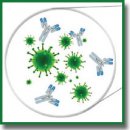
Biosensor Technologies in Medicine: from Detection of Biochemical Markers to Research into Molecular Targets (Review)
Infections are a major cause of premature death. Fast and accurate laboratory diagnostics of infectious diseases is a key condition for the timely initiation and success of treatment. Potentially, it can reduce morbidity, as well as prevent the outbreak and spread of dangerous epidemics. The traditional methods of laboratory diagnostics of infectious diseases are quite time- and labour-consuming, require expensive equipment and trained personnel, which is crucial within limited resources. The fast biosensor-based methods that combine the diagnostic capabilities of biomedicine with modern technological advances in microelectronics, optoelectronics, and nanotechnology make an alternative.
The modern achievements in the development of label-free biosensors make them promising diagnostic tools that combine rapid detection of specific molecular markers, simplicity, ease-of-use, efficiency, accuracy, and cost-effectiveness with the tendency to the development of portable platforms. These qualities exceed the generally accepted standards of microbiological and immunological diagnostics and open up broad prospects for using these analytical systems in clinical practice directly at the site of medical care provision (point-of-care, POC concept).
A wide variety of modern biosensor designs are based on the use of diverse formats of analytical and technological strategies, identification of various regulatory and functional molecular markers associated with infectious pathogens. The solution to the existing problems in biosensing will open up great prospects for these rapidly developing diagnostic biotechnologies.
- Havelaar A.H., Kirk M.D., Torgerson P.R., Gibb H.J., Hald T., Lake R.J., Praet N., Bellinger D.C., De Silva N.R., Gargouri N., Speybroeck N., Cawthorne A., Mathers C., Stein C., Angulo F.J., Devleesschauwer B.; World Health Organization Foodborne Disease Burden Epidemiology Reference Group. World Health Organization global estimates and regional comparisons of the burden of foodborne disease in 2010. PLoS Med 2015; 12(12): e1001923, https://doi.org/10.1371/journal.pmed.1001923.
- Matea C.T., Mocan T., Tabaran F., Pop T., Mosteanu O., Puia C., Iancu C., Mocan L. Quantum dots in imaging, drug delivery and sensor applications. Int J Nanomedicine 2017; 12: 5421–5431, https://doi.org/10.2147/ijn.S138624.
- Sheng L., Lu Y., Deng S., Liao X., Zhang K., Ding T., Gao H., Liu D., Deng R., Li J. A transcription aptasensor: amplified, label-free and culture-independent detection of foodborne pathogens via light-up RNA aptamers. Chem Commun (Camb) 2019; 55(68): 10096–10099, https://doi.org/10.1039/c9cc05036a.
- WHO. Fact sheets: infectious diseases. URL: https://www.who.int/topics/ infectious_diseases/factsheets/ru/.
- Su L., Jia W., Hou C., Lei Y. Microbial biosensors: a review. Biosens Bioelectron 2011; 26(5): 1788–1799, https://doi.org/10.1016/j.bios.2010.09.005.
- Sin M.L., Mach K.E., Wong P.K., Liao J.C. Advances and challenges in biosensor-based diagnosis of infectious diseases. Expert Rev Mol Diagn 2014; 14(2): 225–244, https://doi.org/10.1586/14737159.2014.888313.
- Peltomaa R., Glahn-Martínez B., Benito-Peña E., Moreno-Bondi M.C. Optical biosensors for label-free detection of small molecules. Sensors (Basel) 2018; 18(12): 4126, https://doi.org/10.3390/s18124126.
- Zarei M. Infectious pathogens meet point-of-care diagnostics. Biosens Bioelectron 2018; 106: 193–203, https://doi.org/10.1016/j.bios.2018.02.007.
- Kozel T.R., Burnham-Marusich A.R. Point-of-care testing for infectious diseases: past, present, and future. J Clin Microbiol 2017; 55(8): 2313–2320, https://doi.org/10.1128/jcm.00476-17.
