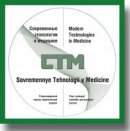
Bile Acids as a New Type of Steroid Hormones Regulating Nonspecific Energy Expenditure of the Body (Review)
The review is devoted to the systematization, classification, and generalization of the results of modern scientific research on the role of bile acids as a new class of steroid hormones. The paper presents the evidence for bile acid participation in the regulation of the body energy metabolism, body weight control, as well as the pathogenesis of obesity, diabetes mellitus, insulin resistance, and cardiovascular diseases.
Particular attention is paid to the role of bile acids in the control of nonspecific energy expenditure of the body. The applied aspects of using the novel data about the membrane and intracellular receptors responsible for the development of hormonal regulatory effects of bile acids are analyzed. According to the authors, the modern data on the role of bile acids in the regulation of body functions allow a deeper understanding of the pathogenesis of body weight disorders and associated cardiovascular diseases. The review demonstrates promising directions in the search for specific methods of prevention and correction of these pathological conditions.
- Lavie C.J., McAuley P.A., Church T.S., Milani R.V., Blair S.N. Obesity and cardiovascular diseases: implications regarding fitness, fathness, and severity in the obesity paradox. J Am Coll Cardiol 2014; 63(14): 1345–1354, https://doi.org/10.1016/j.jacc.2014.01.022.
- Chrostowska M., Szyndler A., Hoffmann M., Narkiewicz K. Impact of obesity on cardiovascular health. Best Pract Res Clin Endocrinol Metab 2013; 27(2): 147–156, https://doi.org/10.1016/j.beem.2013.01.004.
- Ashraf M.J., Baweja P. Obesity: “huge” problem in cardiovaslular diseases. Mo Med 2013; 110(6): 499–504.
- Kachur S., Lavie C.J., de Schutter A., Milani R.V., Ventura H.O. Obesity and cardiovascular diseases. Minerva Med 2017; 108(3): 212–228, https://doi.org/10.23736/S0026-4806.17.05022-4.
- Parto P., Lavie C.J. Obesity and cardiovascular diseases. Curr Probl Cardiol 2017; 42(11): 376–394, https://doi.org/10.1016/j.cpcardiol.2017.04.004.
- Ortega F.B., Lavie C.J., Blair C.N. Obesity and cardiovascular disease. Circ Res 2016; 118(11): 1752–1770, https://doi.org/10.1161/circresaha.115.306883.
- Landsberg L., Aronne L.J., Beilin L.J., Burke V., Igel L.I., Lloyd-Jones D., Sowers J. Obesity-related hypertension: pathogenesis, cardiovascular risk, and treatment: a position paper of The Obesity Society and the American Society of Hypertension. J Clin Hypertens (Greenwich) 2013; 15(1): 14–33, https://doi.org/10.1111/jch.12049.
- Said S., Mukherjee D., Whayne T.F. Interrelationships with metabolic syndrome, obesity and cardiovascular risk. Curr Vasc Pharmacol 2016; 14(5): 415–425, https://doi.org/10.2174/1570161114666160722121615.
- Zagoskin P.P. Nonspecific energy expenditure and body mass regulation. J Nutr Biol 2019; 5(1): 328–349, https://doi.org/10.18314/jnb.v5i1.1630.
- Hofmann A.F., Hagey L.R. Key discoveries in bile acid chemistry and biology and their clinical applications: history of the last eight decades. J Lipid Res 2014; 55(8): 1553–1595, https://doi.org/10.1194/jlr.R049437.
- Boyer J.L. Bile formation and secretion. Compr Physiol 2013; 3(3): 1035–1078, https://doi.org/10.1002/cphy.c120027.
- Cresci G.A., Bawden E. The gut microbiome: what we do and don’t know. Nutr Clin Pract 2015; 30(6): 734–746, https://doi.org/10.1177/0884533615609899.
- Rajilić-Stojanović M. Function of the microbiota. Best Pract Res Clin Gastroenterol 2013; 27(1): 5–16, https://doi.org/10.1016/j.bpg.2013.03.006.
- Wang Z., Koonen D., Hofker M., Fu J. Gut microbiome and lipid metabolism: from associations to mechanisms. Curr Opin Lipidol 2016; 27(3): 216–224, https://doi.org/10.1097/mol.0000000000000308.
- Voigt R.M., Forsyth C.B., Green S.J., Engen P.A., Keshavarzian A. Circadian rhythm and the gut microbiome. Int Rev Neurobiol 2016; 131: 193–205, https://doi.org/10.1016/bs.irn.2016.07.002.
- Govindarajan K., MacSharry J., Casey P.G., Shanahan F., Joyce S.A., Gahan C.G. Unconjugated bile acids influence expression of circadian genes: a potential mechanism for microbe-host crosstalk. PLoS One 2016; 11(12): e0167319, https://doi.org/10.1371/journal.pone.0167319.
- Joyce S.A., MacSharry J., Casey P.G., Kinsella M., Murphy E.F., Shanahan F., Hill C., Gahan C.G.M. Regulation of host weight gain and lipid metabolism by bacterial bile acid modification in the gut. Proc Natl Acad Sci U S A 2014; 111(20): 7421–7426, https://doi.org/10.1073/pnas.1323599111.
- Ramírez-Pérez O., Cruz-Ramón V., Chinchilla-López P., Méndez-Sánchez N. The role of the gut microbiota in bile acid metabolism. Ann Hepatol 2017; 16(Suppl 1): s15–s20, https://doi.org/10.5604/01.3001.0010.5494.
