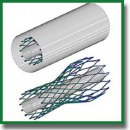
Mechanism of Vascular Injury in Transcatheter Aortic Valve Replacement
The aim of the study was to determine the potential mechanism of vascular complications due to “catheter–vascular wall” interaction in transcatheter aortic valve replacement using experimental and numerical analysis.
Materials and Methods. A series of full-scale bench tests and numerical simulations were carried out using the CoreValve commercial transfemoral delivery system for aortic valve bioprosthesis (Medtronic Inc., USA). Full-scale tests were carried out using a phantom of the vascular system (a polymeric silicone model of Transcatheter Aortic Valve; Trandomed 3D Inc., China) with simulation of all stages of delivery system movement along the vascular bed. They involved introduction into the common femoral artery, movement along the abdominal and thoracic parts of the aorta, the aortic arch, and positioning the system to the implantation site. The force arising from the passage of the delivery system was assessed using sensors of a Z50 universal testing machine (Zwick/Roell, Germany). Numerical simulation of transcatheter valve replacement procedure was carried out in a similar way with allowance for the patient-specific anatomy of the recipient’s aorta using the finite element method in the Abaqus/CAE environment (Dassault Systèmes, France).
Results. It was found that in the process of the delivery system passing through the vascular system, there occurred force fluctuations associated with catheter bending and its interaction with the aortic wall in the region of its arch. For example, in the initial straight portions, the pushing force was 3.8–7.9 N; the force increased to the maximum (11.1 and 14.4 N with and without the prosthesis) with bending of the distal portion of the catheter. A similar increase was observed when performing numerical simulation with high-quality graphic visualization of stress on the “spots” of contact between the catheter and the vascular wall with an increase in stress to 0.8 MPa.
Conclusion. Numerical and full-scale bench tests prove the significant effect of the properties of delivery system catheter for transcatheter aortic valve replacement on the interaction with the aortic walls.
- Ielasi A., Latib A., Tespili M., Donatelli F. Current results and remaining challenges of trans-catheter aortic valve replacement expansion in intermediate and low risk patients. Int J Cardiol Heart Vasc 2019; 23: 100375, https://doi.org/10.1016/j.ijcha.2019.100375.
- Hellhammer K., Piayda K., Afzal S., Kleinebrecht L., Makosch M., Hennig I., Quast C., Jung C., Polzin A., Westenfeld R., Kelm M., Zeus T., Veulemans V. The latest evolution of the Medtronic CoreValve system in the era of transcatheter aortic valve replacement. JACC Cardiovasc Interv 2018; 11: 2314–2322, https://doi.org/10.1016/j.jcin.2018.07.023.
- Ojeda S., Hidalgo F., Romero M., Mazuelos F., Suárez de Lezo J., Martín E., Lostalo A., Luque A., González R., Fernández A., López-Aguilera J., Segura J., Guerrero N., Pan M. Impact of the repositionable Evolut R CoreValve system on the need for a permanent pacemaker after transcatheter aortic valve implantation in patients with severe aortic stenosis. Catheter Cardiovasc Interv 2020; 95(4): 783–790, https://doi.org/10.1002/ccd.28327.
- Chaudhry M.A., Sardar M.R. Vascular complications of transcatheter aortic valve replacement: a concise literature review. World J Cardiol 2017; 9(7): 574–582, https://doi.org/10.4330/wjc.v9.i7.574.
- Dandeniyage L.S., Adhikari R., Bown M., Shanks R., Adhikari B., Easton C.D., Gengenbach T.R., Cookson D., Gunatillake P.A. Morphology and surface properties of high strength siloxane poly(urethane-urea)s developed for heart valve application. J Biomed Mater Res B Appl Biomater 2019; 107(1): 112–121, https://doi.org/10.1002/jbm.b.34101.
- Ullery B.W., Jin R., Kirker E.B., Hayes G., Siwek L., Brevig J., Hodson R.W., Spinelli K.J. Trends in vascular complications and associated treatment strategies following transfemoral transcatheter aortic valve replacement. J Vasc Surg 2020; 72(4): 1313-1324.e5, https://doi.org/10.1016/j.jvs.2020.01.050.
- Hayashida K., Lefèvre T., Chevalier B., Hovasse T., Romano M., Garot P., Mylotte D., Uribe J., Farge A., Donzeau-Gouge P., Bouvier E., Cormier B., Morice M.C. Transfemoral aortic valve implantation. JACC Cardiovasc Interv 2011; 4: 851–858, https://doi.org/10.1016/j.jcin.2011.03.019.
- Heinisch P.P., Richter O., Schünke M., Bombien Quaden R. Transcatheter valve implantation: damage to the human aorta after valved stent delivery system exposure — an in vitro study. Interact Cardiovasc Thorac Surg 2012; 15(3): 352–356, https://doi.org/10.1093/icvts/ivs225.
- Popma J.J., Deeb G.M., Yakubov S.J., Mumtaz M., Gada H., O’Hair D., Bajwa T., Heiser J.C., Merhi W., Kleiman N.S., Askew J., Sorajja P., Rovin J., Chetcuti S.J., Adams D.H., Teirstein P.S., Zorn G.L. 3rd, Forrest J.K., Tchétché D., Resar J., Walton A., Piazza N., Ramlawi B., Robinson N., Petrossian G., Gleason T.G., Oh J.K., Boulware M.J., Qiao H., Mugglin A.S., Reardon M.J.; Evolut Low Risk Trial Investigators. Transcatheter aortic-valve replacement with a self-expanding valve in low-risk patients. N Engl J Med 2019; 380: 1706–1715, https://doi.org/10.1056/nejmoa1816885.
- Salaun E., Pibarot P., Rodés-Cabau J. Transcatheter aortic valve replacement: procedure and outcomes. Cardiol Clin 2020; 38(1): 115–128, https://doi.org/10.1016/j.ccl.2019.09.007.
- Rotman O.M., Kovarovic B., Sadasivan C., Gruberg L., Lieber B.B., Bluestein D. Realistic vascular replicator for TAVR procedures. Cardiovasc Eng Technol 2018; 9(3): 339–350, https://doi.org/10.1007/s13239-018-0356-z.
- Caldwell R.A., Woodell J.E., Ho S.P., Shalaby S.W., Boland T., Langan E.M., LaBerge M. In vitro evaluation of phosphonylated low-density polyethylene for vascular applications. J Biomed Mater Res 2002; 62(4): 514–524, https://doi.org/10.1002/jbm.10249.
- Nagaoka S., Akashi R. Low-friction hydrophilic surface for medical devices. Biomaterials 1990; 11(6): 419–424, https://doi.org/10.1016/0142-9612(90)90098-b.
- García-Herrera C.M., Atienza J.M., Rojo F.J., Claes E., Guinea G.V., Celentano D.J., García-Montero C., Burgos R.L. Mechanical behaviour and rupture of normal and pathological human ascending aortic wall. Med Biol Eng Comput 2012; 50: 559–566, https://doi.org/10.1007/s11517-012-0876-x.