- Kim H., Chung D.R., Kang M. A new point-of-care test for the diagnosis of infectious diseases based on multiplex lateral flow immunoassays. Analyst 2019; 144(8): 2460–2466, https://doi.org/10.1039/c8an02295j.
- Fabri-Faja N., Calvo-Lozano O., Dey P., Terborg R.A., Estevez M.C., Belushkin A., Yesilköy F., Duempelmann L., Altug H., Pruneri V., Lechuga L.M. Early sepsis diagnosis via protein and miRNA biomarkers using a novel point-of-care photonic biosensor. Anal Chim Acta 2019; 1077: 232–242, https://doi.org/10.1016/j.aca.2019.05.038.
- Min J., Nothing M., Coble B., Zheng H., Park J., Im H., Weber G.F., Castro C.M., Swirski F.K., Weissleder R., Lee H. Integrated biosensor for rapid and point-of-care sepsis diagnosis. ACS Nano 2018; 12(4): 3378–3384, https://doi.org/10.1021/acsnano.7b08965.
- Safenkova I.V., Panferov V.G., Panferova N.A., Varitsev Y.A., Zherdev A.V., Dzantiev B.B. Alarm lateral flow immunoassay for detection of the total infection caused by the five viruses. Talanta 2019; 195: 739–744, https://doi.org/10.1016/j.talanta.2018.12.004.
- Zhao Y., Zhang Q., Meng Q., Wu F., Zhang L., Tang Y., Guan Y., An L. Quantum dots-based lateral flow immunoassay combined with image analysis for semiquantitative detection of IgE antibody to mite. Int J Nanomedicine 2017; 12: 4805–4812, https://doi.org/10.2147/IJN.S134539.
- Boisen M.L., Oottamasathien D., Jones A.B., Millett M.M., Nelson D.S., Bornholdt Z.A., Fusco M.L., Abelson D.M., Oda S., Hartnett J.N., Rowland M.M., Heinrich M.L., Akdag M., Goba A., Momoh M., Fullah M., Baimba F., Gbakie M., Safa S., Fonnie R., Kanneh L., Cross R.W., Geisbert J.B., Geisbert T.W., Kulakosky P.C., Grant D.S., Shaffer J.G., Schieffelin J.S., Wilson R.B., Saphire E.O., Branco L.M., Garry R.F., Khan S.H., Pitts K.R.; Viral Hemorrhagic Fever Consortium. Development of prototype filovirus recombinant antigen immunoassays. J Infect Dis 2015; 212(Suppl 2): S359–S367, https://doi.org/10.1093/infdis/jiv353.
- Nielsen K., Yu W.L., Kelly L., Bermudez R., Renteria T., Dajer A., Gutierrez E., Williams J., Algire J., de Eschaide S.T. Development of a lateral flow assay for rapid detection of bovine antibody to Anaplasma marginale. J Immunoassay Immunochem 2008; 29(1): 10–18, https://doi.org/10.1080/15321810701734693.
- Jørgensen C.S., Uldum S.A., Sørensen J.F., Skovsted I.C., Otte S., Elverdal P.L. Evaluation of a new lateral flow test for detection of Streptococcus pneumoniae and Legionella pneumophila urinary antigen. J Microbiol Methods 2015; 116: 33–36, https://doi.org/10.1016/j.mimet.2015.06.014.
- Rohrman B.A., Leautaud V., Molyneux E., Richards-Kortum R.R. A lateral flow assay for quantitative detection of amplified HIV-1 RNA. PLoS One 2012; 7(9): e45611, https://doi.org/10.1371/journal.pone.0045611.
- Kamphee H., Chaiprasert A., Prammananan T., Wiriyachaiporn N., Kanchanatavee A., Dharakul T. Rapid molecular detection of multidrug-resistant tuberculosis by PCR-nucleic acid lateral flow immunoassay. PLos One 2015; 10(9): e0137791, https://doi.org/10.1371/journal.pone.0137791.