- Allegretti J.R., Kearney S., Li N., Bogart E., Bullock K., Gerber G.K., Bry L., Clish C.B., Alm E., Korzenik J.R. Recurrent Clostridium difficile infection associates with distinct bile acid and microbiome profiles. Aliment Pharmacol Ther 2016; 43(11): 1142–1153, https://doi.org/10.1111/apt.13616.
- Watanabe M., Fukiya S., Yokota A. Comprehensive evaluation of the bactericidal activities of free bile acids in the large intestine of humans and rodents. J Lipid Res 2017; 58(6): 1143–1152, https://doi.org/10.1194/jlr.M075143.
- Pevsner-Fischer M., Blacher E., Tatirovsky E., Ben-Dov I.Z., Elinav E. The gut microbiome and hypertension. Curr Opin Nephrol Hypertens 2017; 26(1): 1–8, https://doi.org/10.1097/mnh.0000000000000293.
- Gózd-Barszczewska A., Kozioł-Montewka M., Barszczewski P., Młodzińska A., Humińska K. Gut microbiome as a biomarker of cardiometabolic disorders. Ann Agric Environ Med 2017; 24(3): 416–422, https://doi.org/10.26444/aaem/75456.
- Jie Z., Xia H., Zhong S.L., Feng Q., Li S., Liang S., Zhong H., Liu Z., Gao Y., Zhao H., Zhang D., Su Z., Fang Z., Lan Z., Li J., Xiao L., Li J., Li R., Li X., Li F., Ren H., Huang Y., Peng Y., Li G., Wen B., Dong B., Chen J.Y., Geng Q.S., Zhang Z.W., Yang H., Wang J., Wang J., Zhang X., Madsen L., Brix S., Ning G., Xu X., Liu X., Hou Y., Jia H., He K., Kristiansen K. The gut microbiome in atherosclerotic cardiovascular disease. Nat Commun 2017; 8(1): 845, https://doi.org/10.1038/s41467-017-00900-1.
- Tang W.H., Kitai T., Hazen S.L. Gut microbiota in cardiovascular health and disease. Circ Res 2017; 120(7): 1183–1196, https://doi.org/10.1161/circresaha.117.309715.
- Kitai T., Kirsop J., Tang W.H. Exploring the microbiome in heart failure. Curr Heart Fail Rep 2016; 13(2): 103–109, https://doi.org/10.1007/s11897-016-0285-9.
- Sanmiguel C., Gupta A., Mayer E.A. Gut microbiome and obesity: a plausible explanation for obesity. Curr Obes Rep 2016; 4(2): 250–261, https://doi.org/10.1007/s13679-015-0152-0.
- Greathouse K.L., Faucher M.A., Hastings-Tolsma M. The gut microbiome obesity, and weight control in women’s reproductive health. West J Nurs Res 2017; 39(8): 1094–1119, https://doi.org/10.1177/0193945917697223.
- Gérard P. Gut microbiome and obesity. How to prove causality? Ann Am Thorac Soc 2017; 14(Suppl 5): S354–S356, https://doi.org/10.1513/annalsats.201702-117aw.
- Kelly T.N., Bazzano L.A., Ajami N.J., He H., Zhao J., Petrosino J.F., Correa A., He J. Gut microbiome associates with lifetime cardiovascular disease risk profile among Bogalusa Heart Study participants. Circ Res 2016; 119(8): 956–964, https://doi.org/10.1161/circresaha.116.309219.
- Garcia-Rios A., Torres-Peña J.D., Perez-Jimenez F., Perez-Martinez P. Gut microbiota: a new marker of cardiovascular disease. Curr Pharm Des 2017; 23(22): 3233–3238, https://doi.org/10.2174/1381612823666170317144853.
- John G.K., Mullin G.E. The gut microbiome and obesity. Curr Oncol Rep 2018; 18(7): 45, https://doi.org/10.1007/s11912-016-0528-7.
- Yevsyutina Yu.V., Ivashkin V.T. Metabolism of bile acids, liver diseases, and microbiome. Rossijskij zhurnal gastroenterologii, gepatologii, koloproktologii 2018; 28(2): 4–10.
- Staley C., Weingarden A.R., Khoruts A., Sadowsky M.J. Interaction of gut microbiota with bile acid metabolism and its influence on disease states. Appl Microbiol Biotechnol 2017; 101(1): 47–64, https://doi.org/10.1007/s00253-016-8006-6.
- Joyce S.A., Gahan C.G. Disease-associated changes in bile acid profiles and links to altered gut microbiota. Dig Dis 2017; 35(3): 169–177, https://doi.org/10.1159/000450907.
- Rodríguez V.A., Rivoira M.A., Pérez Adel V., Marchionatti A.M., Tolosa de Talamoni N.G. Ursodeoxycholic and deoxycholic acids: differential effects on intestinal Ca2+ uptake, apoptosis and autophagy of rat intestine. Arch Biochem Biophys 2016; 591: 28–34, https://doi.org/10.1016/j.abb.2015.12.006.
- Kim C.H. Immune regulation by microbiome metabolites. Immunology 2018; 154(2): 220–229, https://doi.org/10.1111/imm.12930.
- Patman G. Colorectal cancer: male hormones increase the incidence of colonic adenomas. Nat Rev Gastroenterol Hepatol 2015; 12(1): 4, https://doi.org/10.1038/nrgastro.2014.196.
