.
- Frank L.M., Brown E.N., Wilson M. Trajectory encoding in the hippocampus and entorhinal cortex. Neuron 2000; 27(1): 169–178, https://doi.org/10.1016/s0896-6273(00)00018-0.
- Wood E.R., Dudchenko P.A., Robitsek R.J., Eichenbaum H. Hippocampal neurons encode information about different types of memory episodes occurring in the same location. Neuron 2000; 27(3): 623–633, https://doi.org/10.1016/s0896-6273(00)00071-4.
- Huxter J.R., Senior T.J., Allen K., Csicsvari J. Theta phase–specific codes for
two-dimensional position, trajectory and heading in the hippocampus. Nat Neurosci 2008; 11(5): 587–594, https://doi.org/10.1038/nn.2106. - Mizuseki K., Sirota A., Pastalkova E., Buzsáki G. Theta oscillations provide temporal windows for local circuit computation in the entorhinal-hippocampal loop. Neuron 2009; 64(2): 267–280, https://doi.org/10.1016/j.neuron.2009.08.037.
- Easton A., Douchamps V., Eacott M., Lever C. A specific role for septohippocampal acetylcholine in memory? Neuropsychologia 2012; 50(13): 3156–3168, https://doi.org/10.1016/j.neuropsychologia.2012.07.022.
- Buzsáki G., Moser E.I. Memory, navigation and theta rhythm in the hippocampal-entorhinal system. Nat Neurosci 2013; 16(2): 130–138, https://doi.org/10.1038/nn.3304.
- Siapas A.G., Lubenov E.V., Wilson M.A. Prefrontal phase locking to hippocampal theta oscillations. Neuron 2005; 46(1): 141–151, https://doi.org/10.1016/j.neuron.2005.02.028.
- Jones M.W., Wilson M.A. Theta rhythms coordinate hippocampal–prefrontal interactions in a spatial memory task. PLoS Biology 2005; 3(12): e402, https://doi.org/10.1371/journal.pbio.0030402.
- Broggini A.C.S., Esteves I.M., Romcy-Pereira R.N., Leite J.P., Leão R.N. Pre-ictal increase in theta synchrony between the hippocampus and prefrontal cortex in a rat model of temporal lobe epilepsy. Exp Neurol 2016; 279: 232–242, https://doi.org/10.1016/j.expneurol.2016.03.007.
- Arabadzisz D., Antal K., Parpan F., Emri Z., Fritschy J.-M. Epileptogenesis and chronic seizures in a mouse model of temporal lobe epilepsy are associated with distinct EEG patterns and selective neurochemical alterations in the contralateral hippocampus. Exp Neurol 2005; 194(1): 76–90, https://doi.org/10.1016/j.expneurol.2005.01.029.
- Colom L.V., García-Hernández A., Castañeda M.T., Perez-Cordova M.G., Garrido-Sanabria E.R. Septo-hippocampal networks in chronically epileptic rats: potential antiepileptic effects of theta rhythm generation. J Neurophysiol 2006; 95(6): 3645–3653, https://doi.org/10.1152/jn.00040.2006.
- Dugladze T., Vida I., Tort A.B., Gross A., Otahal J., Heinemann U., Kopell N.J., Gloveli T. Impaired hippocampal rhythmogenesis in a mouse model of mesial temporal lobe epilepsy. Proc Natl Acad Sci U S A 2007; 104(44): 17530–17535, https://doi.org/10.1073/pnas.0708301104.
- Marcelin B., Chauvière L., Becker A., Migliore M., Esclapez M., Bernard C. h channel-dependent deficit of theta oscillation resonance and phase shift in temporal lobe epilepsy. Neurobiol Dis 2009; 33(3): 436–447, https://doi.org/10.1016/j.nbd.2008.11.019.
- García-Hernández A., Bland B.H., Facelli J.C., Colom L.V. Septo-hippocampal networks in chronic epilepsy. Exp Neurol 2010; 222(1): 86–92, https://doi.org/10.1016/j.expneurol.2009.12.010.