- Pilavaki E., Demosthenous A. Optimized lateral flow immunoassay reader for the detection of infectious diseases in developing countries. Sensors (Basel) 2017; 17(11): 2673, https://doi.org/10.3390/s17112673.
- Posthuma-Trumpie G.A., Korf J., van Amerongen A. Lateral flow (immuno)assay: its strengths, weaknesses, opportunities and threats. A literature survey. Anal Bioanal Chem 2008; 393(2): 569–582, https://doi.org/10.1007/s00216-008-2287-2.
- Bhalla N., Jolly P., Formisano N., Estrela P. Introduction to biosensors. Essays Biochem 2016; 60(1): 1–8, https://doi.org/10.1042/ebc20150001.
- Ragavan K.V., Kumar S., Swaraj S., Neethirajan S. Advances in biosensors and optical assays for diagnosis and detection of malaria. Biosens Bioelectron 2018; 105: 188–210, https://doi.org/10.1016/j.bios.2018.01.037.
- Patel S., Nanda R., Sahoo S., Mohapatra E. Biosensors in health care: the milestones achieved in their development towards lab-on-chip-analysis. Biochem Res Int 2016; 2016: 3130469, https://doi.org/10.1155/2016/3130469.
- Biomaterials nanoarchitectonics. Ebara M. (editor). Elsevier Inc; 2016; 362 p., https://doi.org/10.1016/c2014-0-02556-7.
- Biopolymer composites in electronics. Sadasivuni K.K., Ponnamma D., Kim J., Cabibihan J.-J., AlMaadeed M.A. (editors). Elsevier Inc; 2017; 544 p., https://doi.org/10.1016/c2014-0-04575-3.
- Fan X., White I.M., Shopova S.I., Zhu H., Suter J.D., Sun Y. Sensitive optical biosensors for unlabeled targets: a review. Anal Chim Acta 2008; 620(1–2): 8–26, https://doi.org/10.1016/j.aca.2008.05.022.
- Kumar S., Tripathy S., Jyoti A., Singh S.G. Recent advances in biosensors for diagnosis and detection of sepsis: a comprehensive review. Biosens Bioelectron 2019; 124–125: 205–215, https://doi.org/10.1016/j.bios.2018.10.034.
- McLinden T., Sargeant J.M., Thomas M.K., Papadopoulos A., Fazil A. Component costs of foodborne illness: a scoping review. BMC Public Health 2014; 14: 509, https://doi.org/10.1186/1471-2458-14-509.
- Mazzaracchio V., Neagu D., Porchetta A., Marcoccio E., Pomponi A., Faggioni G., D’Amore N., Notargiacomo A., Pea M., Moscone D., Palleschi G., Lista F., Arduini F. A label-free impedimetric aptasensor for the detection of Bacillus anthracis spore simulant. Biosens Bioelectron 2019; 126: 640–646, https://doi.org/10.1016/j.bios.2018.11.017.
- Waller D.F., Hew B.E., Holdaway C., Jen M., Peckham G.D. Rapid detection of Bacillus anthracis spores using immunomagnetic separation and amperometry. Biosensors (Basel) 2016; 6(4): 61, https://doi.org/10.3390/bios6040061.
- Wynn D., Deo S., Daunert S. Engineering rugged field assays to detect hazardous chemicals using spore-based bacterial biosensors. Methods Enzymol 2017; 589: 51–85, https://doi.org/10.1016/bs.mie.2017.02.005.
- Compendium of chemical terminology. Nič M., Jirát J., Košata B., Jenkins A., McNaught A. (editors). Oxford: Blackwell Scientific Publications; 1997, https://doi.org/10.1351/goldbook.
- Novick A., Weiner M. Enzyme induction as an all-or-none phenomenon. Proc Natl Acad Sci U S A 1957; 43(7): 553–566, https://doi.org/10.1073/pnas.43.7.553.
- Jacob F., Monod J. Genetic regulatory mechanisms in the synthesis of proteins. J Mol Biol 1961; 3: 318–356, https://doi.org/10.1016/s0022-2836(61)80072-7.