- Arumugam A., Lissner E.A., Lakshmanaswamy R. The role of hormones and aromatase inhibitors on breast tumor growth and general health in a postmenopausal mouse model. Reprod Biol Endocrinol 2014; 12: 66, https://doi.org/10.1186/1477-7827-12-66.
- Snaterse G., Visser J.A., Arlt W., Hofland J. Circulating steroid hormone variations throughout different stages of prostate cancer. Endocr Relat Cancer 2017; 24(11): R403–R420, https://doi.org/10.1530/erc-17-0155.
- Duggan C., Stanczyk F., Campbell K., Neuhouser M.L., Baumgartner R.N., Baumgartner K.B., Bernstein L., Ballard R., McTiernan A. Associations of sex steroid hormones with mortality in women with breast cancer. Breast Cancer Res Treat 2016; 155(3): 559–567, https://doi.org/10.1007/s10549-016-3704-4.
- Chuffa L.G., Lupi-Júnior L.A., Costa A.B., Amorim J.P., Seiva F.R. The role of sex hormones and steroid receptors on female reproductive cancers. Steroids 2017; 118: 93–108, https://doi.org/10.1016/j.steroids.2016.12.011.
- Vogiatzi M.G., Jacobson-Dickman E., DeBoer M.D.; Drugs, and Therapeutics Committee of the Pediatric Endocrine Society. Vitamin D supplementation and risk of toxicity in pediatrics: a review of current literature. J Clin Endocrinol Metab 2014; 99(4): 1132–1141, https://doi.org/10.1210/jc.2013-3655.
- Arab J.P., Cabrera D., Arrese M. Bile acids in holestasis and its treatment. Ann Hepatol 2017; 16(Suppl 1): S53–S57, https://doi.org/10.5604/01.3001.0010.5497.
- Li T., Apte U. Bile acid metabolism and signaling in cholestasis, inflammation, and cancer. Adv Pharmacol 2015; 74: 263–302, https://doi.org/10.1016/bs.apha.2015.04.003.
- Lemoine C., Bhardwaj T., Bass L.M., Superina R.A. Outcomes following partial external biliary diversion in patients with progressive familial intrahepatic cholestasis. J Pediatr Surg 2017; 52(2): 268–272, https://doi.org/10.1016/j.jpedsurg.2016.11.021.
- Cui Y., Xu B., Zhang X., He Y., Shao Y., Ding M. Diagnostic and therapeutic profiles of serum bile acids in women with intrahepatic cholestasis of pregnancy-a pseudo-targeted metabolomics study. Clin Chim Acta 2018; 483: 135–141, https://doi.org/10.1016/j.cca.2018.04.035.
- Li Z., Lin B., Lin G., Wu Y., Jie Y., Li X., Ko B., Chong Y., Luo J. Circulating FGF19 closely correlates with bile acid synthesis and cholestasis in patients with primary biliary cirrhosis. PLoS One 2017; 12(6): e0178580, https://doi.org/10.1371/journal.pone.0178580.
- Souza R.F. From reflux esophagitis to esophageal adenocarcinoma. Dig Dis 2016; 34(5): 483–490, https://doi.org/10.1159/000445225.
- Farré R. Pathophysiology of gastro-esophageal reflux disease: a role for mucosa integrity? Neurogastroenterol Motil 2013; 25(10): 783–799, https://doi.org/10.1111/nmo.12201.
- Usai Satta P., Oppia F., Cabras F. Overview of pathophysiological features of GERD. Minerva Gastroenterol Dietol 2017; 63(3): 184–197.
- Ikeda Y., Morita S.Y., Terada T. Cholesterol attenuates cytoprotective effects of phosphatidylcholine against bile salts. Sci Rep 2017; 7(1): 306, https://doi.org/10.1038/s41598-017-00476-2.
- Luciano R.L., Castano E., Moeckel G., Perazella M.A. Bile acid nephropathy in a bodybuilder abusing an anabolic androgenic steroid. Am J Kidney Dis 2014; 64(3): 473–476, https://doi.org/10.1053/j.ajkd.2014.05.010.
- Woolbright B.L., Dorko K., Antoine D.J., Clarke J.I., Gholami P., Li F., Kumer S.C., Schmitt T.M., Forster J., Fan F., Jenkins R.E., Park B.K., Hagenbuch B., Olyaee M., Jaeschke H. Bile acid-induced necrosis in primary human hepatocytes and in patients with obstructive cholestasis. Toxicol Appl Pharmacol 2015; 283(3): 168–177, https://doi.org/10.1016/j.taap.2015.01.015.
- Lang E., Pozdeev V.I., Gatidis S., Qadri S.M., Häussinger D., Kubitz R., Herebian D., Mayatepek E., Lang F., Lang K.S., Lang P.A. Bile acid-induced suicidal erythrocyte death. Cell Physiol Biochem 2016, 38(4): 1500–1509, https://doi.org/10.1159/000443091.
- Woolbright B.L., McGill M.R., Yan H., Jaeschke H. Bile acid-induced toxicity in heparg cells recapitulates the response in primary human hepatocytes. Basic Clin Pharmacol Toxicol 2016; 118(2): 160–167, https://doi.org/10.1111/bcpt.12449.
- Gafar A.A., Draz H.M., Goldberg A.A., Bashandy M.A., Bakry S., Khalifa M.A., AbuShair W., Titorenko V.I., Sanderson J.T. Lithocholic acid induces endoplasmic reticulum stress, autophagy and mitochondrial dysfunction in human prostate cancer cells. Peer J 2016; 4: e2445, https://doi.org/10.7717/peerj.2445.