- Selkoe D.J. Toward a comprehensive theory for Alzheimer’s disease. hypothesis: Alzheimer’s disease is caused by the cerebral accumulation and cytotoxicity of amyloid β-protein. Ann N Y Acad Sci 2006; 924(1): 17–25, https://doi.org/10.1111/j.1749-6632.2000.tb05554.x.
- Palop J.J., Mucke L. Amyloid-beta-induced neuronal dysfunction in Alzheimer’s disease: from synapses toward neural networks. Nat Neurosci 2010; 13(7): 812–818, https://doi.org/10.1038/nn.2583.
- Francis B.M., Kim J., Barakat M.E., Fraenkl S., Yücel Y.H., Peng S., Michalski B., Fahnestock M., McLaurin J., Mount H.T. Object recognition memory and BDNF expression are reduced in young TgCRND8 mice. Neurobiol Aging 2012; 33(3): 555–563, https://doi.org/10.1016/j.neurobiolaging.2010.04.003.
- Kametani F., Hasegawa M. Reconsideration of amyloid hypothesis and tau hypothesis in Alzheimer’s disease. Front Neurosci 2018; 12: 25–36, https://doi.org/10.3389/fnins.2018.00025.
- Gureviciene I., Gurevicius K., Mugantseva E., Kislin M., Khiroug L., Tanila H. Amyloid plaques show
binding capacity of exogenous injected amyloid-β. J Alzheimers Dis 2016; 55(1): 147–157, https://doi.org/10.3233/jad-160453. - Didic M., Barbeau E.J., Felician O., Tramoni E., Guedj E., Poncet M., Ceccaldi M. Which memory system is impaired first in Alzheimer’s disease? J Alzheimers Dis 2011; 27(1): 11–22, https://doi.org/10.3233/jad-2011-110557.
- Lithfous S., Dufour A., Després O. Spatial navigation in normal aging and the prodromal stage of Alzheimer’s disease: insights from imaging and behavioral studies. Ageing Research Reviews 2013; 12(1): 201–213, https://doi.org/10.1016/j.arr.2012.04.007.
- Adler G., Brassen S., Jajcevic A. EEG coherence in Alzheimer’s dementia. J Neural Transm 2003; 110(9): 1051–1058, https://doi.org/10.1007/s00702-003-0024-8.
- Herrmann C., Demiralp T. Human EEG gamma oscillations in neuropsychiatric disorders. Clin Neurophysiol 2005; 116(12): 2719–2733, https://doi.org/10.1016/j.clinph.2005.07.007.
- van der Hiele K., Vein A.A., van der Welle A., van der Grond J., Westendorp R.G., Bollen E.L., van Buchem M.A., van Dijk J.G., Middelkoop H.A. EEG and MRI correlates of mild cognitive impairment and Alzheimer’s disease. Neurobiol Aging 2007; 28(9): 1322–1329, https://doi.org/10.1016/j.neurobiolaging.2006.06.006.
- Czigler B., Csikós D., Hidasi Z., Anna Gaál Z., Csibri E., Kiss E., Salacz P., Molnár M. Quantitative EEG in early Alzheimer’s disease patients — power spectrum and complexity features. Int J Psychophysiol 2008; 68(1): 75–80, https://doi.org/10.1016/j.ijpsycho.2007.11.002.
- Moretti D.V., Pievani M., Geroldi C., Binetti G., Zanetti O., Rossini P.M., Frisoni G.B. EEG markers discriminate among
different subgroup of patients with mild cognitive impairment. Am J Alzheimers Dis Other Demen 2010; 25(1): 58–73, https://doi.org/10.1177/1533317508329814. - Caravaglios G., Castro G., Costanzo E., Di Maria G., Mancuso D., Muscoso E.G. Theta power responses in mild Alzheimer’s disease during an auditory oddball paradigm: lack of theta enhancement during stimulus processing. J Neural Transm 2010; 117(10): 1195–1208, https://doi.org/10.1007/s00702-010-0488-2.