- Wu X., Xu C., Tripp R.A., Huang Y.W., Zhao Y. Detection and differentiation of foodborne pathogenic bacteria in mung bean sprouts using field deployable label-free SERS devices. Analyst 2013; 138(10): 3005–3012, https://doi.org/10.1039/c3an00186e.
- van der Meer J.R., Belkin S. Where microbiology meets microengineering: design and applications of reporter bacteria. Nat Rev Microbiol 2010; 8(7): 511–522, https://doi.org/10.1038/nrmicro2392.
- Marks H., Schechinger M., Garza J., Locke A., Coté G. Surface enhanced Raman spectroscopy (SERS) for in vitro diagnostic testing at the point of care. Nanophotonics 2017; 6(4): 681–701, https://doi.org/10.1515/nanoph-2016-0180.
- Kahraman M., Mullen E.R., Korkmaz A., Wachsmann-Hogiu S. Fundamentals and applications of SERS-based bioanalytical sensing. Nanophotonics 2017; 6(5): 831–852, https://doi.org/10.1515/nanoph-2016-0174.
- Urmann K., Reich P., Walter J.G., Beckmann D., Segal E., Scheper T. Rapid and label-free detection of protein a by aptamer-tethered porous silicon nanostructures. J Biotechnol 2017; 257: 171–177, https://doi.org/10.1016/j.jbiotec.2017.01.005.
- Qiu C., Zhai H., Hou J. Biosensors design in yeast and applications in metabolic engineering. FEMS Yeast Res 2019; 19(8): foz082, https://doi.org/10.1093/femsyr/foz082.
- González-Pabón M.J., Figueredo F., Martínez-Casillas D.C., Cortón E. Characterization of a new composite membrane for point of need paper-based micro-scale microbial fuel cell analytical devices. PLoS One 2019; 14(9): e0222538, https://doi.org/10.1371/journal.pone.0222538.
- Lim J.W., Ha D., Lee J., Lee S.K., Kim T. Review of micro/nanotechnologies for microbial biosensors. Front Bioeng Biotechnol 2015; 3: 61, https://doi.org/10.3389/fbioe.2015.00061.
- Lei Y., Chen W., Mulchandani A. Microbial biosensors. Anal Chim Acta 2006; 568(1–2): 200–210, https://doi.org/10.1016/j.aca.2005.11.065.
- Lee T., Park S.Y., Jang H., Kim G.H., Lee Y., Park C., Mohammadniaei M., Lee M.H., Min J. Fabrication of electrochemical biosensor consisted of multi-functional DNA structure/porous au nanoparticle for avian influenza virus (H5N1) in chicken serum. Mater Sci Eng C Mater Biol Appl 2019; 99: 511–519, https://doi.org/10.1016/j.msec.2019.02.001.
- Faria H.A.M., Zucolotto V. Label-free electrochemical DNA biosensor for zika virus identification. Biosens Bioelectron 2019; 131: 149–155, https://doi.org/10.1016/j.bios.2019.02.018.
- Teengam P., Siangproh W., Tuantranont A., Vilaivan T., Chailapakul O., Henry C.S. Electrochemical impedance-based DNA sensor using pyrrolidinyl peptide nucleic acids for tuberculosis detection. Anal Chim Acta 2018; 1044: 102–109, https://doi.org/10.1016/j.aca.2018.07.045.
- Zhang S., Ma L., Ma K., Xu B., Liu L., Tian W. Label-free aptamer-based biosensor for specific detection of chloramphenicol using AIE probe and graphene oxide. ACS Omega 2018; 3(10): 12886–12892, https://doi.org/10.1021/acsomega.8b01812.
- Zhang F.Z., Keasling J. Biosensors and their applications in microbial metabolic engineering. Trends Microbiol 2011; 19(7): 323–329, https://doi.org/10.1016/j.tim.2011.05.003.