- Woolbright B.L., Li F., Xie Y., Farhood A., Fickert P., Trauner M., Jaeschke H. Lithocholic acid feeding results in direct hepato-toxicity independent of neutrophil function in mice. Toxicol Lett 2014; 228(1): 56–66, https://doi.org/10.1016/j.toxlet.2014.04.001.
- Mostarda S., Passeri D., Carotti A., Cerra B., Colliva C., Benicchi T., Macchiarulo A., Pellicciari R., Gioiello A. Synthesis, physicochemical properties, and biological activity of bile acids 3-glucuronides: novel insights into bile acid signalling and detoxification. Eur J Med Chem 2018; 144: 349–358, https://doi.org/10.1016/j.ejmech.2017.12.034.
- Lee R.H., Ouzounian J.G., Goodwin T.M., Incerpi M.H., Miller D.A., Zhang K., Caulfield M.P., Reitz R., Stanczyk F.Z. Bile acid concentration reference ranges in a pregnant Latina population. Am J Perinatol 2013; 30(5): 389–393, https://doi.org/10.1055/s-0032-1326982.
- Humbert L., Maubert M.A., Wolf C., Duboc H., Mahé M., Farabos D., Seksik P., Mallet J.M., Trugnan G., Masliah J., Rainteau D. Bile acid profiling in human biological samples: comparison of extraction procedures and application to normal and cholestatic patients. J Chromatogr B Analyt Technol Biomed Life Sci 2012; 899: 135–145, https://doi.org/10.1016/j.jchromb.2012.05.015.
- Schmid A., Neumann H., Karrasch T., Liebisch G., Schäffler A. Bile acid metabolome after an oral lipid tolerance test by liquid chromatography-tandem mass spectrometry (LC-MS/MS). PLoS One 2016; 11(2): e0148869, https://doi.org/10.1371/journal.pone.0148869.
- Shah R., John S. Cholestatic jaundice (cholestasis, cholestatic hepatitis). In: StatPearls. Treasure Island (FL): StatPearls Publishing; 2020.
- Horvatits T., Drolz A., Rutter K., Roedl K., Langouche L., Van den Berghe G., Fauler G., Meyer B., Hülsmann M., Heinz G., Trauner M., Fuhrmann V. Circulating bile acids predict outcome in critically ill patients. Ann Intensive Care 2017; 7(1): 48, https://doi.org/10.1186/s13613-017-0272-7.
- Li T., Chiang J.Y. Bile acid signaling in metabolic disease and drug therapy. Pharmacol Rev 2014; 66(4): 948–983, https://doi.org/10.1124/pr.113.008201.
- Mosińska P., Szczepaniak A., Fichna J. Bile acids and FXR in functional gastrointestinal disorders. Dig Liver Dis 2018; 50(8): 795–803, https://doi.org/10.1016/j.dld.2018.05.016.
- Zhou H., Hylemon P.B. Bile acids are nutrient signaling hormones. Steroids 2014; 86: 62–68, https://doi.org/10.1016/j.steroids.2014.04.016.
- Dawson P.A., Karpen S.J. Intestinal transport and metabolism of bile acids. J Lipid Res 2015; 56(6): 1085–1099, https://doi.org/10.1194/jlr.R054114.
- Hofmann A.F., Hagey L.R. Key discoveries in bile acid chemistry and biology and their clinical applications: history of the last eight decades. J Lipid Res 2014; 55(8): 1553–1595, https://doi.org/10.1194/jlr.R049437.
- Strohmeier A., Först G., Tauber P., Schubert R. Membrane/water partition coefficients of bile salts determined using laurdan as a fluorescent probe. Biophys J 2016; 111(8): 1714–1723, https://doi.org/10.1016/j.bpj.2016.08.040.
- Kliewer S.A., Mangelsdorf D.J. Bile acids as hormones: the FXR-FGF15/19 pathway. Dig Dis 2015; 33(3): 327–331, https://doi.org/10.1159/000371670.
- Ye L., Jiang Y., Zuo X. Farnesoid-X-receptor expression in monocrotaline-induced pulmonary arterial hypertension and right heart failure. Biochem Biophys Res Commun 2015; 467(1): 164–170, https://doi.org/10.1016/j.bbrc.2015.09.067.
- Ding L., Yang L., Wang Z., Huang W. Bile acid nuclear receptor FXR and digestive system diseases. Acta Pharm Sin B 2015; 5(2): 135–144, https://doi.org/10.1016/j.apsb.2015.01.004.
- Han C.Y. Update on FXR biology: promising therapeutic target? Int J Mol Sci 2018; 19(7): 2069, https://doi.org/10.3390/ijms19072069.
- De Magalhaes Filho C.D., Downes M., Evans R.M. Farnesoid X receptor an emerging target to combat obesity. Dig Dis 2017; 35(3): 185–190, https://doi.org/10.1159/000450909.
- Parséus A., Sommer N., Sommer F., Caesar R., Molinaro A., Ståhlman M., Greiner T.U., Perkins R., Bäckhed F. Microbiota-induced obesity requires farnesoid X receptor. Gut 2015; 66(3): 429–437, https://doi.org/10.1136/gutjnl-2015-310283.
- Jiang C., Xie C., Lv Y., Li J., Krausz K.W., Shi J., Brocker C.N., Desai D., Amin S.G., Bisson W.H., Liu Y., Gavrilova O., Patterson A.D., Gonzalez F.J. Intestine-selective farnesoid X receptor inhibition improves obesity-related metabolic dysfunction. Nat Commun 2015; 6: 10166, https://doi.org/10.1038/ncomms10166.