- Wang J., Fang Y., Wang X., Yang H., Yu X., Wang H. Enhanced gamma activity and cross-frequency interaction of resting-state electroencephalographic oscillations in patients with Alzheimer’s disease. Front Aging Neurosci 2017; 9: 243, https://doi.org/10.3389/fnagi.2017.00243.
- Schneider F., Baldauf K., Wetzel W., Reymann K.G. Behavioral and EEG changes in male 5xFAD mice. Physiol Behav 2014; 135: 25–33, https://doi.org/10.1016/j.physbeh.2014.05.041.
- Goodman M.S., Kumar S., Zomorrodi R., Ghazala Z., Cheam A.S.M., Barr M.S., Daskalakis Z.J., Blumberger D.M., Fischer C., Flint A., Mah L., Herrmann N., Bowie C.R., Mulsant B.H., Rajji T.K. Theta-gamma coupling and working memory in Alzheimer’s dementia and mild cognitive impairment. Front Aging Neurosci 2018; 10: 101, https://doi.org/10.3389/fnagi.2018.00101.
- Goutagny R., Gu N., Cavanagh C., Jackson J., Chabot J.G., Quirion R., Krantic S., Williams S. Alterations in hippocampal network oscillations and theta-gamma coupling arise before Aβ overproduction in a mouse model of Alzheimer’s disease. Eur J Neurosci 2013; 37(12): 1896–1902, https://doi.org/10.1111/ejn.12233.
- Scott L., Feng J., Kiss T., Needle E., Atchison K., Kawabe T.T., Milici A.J., Hajós-Korcsok E., Riddell D., Hajós M. Age-dependent disruption in hippocampal theta oscillation in amyloid-β overproducing transgenic mice. Neurobiol Aging 2012; 33(7): 1481.e13–1481.e23, https://doi.org/10.1016/j.neurobiolaging.2011.12.010.
- Seabrook G.R., Smith D.W., Bowery B.J., Easter A., Reynolds T., Fitzjohn S.M., Morton R.A., Zheng H., Dawson G.R., Sirinathsinghji D.J., Davies C.H., Collingridge G.L., Hill R.G. Mechanisms contributing to the deficits in hippocampal synaptic plasticity in mice lacking amyloid precursor protein. Neuropharmacology 1999; 38(3): 349–359, https://doi.org/10.1016/s0028-3908(98)00204-4.
- Senechal Y., Kelly P.H., Dev K.K. Amyloid precursor protein knockout mice show age-dependent deficits in passive avoidance learning. Behav Brain Res 2008; 186(1): 126–132, https://doi.org/10.1016/j.bbr.2007.08.003.
- Zhang X., Zhong W., Brankačk J., Weyer S.W., Müller U.C., Tort A.B., Draguhn A. Impaired theta-gamma coupling in APP-deficient mice. Sci Rep 2016; 6: 21948, https://doi.org/10.1038/srep21948.
- Armon C., Peterson G.W., Liwnicz B.H. Alzheimer’s disease underlies some cases of complex partial status epilepticus. J Clin Neurophysiol 2000; 17(5): 511–518, https://doi.org/10.1097/00004691-200009000-00011.
- Amatniek J.C., Hauser W.A., DelCastillo-Castaneda C., Jacobs D.M., Marder K., Bell K., Albert M., Brandt J., Stern Y. Incidence and predictors of seizures in patients with Alzheimer’s disease. Epilepsia 2006; 47(5): 867–872, https://doi.org/10.1111/j.1528-1167.2006.00554.x.
- Braak H., Braak E. Evolution of the neuropathology of Alzheimer’s disease. Acta Neurol Scand Suppl 1996; 165: 3–12, https://doi.org/10.1111/j.1600-0404.1996.tb05866.x.