- Dietrich J.A., McKee A.E., Keasling J.D. High-throughput metabolic engineering: advances in small-molecule screening and selection. Annu Rev Biochem 2010; 79: 563–590, https://doi.org/10.1146/annurev-biochem-062608-095938.
- Durrieu C., Lagarde F., Jaffrezic-Renault N. Nanotechnology assets in biosensors design for environmental monitoring. In: Brayner R., Fiévet F., Coradin T. (editors). Nanomaterials: a danger or a promise? London: Springer; 2013; p. 189–229, https://doi-org-443.webvpn.jnu.edu. cn/10.1007/978-1-4471-4213-3_7.
- Yagi K. Applications of whole-cell bacterial sensors in biotechnology and environmental science. Appl Microbiol Biotechnol 2007; 73(6): 1251–1258, https://doi.org/10.1007/s00253-006-0718-6.
- Renella G., Giagnoni L. Light dazzles from the black box: whole-cell biosensors are ready to inform on fundamental soil biological processes. Chem Biol Technol Agric 2016; 3: 8, https://doi.org/10.1186/s40538-016-0059-3.
- Park M., Tsai S.L., Chen W. Microbial biosensors: engineered microorganisms as the sensing machinery. Sensors (Basel) 2013; 13(5): 5777–5795, https://doi.org/10.3390/s130505777.
- Roy V., Adams B.L., Bentley W.E. Developing next generation antimicrobials by intercepting AI-2 mediated quorum sensing. Enzym Microb Technol 2011; 49(2): 113–123, https://doi.org/10.1016/j.enzmictec.2011.06.001.
- Mangwani N., Dash H.R., Chauhan A., Das S. Bacterial quorum sensing: functional features and potential applications in biotechnology. J Mol Microb Biotechnol 2012; 22(4): 215–227, https://doi.org/10.1159/000341847.
- Amine A., Arduini F., Moscone D., Palleschi G. Recent advances in biosensors based on enzyme inhibition. Biosens Bioelectron 2016; 76: 180–194, https://doi.org/10.1016/j.bios.2015.07.010.
- Rocchitta G., Spanu A., Babudieri S., Latte G., Madeddu G., Galleri G., Nuvoli S., Bagella P., Demartis M.I., Fiore V., Manetti R., Serra P.A. Enzyme biosensors for biomedical applications: strategies for safeguarding analytical performances in biological fluids. Sensors (Basel) 2016; 16(6): 780, https://doi.org/10.3390/s16060780.
- Sang S., Wang Y., Feng Q., Wei Y., Ji J., Zhang W. Progress of new label-free techniques for biosensors: a review. Crit Rev Biotechnol 2016; 36(3): 465–481, https://doi.org/10.3109/07388551.2014.991270.
- Liu X., Marrakchi M., Xu D., Dong H., Andreescu S. Biosensors based on modularly designed synthetic peptides for recognition, detection and live/dead differentiation of pathogenic bacteria. Biosens Bioelectron 2016; 80: 9–16, https://doi.org/10.1016/j.bios.2016.01.041.
- Golichenari B., Velonia K., Nosrati R., Nezami A., Farokhi-Fard A., Abnous K., Behravan J., Tsatsakis A.M. Label-free nano-biosensing on the road to tuberculosis detection. Biosens Bioelectron 2018; 113: 124–135, https://doi.org/10.1016/j.bios.2018.04.059.
- Golichenari B., Nosrati R., Farokhi-Fard A., Faal Maleki M., Gheibi Hayat S.M., Ghazvini K., Vaziri F., Behravan J. Electrochemical-based biosensors for detection of Mycobacterium tuberculosis and tuberculosis biomarkers. Crit Rev Biotechnol 2019; 39(8): 1056–1077, https://doi.org/10.1080/07388551.2019.1668348.
- Mowbray S.E., Amiri A.M. A brief overview of medical fiber optic biosensors and techniques in the modification for enhanced sensing ability. Diagnostics (Basel) 2019; 9(1): 23, https://doi.org/10.3390/diagnostics9010023.