- Wang H., He Q., Wang G., Xu X., Hao H. FXR modulators for enterohepatic and metabolic diseases. Expert Opin Ther Pat 2018; 28(11): 765–782, https://doi.org/10.1080/13543776.2018.1527906.
- Moris D., Giaginis C., Tsourouflis G., Theocharis S. Farnesoid-X receptor (FXR) as a promising pharmaceutical target in atherosclerosis. Curr Med Chem 2017; 24(11): 1147–1157, https://doi.org/10.2174/0929867324666170124151940.
- Xia Y., Zhang F., Zhao S., Li Y., Chen X., Gao E., Xu X., Xiong Z., Zhang X., Zhang J., Zhao H., Wang W., Wang H., Guo Y., Liu Y., Li C., Wang S., Zhang L., Yan W., Tao L. Adiponectin determines farnesoid X receptor agonism-mediated cardioprotection against post-infarction remodelling and dysfunction. Cardiovasc Res 2018; 114(10): 1335–1349, https://doi.org/10.1093/cvr/cvy093.
- Jin L., Wang R., Zhu Y., Zheng W., Han Y., Guo F., Ye F.B., Li Y. Selective targeting of nuclear receptor FXR by avermectin analogues with therapeutic effects on nonalcoholic fatty liver disease. Sci Rep 2015; 5: 17288, https://doi.org/10.1038/srep17288.
- Williamson C., Poston L. Impact of FXR and TGR5 agonists on metabolic syndrome arising from early life exposure to bile acids. King’s College London Health Schools Studentships; 2015.
- Chávez-Talavera O., Tailleux A., Lefebvre P., Staels B. Bile acid control of metabolism and inflammation in obesity, type 2 diabetes, dyslipidemia, and nonalcoholic fatty liver disease. Gastroenterol 2017; 152(7): 1679–1694.e3, https://doi.org/10.1053/j.gastro.2017.01.055.
- Bogolyubova A.V., Mayorov A.Y., Mishina E.E., Shwartz A.M., Belousov P.V. Farnesoid X receptor (FXR) as a potential therapeutic target in nonalcoholic fatty liver disease and associated syndromes. Saharnyy diabet 2017; 20(6): 449–453.
- Takeda S., Kadowaki S., Haga T., Takaesu H., Mitaku S. Identification of G protein-coupled receptor genes from the human genome sequence. FEBS Lett 2002; 520(1–3): 97–101, https://doi.org/10.1016/s0014-5793(02)02775-8.
- Keitel V., Stindt J., Häussinger D. Bile acid-activated receptors: GPBAR1 (TGR5) and other G protein-coupled receptors. Handb Exp Pharmacol 2019; 256: 19–49, https://doi.org/10.1007/164_2019_230.
- Svensson P.A., Olsson M., Andersson-Assarsson J.C., Taube M., Pereira M.J., Froguel P., Jacobson P. The TGR5 gene is expressed in human subcutaneous adipose tissue and is associated with obesity, weight loss and resting metabolic rate. Biochem Biophys Res Commun 2013; 433(4): 563–566, https://doi.org/10.1016/j.bbrc.2013.03.031.
- Li T., Chiang J.Y.L. Bile acids as metabolic regulators. Curr Opin Gastroenterol 2015; 31(2): 159–165, https://doi.org/10.1097/MOG.0000000000000156.
- Duboc H., Taché Y., Hofmann A.F. The bile acid TGR5 membrane receptor: from basic research to clinical application. Dig Liver Dis 2015; 46(4): 302–312, https://doi.org/10.1016/j.dld.2013.10.021.
- Deutschmann K., Reich M., Klindt C., Dröge C., Spomer L., Häussinger D., Keitel V. Bile acid receptors in the biliary tree: TGR5 in physiology and disease. Biochim Biophys Acta Mol Basis Dis 2018; 1864(4 Pt B): 1319–1325, https://doi.org/10.1016/j.bbadis.2017.08.021.
- Chen X., Yan L., Guo Z., Chen Y., Li M., Huang C., Chen Z., Meng X. Chenodeoxycholic acid attenuates high-fat diet-induced obesity and hyperglycemia via the G protein-coupled bile acid receptor 1 and proliferator-activated receptor γ pathway. Exp Ther Med 2017; 14(6): 5305–5312, https://doi.org/10.3892/etm.2017.5232.
- Su J., Zhang Q., Qi H., Wu L., Li Y., Yu D., Huang W., Chen W.D., Wang Y.D. The G-protein-coupled bile acid receptor Gpbar1 (TGR5) protects against renal inflammation and renal cancer cell proliferation and migration through antagonizing NF-κB and STAT3-signaling pathways. Oncotarget 2017; 8(33): 54378–54387, https://doi.org/10.18632/oncotarget.17533.
- Guo C., Chen W.D., Wang W.D. TGR5, not only a metabolic regulator. Front Physiol 2016; 7: 646, https://doi.org/10.3389/fphys.2016.00646.
- Agarwal S., Patil A., Aware U., Deshmukh P., Darji B., Sasane S., Sairam K.V., Priyadarsiny P., Giri P., Patel H., Giri S., Jain M., Desai R.C. Discovery of a potent and orally efficacious TGR5 receptor agonist. ACS Med Chem Lett 2016; 7(1): 51−55, https://doi.org/10.1021/acsmedchemlett.5b00323.