- Born H.A., Kim J.Y., Savjani R.R., Das P., Dabaghian Y.A., Guo Q., Yoo J.W., Schuler D.R., Cirrito J.R., Zheng H., Golde T.E., Noebels J.L., Jankowsky J.L. Genetic suppression of transgenic APP rescues hypersynchronous network activity in a mouse model of
Alzeimer’s disease. J Neurosci 2014; 34(11): 3826–3840, https://doi.org/10.1523/jneurosci.5171-13.2014. - Chan J., Jones N.C., Bush A.I., O’Brien T.J., Kwan P. A mouse model of Alzheimer’s disease displays increased susceptibility to kindling and seizure-associated death. Epilepsia 2015; 56(6): e73–e77, https://doi.org/10.1111/epi.12993.
- Bird S.B., Orr P.G., Mazzola J.L., Brush D.E., Boyer E.W. Levofloxacin-related seizure activity in a patient with Alzheimer’s disease: assessment of potential risk factors. J Clin Psychopharmacol 2005; 25(3): 287–288, https://doi.org/10.1097/01.jcp.0000162811.15066.8e.
Bernardi S., Scaldaferri N., Vanacore N., Trebbastoni A., Francia A., D’Amico A., Prencipe M. Seizures in Alzheimer’s disease: a retrospective study of a cohort of outpatients. Epileptic Disord 2010; 12(1): 16–21.- Palop J.J., Mucke L. Network abnormalities and interneuron dysfunction in Alzheimer disease. Nat Rev Neurosci 2016; 17(12): 777–792, https://doi.org/10.1038/nrn.2016.141.
- Bezzina C., Verret L., Juan C.,
Remaud J., Halley H., Rampon C., Dahan L. Early onset of hypersynchronous network activity and expression of a marker of chronic seizures in the Tg2576 mouse model of Alzheimer’s disease. PLoS One 2015; 10(3): e0119910, https://doi.org/10.1371/journal.pone.0119910. - Palop J.J., Chin J., Roberson E.D., Wang J.,
Thwin M.T., Bien-Ly N., Yoo J., Ho K.O., Yu G.Q., Kreitzer A., Finkbeiner S., Noebels J.L., Mucke L. Aberrant excitatory neuronal activity and compensatory remodeling of inhibitory hippocampal circuits in mouse models of Neuron 2007; 55(5): 697–711, https://doi.org/10.1016/j.neuron.2007.07.025. - Bero A.W., Yan P., Roh J.H., Cirrito J.R., Stewart F.R., Raichle M.E., Lee J.M., Holtzman D.M. Neuronal activity regulates the regional vulnerability to amyloid-β deposition. Nat Neurosci 2011; 14(6): 750–756, https://doi.org/10.1038/nn.2801.
- Cirrito J.R., Kang J.-E., Lee J., Stewart F.R., Verges D.K., Silverio L.M., Bu G., Mennerick S., Holtzman D.M. Endocytosis is required for synaptic activity-dependent release of amyloid-β in vivo. Neuron 2008; 58(1): 42–51, https://doi.org/10.1016/j.neuron.2008.02.003.
Gallassi R. Epileptic amnesic syndrome: an update and further considerations. Epilepsia 2006; 47(s2): 103–105, https://doi.org/10.1111/j.1528-1167.2006.00704.x.- Vossel K.A., Beagle A.J., Rabinovici G.D., Shu H., Lee S.E., Naasan G., Hegde M., Cornes S.B., Henry M.L., Nelson A.B., Seeley W.W., Geschwind M.D., Gorno-Tempini M.L., Shih T., Kirsch H.E., Garcia P.A., Miller B.L., Mucke L. Seizures and epileptiform activity in the early stages of Alzheimer disease. JAMA Neurol 2013; 70(9): 1158, https://doi.org/10.1001/jamaneurol.2013.136.