- Gharatape A., Yari Khosroushahi A. Optical biomarker-based biosensors for cancer/infectious disease medical diagnoses. Appl Immunohistochem Mol Morphol 2019; 27(4): 278–286, https://doi.org/10.1097/PAI.0000000000000586.
- Russell C., Ward A.C., Vezza V., Hoskisson P., Alcorn D., Steenson D.P., Corrigan D.K. Development of a needle shaped microelectrode for electrochemical detection of the sepsis biomarker interleukin-6 (IL-6) in real time. Biosens Bioelectron 2019; 126: 806–814, https://doi.org/10.1016/j.bios.2018.11.053.
- Abisado R.G., Benomar S., Klaus J.R., Dandekar A.A., Chandler J.R. Bacterial quorum sensing and microbial community interactions. mBio 2018; 9(5): e01749-18, https://doi.org/10.1128/mbio.01749-18.
- Sin M.L., Mach K.E., Wong P.K., Liao J.C. Advances and challenges in biosensor-based diagnosis of infectious diseases. Expert Rev Mol Diagn 2014; 14(2): 225–244, https://doi.org/10.1586/14737159.2014.888313.
- Bhardwaj N., Bhardwaj S.K., Mehta J., Kim K.H., Deep A. MOF-bacteriophage biosensor for highly sensitive and specific detection of Staphylococcus aureus. ACS Appl Mater Interfaces 2017; 9(39): 33589–33598, https://doi.org/10.1021/acsami.7b07818.
- Nasrin F., Chowdhury A.D., Takemura K., Lee J., Adegoke O., Deo V.K., Abe F., Suzuki T., Park E.Y. Single-step detection of norovirus tuning localized surface plasmon resonance-induced optical signal between gold nanoparticles and quantum dots. Biosens Bioelectron 2018; 122: 16–24, https://doi.org/10.1016/j.bios.2018.09.024.
- Damborský P., Švitel J., Katrlík J. Optical biosensors. Essays Biochem 2016; 60(1): 91–100, https://doi.org/10.1042/ebc20150010.
- Nquyet N.T., Yen L.T.H., Doan V.Y., Hoang N.L., Van Thu V., Lan H., Trung T., Pham V.H., Tam P.D. A label-free and highly sensitive DNA biosensor based on the core-shell structured CeO2-NR@Ppy nanocomposite for Salmonella detection. Mater Sci Eng C Mater Biol Appl 2019; 96: 790–797, https://doi.org/10.1016/j.msec.2018.11.059.
- Campuzano S., Yáñez-Sedeño P., Pingarrón J.M. Molecular biosensors for electrochemical detection of infectious pathogens in liquid biopsies: current trends and challenges. Sensors (Basel) 2017; 17(11): 2533, https://doi.org/10.3390/s17112533.
- Liu Y., Zhou H., Hu Z., Yu G., Yang D., Zhao J. Label and label-free based surface-enhanced Raman scattering for pathogen bacteria detection: a review. Biosens Bioelectron 2017; 94: 131–140, https://doi.org/10.1016/j.bios.2017.02.032.
- Barreiros dos Santos M., Agusil J.P., Prieto-Simón B., Sporer C., Teixeira V., Samitier J. Highly sensitive detection of pathogen Escherichia coli O157:H7 by electrochemical impedance spectroscopy. Biosens Bioelectron 2013; 45: 174–180, https://doi.org/10.1016/j.bios.2013.01.009.
- Zhang J., Oueslati R., Cheng C., Zhao L., Chen J., Almeida R., Wu J. Rapid, highly sensitive detection of gram-negative bacteria with lipopolysaccharide based disposable aptasensor. Biosens Bioelectron 2018; 112: 48–53, https://doi.org/10.1016/j.bios.2018.04.034.
- Huang Y., Xu J., Liu J., Wang X., Chen B. Disease-related detection with electrochemical biosensors: a review. Sensors (Basel) 2017; 17(10): 2375, https://doi.org/10.3390/s17102375.
