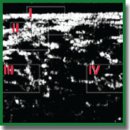
Improving the Transverse Resolution of Optical Coherence Tomography with a Finite Impulse Response Filter and a Series of Numerically Refocused Images
Among the numerous methods for improving the informative value of the optical coherence tomography (OCT), a special place is taken by the methods for increasing the spatial resolution of the resulting images. Increasing the resolution allows one to identify more clinically significant structures in OCT images and thus improve the diagnostic value of OCT. Since the transverse resolution of OCT images is determined by the physical principles different from those for the longitudinal resolution, the ways of their improvement are also different.
The aim of the study is to develop a method for increasing the transverse resolution of OCT by using a finite impulse response (FIR) filter for numerical refocusing and by combining the numerically refocused images.
Results. We have developed a FIR filter able to transfer the focal plane of an OCT image using the data from 17 consecutive measurements of the field scattered by the object. In addition, we propose an original method for automatic synthesis of the final OCT image with an improved transverse resolution over the entire object depth from a series of images having different focal plane positions. This will enable the OCT instrument to produce a sharply focused beam for the scanning and thus obtain images with an improved transverse resolution in the focal plane, restore the required resolution in the out-of-focus areas using numerical transfer of the focal plane, and also synthesize the final OCT image with an improved transverse resolution. The method has been tested with model objects using an OCT device operating at a central wavelength of 1 μm with the 60 nm range and a beam focused onto a spot with a diameter of 5 μm.
Conclusion. The proposed method of numerically increasing the transverse resolution of OCT images allows one to obtain images with an improved transverse resolution using only a small number of OCT measurements; in the future, it will allow for creating an OCT device that produces high-resolution images in real time.
- Adhi M., Duker J.S. Optical coherence tomography — current and future applications. Curr Opin Ophthalmol 2013; 24(3): 213–221, https://doi.org/10.1097/icu.0b013e32835f8bf8.
- Vakoc B.J., Fukumura D., Jain R.K., Bouma B.E. Cancer imaging by optical coherence tomography: preclinical progress and clinical potential. Nat Rev Cancer 2012; 12(5): 363–368, https://doi.org/10.1038/nrc3235.
- Gelikonov V.M., Gelikonov G.V. New approach to cross-polarized optical coherence tomography based on orthogonal arbitrarily polarized modes. Laser Phys Lett 2006; 3(9): 445–451, https://doi.org/10.1002/lapl.200610030.
- Shakhova N.M., Gelikonov V.M., Kamensky V.A., Kuranov R.V., Turchin I.V. Clinical aspects of the endoscopic optical coherence tomography and the ways for improving its diagnostic value. Laser Phys 2002; 12(4): 617–626.
- Sudheendran N., Syed S.H., Dickinson M.E., Larina I.V., Larin K.V. Speckle variance OCT imaging of the vasculature in live mammalian embryos. Laser Phys Lett 2011; 8(3): 247–252, https://doi.org/10.1002/lapl.201010120.
- Wang S., Larin K.V., Li J., Vantipalli S., Manapuram R.K., Aglyamov S., Emelianov S., Twa M.D. A focused air-pulse system for optical-coherence-tomography-based measurements of tissue elasticity. Laser Phys Lett 2013; 10(7): 075605, https://doi.org/10.1088/1612-2011/10/7/075605.
- Gambichler T., Schmid-Wendtner M.H., Plura I., Kampilafkos P., Stücker M., Berking C., Maier T. A multicentre pilot study investigating high-definition optical coherence tomography in the differentiation of cutaneous melanoma and melanocytic naevi. J Eur Acad Dermatol Venereol 2014; 29(3): 537–541, https://doi.org/10.1111/jdv.12621.
- Erickson-Bhatt S.J., Nolan R.M., Shemonski N.D., Adie S.G., Putney J., Darga D., McCormick D.T., Cittadine A.J., Zysk A.M., Marjanovic M., Chaney E.J., Monroy G.L., South F.A., Cradock K.A., Liu Z.G., Sundaram M., Ray P.S., Boppart S.A. Real-time imaging of the resection bed using a handheld probe to reduce incidence of microscopic positive margins in cancer surgery. Cancer Res 2015; 75(18): 3706–3712, https://doi.org/10.1158/0008-5472.can-15-0464.