- Cretin B., Sellal F., Philippi N., Bousiges O., Di Bitonto L., Martin-Hunyadi C., Blanc F. Epileptic prodromal Alzheimer’s disease, a retrospective study of 13 new cases: expanding the spectrum of Alzheimer’s disease to an epileptic variant? J Alzheimers Dis 2016; 52(3): 1125–1133, https://doi.org/10.3233/jad-150096.
- Chin J., Scharfman H.E. Shared cognitive and behavioral impairments in epilepsy and Alzheimer’s disease and potential underlying mechanisms. Epilepsy Behav 2013; 26(3): 343–351, https://doi.org/10.1016/j.yebeh.2012.11.040.
- Eichler S.A. E-I balance and human diseases — from molecules to networking. Front Mol Neurosci 2008; 1: 2, https://doi.org/10.3389/neuro.02.002.2008.
- Noebels J. A perfect storm: converging paths of epilepsy and Alzheimer’s dementia intersect in the hippocampal formation. Epilepsia 2011; 52: 39–46, https://doi.org/10.1111/j.1528-1167.2010.02909.x.
- Saito Y., Inoue T., Zhu G., Kimura N., Okada M., Nishimura M., Kimura N., Murayama S., Kaneko S., Shigemoto R., Imoto K., Suzuki T. Hyperpolarization-activated cyclic
nucleotide gated channels: a potential molecular link between epileptic seizures and Aβ generation in Alzheimer’s disease. Mol Neurodegener 2012; 7: 50, https://doi.org/10.1186/1750-1326-7-50. - Varga E., Juhász G., Bozsó Z., Penke B., Fülöp L., Szegedi V. Abeta(1-42) enhances neuronal excitability in the CA1 via NR2B subunit-containing NMDA receptors. Neural Plast 2014; 2014: 1–12, https://doi.org/10.1155/2014/584314.
- Irizarry M.C., Jin S., He F., Emond J.A., Raman R., Thomas R.G., Sano M., Quinn J.F., Tariot P.N., Galasko D.R., Ishihara L.S., Weil J.G., Aisen P.S. Incidence of new-onset seizures in mild to moderate Alzheimer disease. Arch Neurol 2012; 69(3): 368, https://doi.org/10.1001/archneurol.2011.830.
- Scarmeas N., Honig L.S., Choi H., Cantero J., Brandt J., Blacker D., Albert M., Amatniek J.C., Marder K., Bell K., Hauser W.A., Stern Y. Seizures in Alzheimer disease: who, when, and how common? Arch Neurol 2009; 66(8): 992–997, https://doi.org/10.1001/archneurol.2009.130.
- Sarkis R.A., Willment K.C., Gale S.A., Dworetzky B.A. Recurrent epileptic auras as a presenting symptom of Alzheimer’s disease. Front Neurol 2017; 8: 360, https://doi.org/10.3389/fneur.2017.00360.
- Minkeviciene R., Rheims S., Dobszay M.B., Zilberter M., Hartikainen J., Fülöp L., Penke B., Zilberter Y., Harkany T., Pitkänen A., Tanila H. Amyloid beta-induced neuronal hyperexcitability triggers progressive epilepsy. J Neurosci 2009; 29(11): 3453–3462, https://doi.org/10.1523/jneurosci.5215-08.2009.
- Vogt D.L., Thomas D., Galvan V., Bredesen D.E., Lamb B.T., Pimplikar S.W. Abnormal neuronal networks and seizure susceptibility in mice overexpressing the APP intracellular domain. Neurobiol Aging 2011; 32(9): 1725–1729, https://doi.org/10.1016/j.neurobiolaging.2009.09.002.
- Yener G.G., Başar E. Biomarkers in Alzheimer’s disease with a special emphasis on event-related oscillatory responses. Suppl Clin Neurophysiol 2013: 62: 237–273, https://doi.org/10.1016/b978-0-7020-5307-8.00020-x.
- Wang B., Meng L. Functional brain network alterations in epilepsy: a magnetoencephalography study. Epilepsy Res 2016; 126: 62–69, https://doi.org/10.1016/j.eplepsyres.2016.06.014.