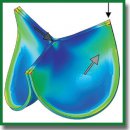
Study of Biomechanics of the Heart Valve Leaflet Apparatus Using Numerical Simulation Method
The aim of the study was to study the complex biomechanics of the aortic valve prosthesis and to analyze the effect of frame mobility on the stress-strain state and geometry of the valve leaflet apparatus using a numerical simulation method, which reproduces the qualitative and quantitative results of its bench tests.
Materials and Methods. The object of the study was a commercial valve bioprosthesis UniLine (NeoCor, Russia), a three-dimensional mesh of which was obtained on the basis of computer microtomography with a subsequent analysis of its stress-strain state in the systole–diastole cycle by the finite element method in the Abaqus/CAE medium. The simulation was validated by comparing the results of numerical and bench simulation on the ViVitro Labs hydrodynamic system (ViVitro Labs Inc., Canada).
Results. The method proposed in this study to simulate the mobility of commissural struts by including elastic connectors of adjustable stiffness in the calculation made it possible to reproduce the qualitative effects of the valve leaflet work observed in the bench experiment. The bioprosthetic orifice area in the systolic phase corresponded to the values obtained in the hydrodynamic system throughout the entire systole–diastole cycle. The analysis of the stress-strain state has shown the fundamental difference in the distribution of the von Mises stress fields depending on the numerical experiment design: the concentration of high amplitudes in the area of commissural struts and the central part of the free edge. However, quantitatively, the stress values reached the maximum of 0.850–0.907 MPa (0.141–0.156 MPa on average), which is below the ultimate strength of the biological material.
Conclusion. The results of this study with the validation performed allowed us to conclude that adequate results of modeling the biomechanics of the heart valve leaflet bioprosthesis based on the finite element method can be achieved by using a high-resolution model with the imposition of elastic connectors in the area of commissural struts. Taking into account the mobility of the frame struts of the heart valve prosthesis is decisive in relation to the final geometry of the valve apparatus and can act as a negative factor in case of a highly elastic material of the valve apparatus. The simulation method presented can be used to optimize the leaflet apparatus geometry of heart valve prostheses from the standpoint of assessing the distribution of the stress-strain state.
- Abbasi M., Azadani A.N. A geometry optimization framework for transcatheter heart valve leaflet design. J Mech Behav Biomed Mater 2020; 102: 103491, https://doi.org/10.1016/j.jmbbm.2019.103491.
- Dabiri Y., Ronsky J., Ali I., Basha A., Bhanji A., Narine K. Effects of leaflet design on transvalvular gradients of bioprosthetic heart valves. Cardiovasc Eng Technol 2016; 7(4): 363–373, https://doi.org/10.1007/s13239-016-0279-5.
- Li K., Sun W. Simulated transcatheter aortic valve deformation: a parametric study on the impact of leaflet geometry on valve peak stress. Int J Numer Method Biomed Eng 2017; 33(3): e02814, https://doi.org/10.1002/cnm.2814.
- De Gaetano F., Bagnoli P., Zaffora A., Pandolfi A., Serrani M., Brubert J., Stasiak J., Moggridge G.D., Costantino M.L. A newly developed tri-leaflet polymeric heart valve prosthesis. J Mech Med Biol 2015; 15(02): 1540009, https://doi.org/10.1142/s0219519415400096.
- Hsu M.C., Kamensky D., Xu F., Kiendl J., Wang C., Wu M.C.H., Mineroff J., Reali A., Bazilevs Y., Sacks M.S. Dynamic and fluid–structure interaction simulations of bioprosthetic heart valves using parametric design with T-splines and Fung-type material models. Comput Mech 2015; 55(6): 1211–1225, https://doi.org/10.1007/s00466-015-1166-x.
- Martin C., Sun W. Comparison of transcatheter aortic valve and surgical bioprosthetic valve durability: a fatigue simulation study. J Biomech 2015; 48(12): 3026–3034, https://doi.org/10.1016/j.jbiomech.2015.07.031.
- Balu A., Nallagonda S., Xu F., Krishnamurthy A., Hsu M.C., Sarkar S. A deep learning framework for design and analysis of surgical bioprosthetic heart valves. Sci Rep 2019; 9(1): 18560, https://doi.org/10.1038/s41598-019-54707-9.