- Chen Z., Ning M., Zou O., Cao H., Ye Y., Leng Y., Shen J. Discovery and structure–activity relationship study of 4-phenoxythiazol-5-carboxamides as highly potent TGR5 agonists. Chem Pharm Bull (Tokyo) 2016; 64(4): 326–339, https://doi.org/10.1248/cpb.c15-00905.
- Park E.J., Ahn Y.G., Jung S.H., Bang H.J., Kim M., Hong D.J., Kim J., Suh K.H., Kim Y.J., Kim D., Kim E.Y., Lee K., Min K.H. Discovery of novel pyrimidine and malonamide derivatives as TGR5 agonists. Bioorg Med Chem Lett 2014; 24(17): 4271–4275, https://doi.org/10.1016/j.bmcl.2014.07.026.
- Wang X.X., Wang D., Luo Y., Myakala K., Dobrinskikh E., Rosenberg A.Z., Levi J., Kopp J.B., Field A., Hill A., Lucia S., Qiu L., Jiang T., Peng Y., Orlicky D., Garcia G., Herman-Edelstein M., D‘Agati V., Henriksen K., Adorini L., Pruzanski M., Xie C., Krausz K.W., Gonzalez F.J., Ranjit S., Dvornikov A., Gratton E., Levi M. FXR/TGR5 dual agonist prevents progression of nephropathy in diabetes and obesity. J Am Soc Nephrol 2018; 29(1): 118–137, https://doi.org/10.1681/asn.2017020222.
- Hodge R.G., Nunez D.J. Therapeutic potential of Takeda-G-protein-receptor-5 (TGR5) agonists. Hope or hype? Diabetes Obes Metab 2016; 18(5): 439–443, https://doi.org/10.1111/dom.12636.
- Brighton C.A., Rievaj J., Kuhre R.E., Glass L.L., Schoonjans K., Holst J.J., Gribble F.M., Reimann F. Bile acids trigger GLP-1 release predominantly by accessing basolaterally located G protein-coupled bile acid receptors. Endocrinology 2015; 156(11): 3961–3970, https://doi.org/10.1210/en.2015-1321.
- Trabelsi M.S., Daoudi M., Prawitt J., Ducastel S., Touche V., Sayin S.I., Perino A., Brighton C.A., Sebti Y., Kluza J., Briand O., Dehondt H., Vallez E., Dorchies E., Baud G., Spinelli V., Hennuyer N., Caron S., Bantubungi K., Caiazzo R., Reimann F., Marchetti P., Lefebvre P., Bäckhed F., Gribble F.M., Schoonjans K., Pattou F., Tailleux A., Staels B., Lestavel S. Farnesoid X receptor inhibits glucagon-like peptide-1 production by enteroendocrine L cells. Nat Commun 2015, 6: 7629, https://doi.org/10.1038/ncomms8629.
- Ikura T., Ito N. Crystal structure of the vitamin D receptor ligand-binding domain with lithocholic acids. Vitam Horm 2016; 100: 117–136, https://doi.org/10.1016/bs.vh.2015.10.004.
- Schaap F.G, Trauner M., Jansen P.L. Bile acid receptors as targets for drug development. Nat Rev Gastroenterol Hepatol 2014; 11(1): 55–67, https://doi.org/10.1038/nrgastro.2013.151.
- Chai S.C., Cherian M.T., Wang Y.M., Chen T. Small-molecule modulators of PXR and CAR. Biochim Biophys Acta 2016; 1859(9): 1141–1154, https://doi.org/10.1016/j.bbagrm.2016.02.013.
- McMahon M., Ding S., Jimenez L.A., Terranova R., Gerard M.A., Vitobello A., Moggs J., Henderson C.J., Wolf C.R. Constitutive androstane receptor 1 is constitutively bound to chromatin and ‘primed’ for transactivation in hepatocytes. Mol Pharmacol 2019; 95(1): 97–105, https://doi.org/10.1124/mol.118.113555.
- Mackowiak B., Hodge J., Stern S., Wang H. The roles of xenobiotic receptors: beyond chemical disposition. Drug Metab Dispos 2018; 46(9): 1361–1371, https://doi.org/10.1124/dmd.118.081042.
- Lickteig A.G., Csanaky I.L., Pratt-Hyatt M., Klaassen C.D. Activation of constitutive androstane receptor (CAR) in mice results in maintained biliary excretion of bile acids despite a marked decrease of bile acids in liver. Toxicol Sci 2016; 151(2): 403–418, https://doi.org/10.1093/toxsci/kfw054.
- Carazo A., Hyrsova L., Dusek J., Chodounska H., Horvatova A., Berka K., Bazgier V., Gan-Schreier H., Chamulitrat W., Kudova E., Pavek P. Acetylated deoxycholic (DCA) and cholic (CA) acids are potent ligands of pregnane X (PXR) receptor. Toxicol Lett 2017; 265: 86–96, https://doi.org/10.1016/j.toxlet.2016.11.013.
- Ali A.H., Carey E.J., Lindor K.D. Recent advances in the development of farnesoid X receptor agonists. Ann Transl Med 2015; 3(1): 5, https://doi.org/10.3978/j.issn.2305-5839.2014.12.06.
- Vítek L., Haluzík M. The role of bile acids in the metabolic regulations. J Endocrinol 2016; 228(3): R85–R96, https://doi.org/10.1530/JOE-15-0469.
- Das M., Du Y., Mortensen J.S., Bae H.E., Byrne B., Loland C.J., Kobilka B.K., Chae P.S. An engineered lithocholate-based facial amphiphile stabilizes membrane proteins: assessing the impact of detergent customizability on protein stability. Chemistry 2018; 24(39): 9860–9868, https://doi.org/10.1002/chem.201801141.
