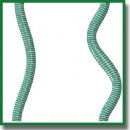
Loss of Stability in a Small-Caliber Vascular Graft
The aim of the study is to develop and test a numerical method for assessing a loss of stability in a polymer vascular graft in response to a longitudinal dynamic high-speed load.
Materials and Methods. A small-caliber vascular graft made by electrospinning from the composite mixture of polyhydroxybutyrate/valerate and polycaprolactone polymers was studied. Numerical methods (finite element method) and prototype test (uniaxial tension) were used to assess the loading speeds and the resulting loss of stability, which are critical for small-caliber vascular grafts.
Results. The mechanical testing of a vascular graft prototype allowed us to determine its response to quasi-static load. We also showed that the high-speed longitudinal dynamic loads significantly reduced the load-carrying capacity of the structure. This and related effects are suggested to be potential causes of the vessel lumen collapse resulted from irreversible loss of stability.
Conclusion. The impact of a dynamic load significantly weakens the vascular graft and affects its elastic and deformation characteristics, leading to the premature critical deformation and eventual occlusion. Therefore, the quality of such products can be improved by a preliminary prognostic work and reinforcement of their construction.
- Chen H., Kassab G.S. Microstructure-based constitutive model of coronary artery with active smooth muscle contraction. Sci Rep 2017; 7(1): 9339, https://doi.org/10.1038/s41598-017-08748-7.
- Stankiewicz J.M., Robertson S.W., Ritchie R.O. Fatigue-crack growth properties of thin-walled superelastic austenitic Nitinol tube for endovascular stents. J Biomed Mater Res A 2007; 81(3): 685–691, https://doi.org/10.1002/jbm.a.31100.
- Bernardini A., Larrabide I., Morales H.G., Pennati G., Petrini L., Cito S., Frangi A.F. Influence of different computational approaches for stent deployment on cerebral aneurysm haemodynamics. Interface Focus 2011; 1(3): 338–348, https://doi.org/10.1098/rsfs.2011.0004.
- Lee A.Y., Han H.-C. A nonlinear thin-wall model for vein buckling. Cardiovasc Eng 2010; 1(4): 282–289, https://doi.org/10.1007/s13239-010-0024-4.
- Suzuki T., Takao H., Fujimura S., Dahmani C., Ishibashi T., Mamori H., Fukushima N., Yamamoto M., Murayama Y. Selection of helical braided flow diverter stents based on hemodynamic performance and mechanical properties. J Neurointerv Surg 2017; 9(10): 999–1005, https://doi.org/10.1136/neurintsurg-2016-012561.
- Li H., Gu J., Wang M., Zhao D., Li Z., Qiao A., Zhu B. Multi-objective optimization of coronary stent using Kriging surrogate model. Biomed Eng Online 2016; 15(Suppl 2): 148, https://doi.org/10.1186/s12938-016-0268-9.
- Smouse H.B., Nikanorov A., LaFlash D. Biomechanical forces in the femoropopliteal arterial segment. What happens during extremity movement and what is the effect on stenting? Endovasc Today 2005; 4: 60–66.
- Li H., Leow W.K., Chiu I.S. Modeling torsion of blood vessels in surgical simulation and planning. Stud Health Technol Inform 2009; 142: 153–158.
- Selvaggi G., Anicic S., Formaggia L. Mathematical explanation of the buckling of the vessels after twisting of the microanastomosis. Microsurgery 2006; 26(7): 524–528, https://doi.org/10.1002/micr.20281.
- Garcia J.R., Lamm S.D., Han H.-C. Twist buckling behavior of arteries. Biomech Model Mechanobiol 2013; 12(5): 915–927, https://doi.org/10.1007/s10237-012-0453-0.
- Ding Z., Friedman M.H. Dynamics of human coronary arterial motion and its potential role in coronary atherogenesis. J Biomech Eng 2000; 122(5): 488–492, https://doi.org/10.1115/1.1289989.
- Antonova L.V., Sevostyanova V.V., Velikanova E.A., Matveeva V.G., Glushkova T.V., Mironov A.V., Krivkina E.O., Barbarash L.S. Biofunctionalization of biodegradable small-diameter vascular grafts VEGF, BFGF, and SDF-1A: experimental results. Vestnik transplantologii i iskusstvennykh organov 2017; 19(5): 197.
- Belytschko T., Bindeman L.P. Assumed strain stabilization of the eight node hexahedral element. Comput Methods Appl Mech Eng 1993; 105: 225–260, https://doi.org/10.1016/0045-7825(93)90124-g.
- Nushtaev D.V., Zhavoronok S.I., Klyshnikov K.Y., Ovcharenko E.A. Combined numerical and experimental investigation of the deformed state and buckling of the meshed cylindrical shell subjected to the axial compression. Trudy MAI 2015; 82: 9.
- Glushkova T.V., Sevostyanova V.V., Antonova L.V., Klyshnikov K.Yu., Ovcharenko E.A., Sergeeva E.A., Vasyukov G.Yu., Seifalian A.M., Barbarash L.S. Biomechanical remodeling of biodegradable small-diameter vascular grafts in situ. Vestnik transplantologii i iskusstvennykh organov 2016; 18(2): 99–109.
- Belytschko T., Lin J.I., Tsay C.S. Explicit algorithms for the nonlinear dynamics of shells. Comput Methods Appl Mech Eng 1984; 42(2): 251–276, https://doi.org/10.1016/0045-7825(84)90026-4.
- Xu J., Yang J., Huang N., Uhl C., Zhou Y., Liu Y. Mechanical response of cardiovascular stents under vascular dynamic bending. Biomed Eng Online 2016; 15(1): 21, https://doi.org/10.1186/s12938-016-0135-8.
- Spranger K., Capelli C., Bosi G.M., Schievano S., Ventikos Y. Comparison and calibration of a real-time virtual stenting algorithm using finite element analysis and genetic algorithms. Comput Methods Appl Mech Eng 2015; 293: 462–480, https://doi.org/10.1016/j.cma.2015.03.022.
- Fu W., Xia Q. Interaction between flow diverter and parent artery of intracranial aneurysm: a computational study. Appl Bionics Biomech 2017; 2017: 3751202, https://doi.org/10.1155/2017/3751202.
- Antonova L.V., Krivkina E.O., Sergeeva E.A., Sevostyanova V.V., Burago A.Yu., Burkov N.N., Sharifulin R.F., Velikanova E.A., Kudryavtseva Yu.A., Barbarash O.L., Barbarash L.S. Tissue engineered scaffold modified by bioactive molecules for directed tissue regeneration. Complex Issues of Cardiovascular Diseases 2016; 5(1): 18–25, https://doi.org/10.17802/2306-1278-2016-1-18-25.