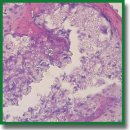
Biological Response to a Novel Hybrid Polyoligomer: in vitro and in vivo Models
The aim of the study is to evaluate biocompatibility of a novel hybrid polyoligomer in in vitro and in vivo models.
Materials and Methods. Cytotoxicity of the material was investigated using the MTT assay with human dermal fibroblasts as test cultures. To study direct interaction of the hybrid polyoligomer with cells, the fibroblasts were cultured on the polymer samples for 96 h, the cultures were assessed every 24 h using fluorescence microscopy. To study the tissue reaction in the area of contact with the donor bed and the morphological features of the implanted sample restructuring, a case-control study was performed using a rabbit model. Samples of hybrid polyoligomer were implanted into the bone defect formed in the left iliac crest in 10 rabbits. In the control group, the prepared allograft samples were transplanted into similar defects in 10 animals. The rabbits were sacrificed 4 and 8 weeks after the operation. The standard morphological methods with hematoxylin and eosin staining and immunohistochemical Ki-67 proliferation marker evaluation were used to assess the state of tissues in the defect area.
Results. The results demonstrate that the hybrid polyoligomer is not cytotoxic (cytotoxicity score 0–1), cells adhere well to its surface, retain their viability and typical morphology throughout the entire observation period. No negative impact of material implantation on the health state and behavior of animals was detected. Morphological examination showed the absence of inflammatory changes, formation of thin-walled capillary vessels, and considerable proliferative activity of mesenchymal cells in the defect area, even though it was more intense than in the control group.
Conclusion. No inflammation signs were detected by 8th week of the experiment. It was defined that new bone was beginning to form. The results of analysis support the conclusion that the developed hybrid materials are prospective for further research as potential bone substitute.
- Kristensen B.B. Autologous tissue transplantations for osteochondral repair. Dan Med J 2016; 63(4): B5236.
- Oryan A., Alidadi S., Moshiri A., Maffulli N. Bone regenerative medicine: classic options, novel strategies, and future directions. J Orthop Surg Res 2014; 9(1): 18, https://doi.org/10.1186/1749-799x-9-18.
- Ehrler D.M., Vaccaro A.R. The use of allograft bone in lumbar spine surgery. Clin Orthop Relat Res 2000; 371: 38–45, https://doi.org/10.1097/00003086-200002000-00005.
- Tadjoedin E.S., de Lange G.L., Lyaruu D.M., Kuiper L., Burger E.H. High concentrations of bioactive glass material (BioGran®) vs. autogenous bone for sinus floor elevation. Clin Oral Implants Res 2002; 13(4): 428–436, https://doi.org/10.1034/j.1600-0501.2002.130412.x.
- Goldstein S.A. Tissue engineering: functional assessment and clinical outcome. Ann N Y Acad Sci 2002; 961: 183–192, https://doi.org/10.1111/j.1749-6632.2002.tb03079.x.
- Badylak S.F., Gilbert T.W. Immune response to biologic scaffold materials. Semin Immunol 2008; 20(2): 109–116, https://doi.org/10.1016/j.smim.2007.11.003.
- Song R., Murphy M., Li C.S., Ting K., Soo C., Zheng Z. Current development of biodegradable polymeric materials for biomedical applications. Drug Des Devel Ther 2018; 12: 3117–3145, https://doi.org/10.2147/dddt.s165440.
- Ritz U., Gerke R., Götz H., Stein S., Rommens P.M. A new bone substitute developed from 3D-prints of polylactide (PLA) loaded with collagen I: an in vitro study. Int J Mol Sci 2017; 18(12): 2569, https://doi.org/10.3390/ijms18122569.
- Shen J., Shi D., Shi C., Li X., Chen M. Fabrication of dopamine modified polylactide-poly(ethylene glycol) scaffolds with adjustable properties. J Biomater Sci Polym Ed 2017; 28(17): 2006–2020, https://doi.org/10.1080/09205063.2017.1366250.
- Mota C., Camarero-Espinosa S., Baker M.B., Wieringa P., Moroni L. Bioprinting: from tissue and organ development to in vitro models. Chem Rev 2020; 120(19): 10547–10607, https://doi.org/10.1021/acs.chemrev.9b00789.
- Leprince J.G., Palin W.M., Hadis M.A., Devaux J., Leloup G. Progress in dimethacrylate-based dental composite technology and curing efficiency. Dent Mater 2013; 29(2): 139–156, https://doi.org/10.1016/j.dental.2012.11.005.
- Deb S., Aiyathurai L., Roether J.A., Luklinska Z.B. Development of high-viscosity, two-paste bioactive bone cements. Biomaterials 2005; 26(17): 3713–3718, https://doi.org/10.1016/j.biomaterials.2004.09.065.
- Behl G., Iqbal J., O’Reilly N.J., McLoughlin P., Fitzhenry L. Synthesis and characterization of poly(2-hydroxyethylmethacrylate) contact lenses containing chitosan nanoparticles as an ocular delivery system for dexamethasone sodium phosphate. Pharm Res 2016; 33(7): 1638–1648, https://doi.org/10.1007/s11095-016-1903-7.