- Glushkova T.V., Ovcharenko E.A., Batranin A.V., Klyshnikov K.Yu., Kudryavtseva Yu.A., Barbarash L.S. A case report of bioprosthetic valve dysfunction after tricuspid valve replacement in a preschool patient: the contribution of pannus and calcification. Vestnik transplantologii i iskusstvennyh organov 2018; 20(3): 45–53, https://doi.org/10.15825/1995-1191-2018-3-45-53.
- Glushkova T.V., Ovcharenko E.A., Rogulina N.V., Klyshnikov K.Yu., Kudryavtseva Yu.A., Barbarash L.S. Dysfunction patterns of epoxy-treated tissue heart valves. Kardiologiia 2019; 59(10): 49–59, https://doi.org/10.18087/cardio.2019.10.n327.
- Mukhamadiyarov R.A., Rutkovskaya N.V., Milto I.V., Vasyukov G.Yu., Barbarash L.S. Pathogenetic parallels between native and bioprosthetic aortic valve calcification. Geny i Kletki 2016; 11(3): 72–79.
- Karas’kov A.M., Zheleznev S.I., Nazarov V.M., Lavinyukov S.O., Larionov P.M., Bogachev-Prokof’ev A.V., Glotova N.I., Matyugin M.P. Clinical and morphological changes in dysfunctions of biological heart prostheses. Patologia krovoobrasenia i kardiohirurgia 2006; 2: 21–26.
- Foroutan F., Guyatt G.H., O’Brien K., Bain E., Stein M., Bhagra S., Sit D., Kamran R., Chang Y., Devji T., Mir H., Manja V., Schofield T., Siemieniuk R.A., Agoritsas T., Bagur R., Otto C.M., Vandvik P.O. Prognosis after surgical replacement with a bioprosthetic aortic valve in patients with severe symptomatic aortic stenosis: systematic review of observational studies. BMJ 2016; 354: i5065, https://doi.org/10.1136/bmj.i5065.
- Odarenko Y.N., Rutkovskaya N.V., Rogulina N.V., Stasev A.N., Kokorin S.G., Kagan E.S., Barbarash L.S. Analysis of 23-year experience epoxy treated xenoaortic bioprosthesisin surgery mitral heart disease. Research factors of recipients by positions of influence on the development of calcium degeneration. Kompleksnye problemy serdecno-sosudistyh zabolevanij 2015; 4: 17–25.
- Rogulina N.V., Odarenko Y.N., Zhuravleva I.Y., Barbarash L.S. Remote results of application of mechanical and biological prostheses at patients of various age. Journal of Siberian Medical Sciences 2014; 3: 47.
- Zhuravleva I.Y., Bogachev-Prokophiev A.V., Timchenko T.P., Trebushat D.V., Mayorov A.P., Goncharenko A.M., Astapov D.A., Nushtaev D.V., Demidov D.P. A model aortic valve bioprosthesis for sutureless implantation. Medicinskaa tehnika 2017; 3: 15–18.
- Abbasi M., Qiu D., Behnam Y., Dvir D., Clary C., Azadani A.N. High resolution three-dimensional strain mapping of bioprosthetic heart valves using digital image correlation. J Biomech 2018; 76: 27–34, https://doi.org/10.1016/j.jbiomech.2018.05.020.
- Abbasi M., Barakat M.S., Vahidkhah K., Azadani A.N. Characterization of three-dimensional anisotropic heart valve tissue mechanical properties using inverse finite element analysis. J Mech Behav Biomed Mater 2016; 62: 33–44, https://doi.org/10.1016/j.jmbbm.2016.04.031.
- Abbasi M., Barakat M., Dvir D., Azadani A. Detailed stress analysis of Edwards-SAPIEN and Medtronic CoreValve devices. Is leaflet stress comparable to surgical Carpentier-Edwards PERIMOUNT Magna bioprosthesis? Struct Heart 2019; 3(Suppl 1): 192, https://doi.org/10.1080/24748706.2019.1591103.
- Liang L., Sun B. A proof of concept study of using machine-learning in artificial aortic valve design: from leaflet design to stress analysis. Bioengineering (Basel) 2019; 6(4): 104, https://doi.org/10.3390/bioengineering6040104.
- Vesely I. The evolution of bioprosthetic heart valve design and its impact on durability. Cardiovasc Pathol 2003; 12(5): 277–286, https://doi.org/10.1016/s1054-8807(03)00075-9.
- Mao W., Li K., Sun W. Fluid–structure interaction study of transcatheter aortic valve dynamics using smoothed particle hydrodynamics. Cardiovasc Eng Technol 2016; 7(4): 374–388, https://doi.org/10.1007/s13239-016-0285-7.