- Schulz-Hildebrandt H., Pieper M., Stehmar C., Ahrens M., Idel C., Wollenberg B., König P., Hüttmann G. Novel endoscope with increased depth of field for imaging human nasal tissue by microscopic optical coherence tomography. Biomed Opt Express 2018; 9(2): 636, https://doi.org/10.1364/boe.9.000636.
- Verma Y., Divakar Rao K., Mohanty S.K., Gupta P.K. Optical coherence tomography using a tapered single mode fiber tip. Laser Phys Lett 2007; 4(9): 686–689, https://doi.org/10.1002/lapl.200710045.
- Villiger M., Pache C., Lasser T. Dark-field optical coherence microscopy. Opt Lett 2010; 35(20): 3489, https://doi.org/10.1364/ol.35.003489.
- Yin B., Chu K.K., Liang C.-P., Singh K., Reddy R., Tearney G.J. μOCT imaging using depth of focus extension by self-imaging wavefront division in a common-path fiber optic probe. Opt Express 2016; 24(5): 5555, https://doi.org/10.1364/oe.24.005555.
- Aguirre A.D., Zhou C., Lee H.-C., Ahsen O.O., Fujimoto J.G. Optical coherence microscopy. In: Optical coherence tomography. Springer International Publishing; 2015; p. 865–911, https://doi.org/10.1007/978-3-319-06419-2_29.
- Dubois A., Vabre L., Boccara A.-C., Beaurepaire E. High-resolution full-field optical coherence tomography with a Linnik microscope. Appl Opt 2002; 41(4): 805, https://doi.org/10.1364/ao.41.000805.
- Qi B., Phillip Himmer A., Maggie Gordon L., Victor Yang X.D., David Dickensheets L., Alex Vitkin I. Dynamic focus control in high-speed optical coherence tomography based on a microelectromechanical mirror. Opt Commun 2004; 232(1–6): 123–128, https://doi.org/10.1016/j.optcom.2004.01.015.
- Ralston T.S., Adie S.G., Marks D.L., Boppart S.A., Carney P.S. Cross-validation of interferometric synthetic aperture microscopy and optical coherence tomography. Opt Lett 2010; 35(10): 1683, с.
- Ralston T.S., Marks D.L., Scott Carney P., Boppart S.A. Interferometric synthetic aperture microscopy. Nature Physics 2007; 3(2): 129–134, https://doi.org/10.1038/nphys514.
- Yasuno Y., Sugisaka J., Sando Y., Nakamura Y., Makita S., Itoh M., Yatagai T. Non-iterative numerical method for laterally superresolving Fourier domain optical coherence tomography. Opt Express 2006; 14(3): 1006, https://doi.org/10.1364/oe.14.001006.
- Yu L., Rao B., Zhang J., Su J., Wang Q., Guo S., Chen Z. Improved lateral resolution in optical coherence tomography by digital focusing using two-dimensional numerical diffraction method. Opt Express 2007; 15(12): 7634, https://doi.org/10.1364/oe.15.007634.
- Moiseev A.A., Gelikonov G.V., Terpelov D.A., Shilyagin P.A., Gelikonov V.M. Digital refocusing for transverse resolution improvement in optical coherence tomography. Laser Phys Lett 2012; 9(11): 826–832, https://doi.org/10.7452/lapl.201210102.
- Moiseev A.A., Gelikonov G.V., Terpelov D.A., Shilyagin P.A., Gelikonov V.M. Noniterative method of reconstruction optical coherence tomography images with improved lateral resolution in semitransparent media. Laser Phys Lett 2013; 10(12): 125601, https://doi.org/10.1088/1612-2011/10/12/125601.
- Moiseev A., Ksenofontov S., Sirotkina M., Kiseleva E., Gorozhantseva M., Shakhova N., Matveev L., Zaitsev V., Matveyev A., Zagaynova E., Gelikonov V., Gladkova N., Vitkin A., Gelikonov G. Optical coherence tomography-based angiography device with real-time angiography B-scans visualization and hand-held probe for everyday clinical use. J Biophotonics 2018; 11(10): e201700292, https://doi.org/10.1002/jbio.201700292.
- Fonseca E.S.R., Fiadeiro P.T., Pereira M., Pinheiro A. Comparative analysis of autofocus functions in digital in-line phase-shifting holography. Appl Opt 2016; 55(27): 7663, https://doi.org/10.1364/ao.55.007663.