- Zanchetta G., Lanfranco R., Giavazzi F., Bellini T., Buscaglia М. Emerging applications of label-free optical biosensors. Nanophotonics 2017; 6(4): 627–645, https://doi.org/10.1515/nanoph-2016-0158.
- Dudak F.C., Boyaci I.H. Rapid and label-free bacteria detection by surface plasmon resonance (SPR) biosensors. Biotechnol J 2009; 4(7): 1003–1011, https://doi.org/10.1002/biot.200800316.
- Yang F., Chang T.L., Liu T., Wu D., Du H., Liang J., Tian F. Label-free detection of Staphylococcus aureus bacteria using long-period fiber gratings with functional polyelectrolyte coatings. Biosens Bioelectron 2019; 133: 147–153, https://doi.org/10.1016/j.bios.2019.03.024.
- Muniandy S., Teh S.J., Appaturi J.N., Thong K.L., Lai C.W., Ibrahim F., Leo B.F. A reduced graphene oxide-titanium dioxide nanocomposite based electrochemical aptasensor for rapid and sensitive detection of Salmonella enterica. Bioelectrochemistry 2019; 127: 136–144, https://doi.org/10.1016/j.bioelechem.2019.02.005.
- Wang Z., Yao X., Wang R., Ji Y., Yue T., Sun J., Li T., Wang J., Zhang D. Label-free strip sensor based on surface positively charged nitrogen-rich carbon nanoparticles for rapid detection of Salmonella enteritidis. Biosens Bioelectron 2019; 132: 360–367, https://doi.org/10.1016/j.bios.2019.02.061.
- Srinivasan S., Ranganathan V., DeRosa M.C., Murari B.M. Label-free aptasensors based on fluorescent screening assays for the detection of Salmonella typhimurium. Anal Biochem 2018; 559: 17–23, https://doi.org/10.1016/j.ab.2018.08.002.
- Zhang Y., Tian J., Li K., Tian H., Xu W. Label-free visual biosensor based on cascade amplification for the detection of Salmonella. Anal Chim Acta 2019; 1075: 144–151, https://doi.org/10.1016/j.aca.2019.05.020.
- Silva N.F.D., Magalhães J.M.C.S., Barroso M.F., Oliva-Teles T., Freire C., Delerue-Matos C. In situ formation of gold nanoparticles in polymer inclusion membrane: application as platform in a label-free potentiometric immunosensor for Salmonella typhimurium detection. Talanta 2019; 194: 134–142, https://doi.org/10.1016/j.talanta.2018.10.024.
- Han S., Soylu M.C., Kirimli C.E., Wu W., Sen B., Joshi S.G., Emery C.L., Au G., Niu X., Hamilton R., Krevolin K., Shih W.H., Shih W.Y. Rapid, label-free genetic detection of enteropathogens in stool without genetic isolation or amplification. Biosens Bioelectron 2019; 130: 73–80, https://doi.org/10.1016/j.bios.2019.01.025.
- Lin P.H., Huang S.C., Chen K.P., Li B.R., Li Y.K. Effective construction of a high-capacity boronic acid layer on a quartz crystal microbalance chip for high-density antibody immobilization. Sensors (Basel) 2019; 19(1): 28, https://doi.org/10.3390/s19010028.
- Wu R., Ma Y., Pan J., Lee S.H., Liu J., Zhu H., Gu R., Shea K.J., Pan G. Efficient capture, rapid killing and ultrasensitive detection of bacteria by a nano-decorated multi-functional electrode sensor. Biosens Bioelectron 2018; 101: 52–59, https://doi.org/10.1016/j.bios.2017.10.003.
- Kimuda S.G., Biraro I.A., Bagaya B.S., Raynes J.G., Cose S. Characterising antibody avidity in individuals of varied Mycobacterium tuberculosis infection status using surface plasmon resonance. PLoS One 2018; 13(10): e0205102, https://doi.org/10.1371/journal.pone.0205102.