- Liaset B., Hao O., Jørgensen H., Hallenborg P., Du Z.Y., Ma T., Marschall H.U., Kruhøffer M., Li R., Li Q., Yde C.C., Criales G., Bertram H.C., Mellgren G., Ofjord E.S., Lock E.J., Espe M., Frøyland L., Madsen L., Kristiansen K. Nutritional regulation of bile acid metabolism is associated with improved pathological characteristics of the metabolic syndrome. J Biol Chem 2011; 286(32): 28382–28395, https://doi.org/10.1074/jbc.M111.234732.
- Ziętak M., Kozak L.P. Bile acids induce uncoupling protein 1-dependent thermogenesis and stimulate energy expenditure at thermoneutrality in mice. Am J Physiol Endocrinol Metab 2016; 310(5): E346–E354, https://doi.org/10.1152/ajpendo.00485.2015.
- Heeren J., Scheja L. Brown adipose tissue and lipid metabolism. Curr Opin Lipidol 2018; 29(3): 180–185, https://doi.org/10.1097/mol.0000000000000504.
- Hanatani S., Motoshima H., Takaki Y., Kawasaki S., Igata M., Matsumura T., Kondo T., Senokuchi T., Ishii N., Kawashima J., Kukidome D., Shimoda S., Nishikawa T., Araki E. Acetate alters expression of genes involved in beige adipogenesis in 3T3-L1 cells and obese KK-Ay mice. J Clin Biochem Nutr 2016; 59(3): 207–214, https://doi.org/10.3164/jcbn.16-23.
- Santos R.S., Frank A.P., Fátima L.A., Palmer B.F., Öz O.K., Clegg D.J. Activation of estrogen receptor alpha induces beiging of adipocytes. Mol Metab 2018; 18: 51–59, https://doi.org/10.1016/j.molmet.2018.09.002.
- Velazquez-Villegas L.A., Perino A., Lemos V., Ziętak M., Nomura M., Pols T.W.H., Schoonjans K. TGR5 signalling promotes mitochondrial fission and beige remodelling of white adipose tissue. Nat Commun 2018; 9(1): 245, https://doi.org/10.1038/s41467-017-02068-0.
- Scheja L., Heeren J. Metabolic interplay between white, beige, brown adipocytes and the liver. J Hepatol 2016; 64(5): 1176–1186, https://doi.org/10.1016/j.jhep.2016.01.025.
- Worthmann A., John C., Rühlemann M.C., Baguhl M., Heinsen F.A., Schaltenberg N., Heine M., Schlein C., Evangelakos I., Mineo C., Fischer M., Dandri M., Kremoser C., Scheja L., Franke A., Shaul P.W., Heeren J. Cold-induced conversion of cholesterol to bile acids in mice shapes the gut microbiome and promotes adaptive thermogenesis. Nat Med 2017; 23(7): 839–849, https://doi.org/10.1038/nm.4357.
- Ziętak M., Kovatcheva-Datchary P., Markiewicz L.H., Ståhlman M., Kozak L.P., Bäckhed F. Altered microbiota contributes to reduced diet-induced obesity upon cold exposure. Cell Metab 2016; 23(6): 1216–1223, https://doi.org/10.1016/j.cmet.2016.05.001.
- Ramadan W., Marsili A., Larsen P.R., Zavacki A.M., Silva J.E. Type-2 iodothyronine 5’deiodinase (D2) in skeletal muscle of C57Bl/6 mice. II. Evidence for a role of D2 in the hypermetabolism of thyroid hormone receptor alpha-deficient mice. Endocrinol 2011; 152(8): 3093–3102, https://doi.org/10.1210/en.2011-0139.
- Broeders E.P., Nascimento E.B., Havekes B., Brans B., Roumans K.H., Tailleux A., Schaart G., Kouach M., Charton J., Deprez B., Bouvy N.D., Mottaghy F., Staels B., van Marken Lichtenbelt W.D., Schrauwen P. The bile acid chenodeoxycholic acid increases human brown adipose tissue activity. Cell Metab 2015; 22(3): 418–426, https://doi.org/10.1016/j.cmet.2015.07.002.
- Teodoro J.S., Rolo A.P., Jarak I., Palmeira C.M., Carvalho R.A. The bile acid chenodeoxycholic acid directly modulates metabolic pathways in white adipose tissue in vitro: insight into how bile acids decrease obesity. NMR Biomed 2016; 29(10): 1391–1402, https://doi.org/10.1002/nbm.3583.
- Yang Q., Vijayakumar A., Kahn B.B. Metabolites as regulators of insulin sensitivity and metabolism. Nat Rev Mol Cell Biol 2018; 19(10): 654–672, https://doi.org/10.1038/s41580-018-0044-8.
- Ma H., Patti M.E. Bile acids, obesity, and the metabolic syndrome. Best Pract Res Clin Gastroenterol 2014; 28(4): 573–583, https://doi.org/10.1016/j.bpg.2014.07.004.
- Kaneko M., Imaizumi K., Saito A., Kanemoto S., Asada R., Matsuhisa K., Ohtake Y. ER stress and disease: toward prevention and treatment. Biol Pharm Bull 2017; 40(9): 1337–1343, https://doi.org/10.1248/bpb.b17-00342.
- Zhu C., Fuchs C.D., Halilbasic E., Trauner M. Bile acids in regulation of inflammation and immunity: friend or foe? Clin Exp Rheumatol 2016; 34(4 Suppl 98): 25–31.