- Zakerzadeh R., Hsu M.C., Sacks M.S. Computational methods for the aortic heart valve and its replacements. Expert Rev Med Devices 2017; 14(11): 849–866, https://doi.org/10.1080/17434440.2017.1389274.
- Zhuravleva I.Y., Nushtaev D.V., Timchenko T.V., Trebushat D.V., Mayorov А.P., Zheleznev S.I., Demidov D.P., Bogachev-Prokophiev А.V. The concept of a device for the redo transcatheter mitral valve implantation. Sovremennye tehnologii v medicine 2017; 9(3): 7–14, https://doi.org/10.17691/stm2017.9.3.01.
- Zhang W., Rossini G., Kamensky D., Bui-Thanh T., Sacks M.S. Isogeometric finite element-based simulation of the aortic heart valve: integration of neural network structural material model and structural tensor fiber architecture representations. Int J Numer Method Biomed Eng 2021; 37(4): e3438, https://doi.org/10.1002/cnm.3438.
- Smuts A.N., Blaine D.C., Scheffer C., Weich H., Doubell A.F., Dellimore K.H. Application of finite element analysis to the design of tissue leaflets for a percutaneous aortic valve. J Mech Behav Biomed Mater 2011; 4(1): 85–98, https://doi.org/10.1016/j.jmbbm.2010.09.009.
- Soares J.S., Feaver K.R., Zhang W., Kamensky D., Aggarwal A., Sacks M.S. Biomechanical behavior of bioprosthetic heart valve heterograft tissues: characterization, simulation, and performance. Cardiovasc Eng Technol 2016; 7(4): 309–351, https://doi.org/10.1007/s13239-016-0276-8.
- Serrani M., Brubert J., Stasiak J., De Gaetano F., Zaffora A., Costantino M.L., Moggridge G.D. A computational tool for the microstructure optimization of a polymeric heart valve prosthesis. J Biomech Eng 2016; 138(6): 061001, https://doi.org/10.1115/1.4033178.
- Abbasi M., Barakat M.S., Dvir D., Azadani A.N. A non-invasive material characterization framework for bioprosthetic heart valves. Ann Biomed Eng 2019; 47(1): 97–112, https://doi.org/10.1007/s10439-018-02129-5.
- Lee J.H., Rygg A.D., Kolahdouz E.M., Rossi S., Retta S.M., Duraiswamy N., Scotten L.N., Craven B.A., Griffith B.E. Fluid–structure interaction models of bioprosthetic heart valve dynamics in an experimental pulse duplicator. Ann Biomed Eng 2020; 48(5): 1475–1490, https://doi.org/10.1007/s10439-020-02466-4.
- Vriesendorp M.D., de Lind van Wijngaarden R.A.F., Rao V., Moront M.G., Patel H.J., Sarnowski E., Vatanpour S., Klautz R.J.M. An in vitro comparison of internally versus externally mounted leaflets in surgical aortic bioprostheses. Interact Cardiovasc Thorac Surg 2020; 30(3): 417–423, https://doi.org/10.1093/icvts/ivz277.
- Martin C., Sun W. Simulation of long-term fatigue damage in bioprosthetic heart valves: effects of leaflet and stent elastic properties. Biomech Model Mechanobiol 2014; 13(4): 759–770, https://doi.org/10.1007/s10237-013-0532-x.
- Fedorov S.A., Chiginev V.A., Zhurko S.A., Gamzaev A.B., Medvedev A.P. Clinical and hemodynamic results of applying different biological prosthesis models for correction of calcific aortic valve disease. Sovremennye tehnologii v medicine 2016; 8(4): 292–296.
- Barbarash L.S., Rogulina N.V., Rutkovskaya N.V., Odarenko Yu.N., Stasev A.N., Levadin Yu.V., Kokorin S.G. 5-year experience of mitral valve replacement using Uniline bioprosthesis. Kardiologia i serdecno-sosudistaa hirurgia 2015; 8(5): 49–54, https://doi.org/10.17116/kardio20158549-54.
- Karas’kov A.M., Zheleznev S.I., Rogulina N.V., Sapegin A.V., Odarenko Yu.N., Levadin Yu.V., Rutkovskaya N.V., Barbarash L.S. Next generation russian biological prosthesis “UniLin” for mitral valve replacement: first experience. Grudnaa i serdecno-sosudistaa hirurgia 2017; 59(2): 98–104, https://doi.org/10.24022/0236-2791-2017-59-2-98-104.