- Rebelo R., Barbosa A.I., Caballero D., Kwon I.K., Oliveira J.M., Kundu S.C., Reis R.L., Correlo V.M. 3D biosensors in advanced medical diagnostics of high mortality diseases. Biosens Bioelectron 2019; 130: 20–39, https://doi.org/10.1016/j.bios.2018.12.057.
- Erdem Ö., Saylan Y., Cihangir N., Denizli A. Molecularly imprinted nanoparticles based plasmonic sensors for real-time Enterococcus faecalis detection. Biosens Bioelectron 2019; 126: 608–614, https://doi.org/10.1016/j.bios.2018.11.030.
- Hoyos-Nogués M., Gil F.J., Mas-Moruno C. Antimicrobial peptides: powerful biorecognition elements to detect bacteria in biosensing technologies. Molecules 2018; 23(7): 1683, https://doi.org/10.3390/molecules23071683.
- Liébana S., Brandão D., Alegret S., Pividori M.I. Electrocehmical immunosensors, genosensors and phagosensors for Salmonella detection. Anal Methods 2014; 6(22): 8858–8873, https://doi.org/10.1039/c4ay01373e.
- Cui F., Xu Y., Wang R., Liu H., Chen L., Zhang Q., Mu X. Label-free impedimetric glycan biosensor for quantitative evaluation interactions between pathogenic bacteria and mannose. Biosens Bioelectron 2018; 103: 94–98, https://doi.org/10.1016/j.bios.2017.11.068.
- Bu T., Huang Q., Yan L., Zhang W., Dou L., Huang L., Yang Q., Zhao B., Yang B., Li T., Wang J., Zhang D. Applicability of biological dye tracer in strip biosensor for ultrasensitive detection of pathogenic bacteria. Food Chem 2019; 274: 816–821, https://doi.org/10.1016/j.foodchem.2018.09.066.
- Zarei S.S., Soleimanian-Zad S., Ensafi A.A. An impedimetric aptasensor for Shigella dysenteriae using a gold nanoparticle-modified glassy carbon electrode. Mikrochim Acta 2018; 185(12): 538, https://doi.org/10.1007/s00604-018-3075-0.
- Chuensirikulchai K., Laopajon W., Phunpae P., Apiratmateekul N., Surinkaew S., Tayapiwatana C., Pata S., Kasinrerk W. Sandwich antibody-based biosensor system for identification of Mycobacterium tuberculosis complex and nontuberculous mycobacteria. J Immunoassay Immunochem 2019; 40(6): 590–604, https://doi.org/10.1080/15321819.2019.1659814.
- Savas S., Altintas Z. Graphene quantum dots as nanozymes for electrochemical sensing of Yersinia enterocolitica in milk and human serum. Materials (Basel) 2019; 12(13): 2189, https://doi.org/10.3390/ma12132189.
- Chang H.J., Voyvodic P.L., Zúñiga A., Bonnet J. Microbially derived biosensors for diagnosis, monitoring and epidemiology. Microb Biotechnol 2017; 10(5): 1031–1035, https://doi.org/10.1111/1751-7915.12791.
- Kuss S., Amin H.M.A., Compton R.G. Electrochemical detection of pathogenic bacteria-recent strategies, advances and challenges. Chem Asian J 2018; 13(19): 2758–2769, https://doi.org/10.1002/asia.201800798.
- Singh R., Mukherjee M.D., Sumana G., Gupta R.K., Sood S., Malhotra B.D. Biosensors for pathogen detection: a smart approach towards clinical diagnosis. Sens Actuators B Chem 2014; 197: 385–404, https://doi.org/10.1016/j.snb.2014.03.005.
- Zhou Q., Son K., Liu Y., Revzin A. Biosensors for cell analysis. Annu Rev Biomed Eng 2015; 17: 165–190, https://doi.org/10.1146/annurev-bioeng-071114-040525.