- Yudin V.V., Kovylin R.S., Baten’kin M.A., Kulikova T.I., Chesnokov S.A., Fedushkin I.L., Egorikhina M.N., Rubtsova Y.P., Charykova I.N., Mlyavykh S.G., Aleynik D.Ya. Visible-light induced synthesis of biocompatible porous polymers from oligocarbonatedimethacrylate (OСM-2) in the presence of dialkyl phthalates. Polymer 2020; 192: 122302, https://doi.org/10.1016/j.polymer.2020.122302.
- Kovylin R.S., Baten’kin M.A., Kulikova T.I., Egorikhina M.N., Charikova I.N., Gusev S.A., Rubtsova Y.P., Mlyavykh S.G., Aleynik D.Ya., Chesnokov S.A., Fedushkin I.L. Biocompatible non-toxic porous polymeric materials based on carbonate- and phthalate-containing dimethacrylates materials. ChemistrySelect 2019; 4: 4147, https://doi.org/10.1002/slct.201803810.
- Morozov А.G., Razborov D.A., Egiazaryan T.A., Baten’kin M.A., Aleynik D.Ya., Egorikhina M.N., Rubtsova Yu.P., Charikova I.N., Chesnokov S.A., Fedushkin I.L. In vitro study of degradation behavior, cytotoxicity, and cell adhesion of the atactic polylactic acid for biomedical purposes. J Polym Environ 2020; 28: 2652–2660, https://doi.org/10.1007/s10924-020-01803-x.
- Mossman T. Rapid colorimetric assay for cellular growth and survival: application to proliferation and cytotoxicity assays. J Immunol Methods 1983; 65(1–2): 55–63, https://doi.org/10.1016/0022-1759(83)90303-4.
- Shanmugam S., Copal B. Antimicrobial and cytotoxicity evaluation of. aliovalent substituted hydroxyapatite. Appl Surf Sci 2014; 303: 277–281, https://doi.org/10.1016/j.apsusc.2014.02.166.
- Mapara M., Thomas B.S., Bhat K.M. Rabbit as an animal model for experimental research. Dental Res J (Isfahan) 2012; 9(1): 111–118, https://doi.org/10.4103/1735-3327.92960.
- Zimmermann G., Moghaddam A. Allograft bone matrix versus synthetic bone graft substitutes. Injury 2011; 42(Suppl 2): S16–S21, https://doi.org/10.1016/j.injury.2011.06.199.
- Myciński P., Zarzecka J., Skórska-Stania A., Jelonek A., Okoń K., Wróbel M. Ceramic-polylactide composite material used in a model of healing of osseous defects in rabbits. Pol J Pathol 2017; 68(2): 153–161, https://doi.org/10.5114/pjp.2017.69692.
- Talley A.D., McEnery M.A., Kalpakci K.N., Zienkiewicz K.J., Shimko D.A., Guelcher S.A. Remodeling of injectable, low-viscosity polymer/ceramic bone grafts in a sheep femoral defect model. J Biomed Mater Res B Appl Biomater 2017; 105(8): 2333–2343, https://doi.org/10.1002/jbm.b.33767.
- Ikumi R., Miyahara T., Akino N., Tachikava N., Kasugai S. Guided bone regeneration using a hydrophilic membrane made of unsintered hydroxyapatite and poly(L-lactic acid) in a rat bone-defect model. Dent Mater J 2018; 37(6): 912–918, https://doi.org/10.4012/dmj.2017-385.
- Mahmood S.K., Razak I.A., Ghaji M.S., Yusof L.M., Mahmood Z.K., Rameli M.A.B.P., Zakaria Z.A.B. In vivo evaluation of a novel nanocomposite porous 3D scaffold in a rabbit model: histological analysis. Int J Nanomedicine 2017; 12: 8587–8598, https://doi.org/10.2147/ijn.S145663.
- Jin H.H., Kim D.H., Kim T.W., Shin K.K., Jung J.S., Park H.C., Yoon S.Y. In vivo evaluation of porous hydroxyapatite/chitosan-alginate composite scaffolds for bone tissue engineering. Int J Biol Macromol 2012; 51(5): 1079–1085, https://doi.org/10.1016/j.ijbiomac.2012.08.027.
- Li Z., Ramay H.R., Hauch K.D., Xia D., Zhang M. Chitosan–alginate hybrid scaffolds for bone tissue engineering. Biomaterials 2005; 26(18): 3919–3928, https://doi.org/10.1016/j.biomaterials.2004.09.062.
- Cai K., Yao K., Yang Z., Qu Y., Li X. Histological study of surface modified three dimensional poly (D, L-lactic acid) scaffolds with chitosan in vivo. J Mater Sci Mater Med 2007; 18: 2017–2024, https://doi.org/10.1007/s10856-007-3151-1.