- Lajczak N.K., Saint-Criq V., O’Dwyer A.M., Perino A., Adorini L., Schoonjans K., Keely S.J. Bile acids, deoxycholic acid, and ursodeoxycholic acid differentially regulate human β-defensin-1 and-2 secretion by colonic epithelial cells. FASEB J 2017; 31(9): 3848–3857, https://doi.org/10.1096/fj.201601365R.
- Jiang X., Lian M., Li Y., Zhang W., Wang Q., Wei Y., Zhang J., Chen W., Xiao X., Miao Q., Bian Z., Qiu D., Fang J., Ansari A.A., Leung P.S.C., Coppel R.L., Tang R., Gershwin M.E., Ma X. The immunobiology of mucosal-associated invariant T cell (MAIT) function in primary biliary cholangitis: regulation by cholic acid induced Interleukin-7. J Autoimmun 2018; 90: 64–75, https://doi.org/10.1016/j.jaut.2018.01.007.
- O’Dwyer A.M., Lajczak N.K., Keyes J.A., Ward J.B., Greene C.M., Keely S.J. Ursodeoxycholic acid inhibits TNFα-induced IL-8 release from monocytes. Am J Physiol Gastrointest Liver Physiol 2016; 311(2): G334-G341, https://doi.org/10.1152/ajpgi.00406.2015.
- Chiang J.Y. Bile acid metabolism and signaling. Compr Physiol 2013; 3(3): 1191–1212, https://doi.org/10.1002/cphy.c120023.
- Wammers M., Schupp A.K., Bode J.G., Ehlting C., Wolf S., Deenen R., Köhrer K., Häussinger D., Graf D. Reprogramming of pro-inflammatory human macrophages to an anti-inflammatory phenotype by bile acids. Sci Rep 2018; 8(1): 255, https://doi.org/10.1038/s41598-017-18305-x.
- Sipka S., Bruckner G. The immunomodulatory role of bile acids. Int Arch Allergy Immunol 2014; 165(1): 1–8, https://doi.org/10.1159/000366100.
- Chong E.S. A potential role of probiotics in colorectal cancer prevention: review of possible mechanisms of action. World J Microbiol Biotechnol 2014; 30(2): 351–374, https://doi.org/10.1007/s11274-013-1499-6.
- Massafra V., van Mil S.W.C. Farnesoid X receptor: a “homeostat” for hepatic nutrient metabolism. Biochim Diophys Acta Mol Basis Dis 2018; 1864(1): 45–59, https://doi.org/10.1016/j.bbadis.2017.10.003.
- Zhu Y., Liu H., Zhang M., Guo G.L. Fatty liver diseases, bile acids, and FXR. Acta Pharm Sin B 2016; 6(5): 409–412, https://doi.org/10.1016/j.apsb.2016.07.008.
- Ferrebee C.B., Dawson P.A. Metabolic effects of intestinal absorption and enterohepatic cycling of bile acids. Acta Pharm Sin B 2015; 5(2): 129–134, https://doi.org/10.1016/j.apsb.2015.01.001.
- Feingold K.R. Cholesterol lowering drugs. In: Endotext. South Dartmouth (MA): MDText.com, Inc.; 2020.
- Mueller M., Thorell A., Claudel T., Jha P., Koefeler H., Lackner C., Hoesel B., Fauler G., Stojakovic T., Einarsson C., Marschall H.U., Trauner M. Ursodeoxycholic acid exerts farnesoid X receptor-antagonistic effects on bile acid and lipid metabolism in morbid obesity. J Hepatol 2015; 62(6): 1398–1404, https://doi.org/10.1016/j.jhep.2014.12.034.
- Saltiel A.R. New therapeutic approaches for the treatment of obesity. Sci Transl Med 2016; 8(323): 323rv2, https://doi.org/10.1126/scitranslmed.aad1811.
- Donkers J.M., Roscam Abbing R.L.P., van de Graaf S.F.J. Developments in bile salt based therapies: a critical overview. Biochem Pharmacol 2019; 161: 1–13, https://doi.org/10.1016/j.bcp.2018.12.018.
- Zafrir B., Jain M. Lipid-lowering therapies, glucose control and incident diabetes: evidence, mechanisms and clinical implications. Cardiovasc Drugs Ther 2014; 28(4): 361–377, https://doi.org/10.1007/s10557-014-6534-9.
- Mazidi M., Rezaie P., Karimi E., Kengne A.P. The effects of bile acid sequestrants on lipid profile and blood glucose concentrations: a systematic review and meta-analysis of randomized controlled trials. Int J Cardiol 2017; 227: 850–857, https://doi.org/10.1016/j.ijcard.2016.10.011.
- Hansen M., Scheltema M.J., Sonne D.P., Hansen J.S., Sperling M., Rehfeld J.F., Holst J.J., Vilsbøll T., Knop F.K. Effect of chenodeoxycholic acid and the bile acid sequestrant colesevelam on glucagon-like peptide-1 secretion. Diabetes Obes Metab 2016; 18(6): 571–580, https://doi.org/10.1111/dom.12648.
- Sedgeman L.R., Beysen C., Allen R.M., Ramirez Solano M.A., Turner S.M., Vickers K.C. Intestinal bile acid sequestration improves glucose control by stimulating hepatic miR-182-5p in type 2 diabetes. Am J Physiol Gastrointest Liver Physiol 2018; 315(5): G810–G823, https://doi.org/10.1152/ajpgi.00238.2018.