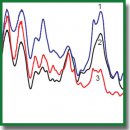
Express Analysis of Cartilage Tissue Using Multivariate Analysis of IR Spectra
The aim of the study was to develop a diagnostic method for the quantitative determination of the main components of cartilage tissue of various types based on multivariate IR spectral analysis and verification of data using classical chemical analysis.
Materials and Methods. Cartilages of the nasal septum, knee joint, rib, and nucleus pulposus of the intervertebral disc, as well as trypsinized and defective cartilage samples, were examined as samples. The IR spectra of the cartilage samples, as well as calibration mixtures of collagen and chondroitin sulfate, were obtained. The IR spectra were collected using the attenuated total reflectance techniques, and their processing was performed using the TQ Analyst software and the principal component regression calibration technique. Based on calibration dependence, the Ksp coefficient was determined as the ratio of the mass fractions of collagen and chondroitin sulfate. Its value was compared with the value of Kchem, equal to the ratio of the mass fractions of collagen and chondroitin sulfate, obtained using the classical chemical analysis of these substances.
Results. The IR spectra of cartilage tissues are a superposition of the IR spectra of collagen and chondroitin sulfate and qualitatively reflect their composition. A change in the ratio between the relative intensities of the characteristic bands of compounds in the IR spectrum is obvious only with a significant change in the content of these compounds in cartilage. This change occurs after trypsinization, when Ksp increases from 0.88±0.05 (Kchem~0.8) to 4.55. The use of a calibration model with a complete analysis of the cartilage IR spectrum made it possible to determine the difference in the ratio of the main components in the matrix of different samples in the absence of obvious changes in the IR spectra. Thus, a statistically significant decrease in the content of chondroitin sulfate in degraded articular cartilage (Ksp=4.4±1.8; Kchem~5.5) was shown compared with intact samples (Ksp=2.8±1.1; Kchem~2.6).
Conclusion. IR spectrometric express analysis of cartilage tissue employing the principal component regression method allows a correct determination of the ratio of the main components in the cartilage matrix, those of collagen and glycosaminoglycans. The proposed technique includes one measurement, does not require prolonged and laborious sample preparation, does not require long, multi-stage and laborious chemical manipulations to determine each of the components, and makes it possible to determine the features and changes in the composition for a large set of samples of cartilage tissue of different types. In future, this approach can be used for non-invasive diagnostics of cartilage tissue.
- Silver F.H., Kato Y.P., Ohno M., Wasserman A.J. Analysis of mammalian connective tissue: relationship between hierarchical structures and mechanical properties. J Long Term Eff Med Implants 1992; 2(2–3): 165–198.
- Muir H. The chondrocyte, architect of cartilage. Biomechanics, structure, function and molecular biology of cartilage matrix macromolecules. Bioassays 1995; 17(12): 1039–1048, https://doi.org/10.1002/bies.950171208.
- Rotter N., Tobias G., Lebl M., Roy A.K., Hansen M.C., Vacanti C.A., Bonassar L.J. Age-related changes in the composition and mechanical properties of human nasal cartilage. Arch Biochem Biophys 2002; 403(1): 132–140, https://doi.org/10.1016/s0003-9861(02)00263-1.
- Homicz M.R., McGowan K.B., Lottman L.M., Beh G., Sah R.L., Watson D. A compositional analysis of human nasal septal cartilage. Arch Facial Plast Surg 2003; 5(1): 53–58, https://doi.org/10.1001/archfaci.5.1.53.
- Karchner J.P., Yousefi F., Bitman S.R., Darvish K., Pleshko N. Non-destructive spectroscopic assessment of high and low weight bearing articular cartilage correlates with mechanical properties. Cartilage 2019; 10(4): 480–490, https://doi.org/10.1177/1947603518764269.
- Li G., Thomson M., Dicarlo E., Yang X., Nestor B., Bostrom M.P.G., Camacho N.P. A chemometric analysis for evaluation of early-stage cartilage degradation by infrared fiber-optic probe spectroscopy. Appl Spectrosc 2005; 59(12): 1527–1533, https://doi.org/10.1366/000370205775142593.
- Mrosek E.H., Lahm A., Erggelet C., Uhl M., Kurz H., Eissner B., Schagemann J.C. Subchondral bone trauma causes cartilage matrix degeneration: an immunohistochemical analysis in a canine model. Osteoarthritis Cartilage 2006; 14(2): 171–178, https://doi.org/10.1016/j.joca.2005.08.004.
- West P.A., Bostrom M.P.G., Torzilli P.A., Camacho N.P. Fourier transform infrared spectral analysis of degenerative cartilage: an infrared fiber optic probe and imaging study. Appl Spectrosc 2004; 58(4): 376–381, https://doi.org/10.1366/000370204773580194.
- Franz T., Hasler E.M., Hagg R., Weiler C., Jakob R.P., Mainil-Varlet P. In situ compressive stiffness, biochemical composition, and structural integrity of articular cartilage of the human knee joint. Osteoarthritis Cartilage 2001; 9(6): 582–592, https://doi.org/10.1053/joca.2001.0418.
- Laverty S., O’Kouneff S., Ionescu M., Reiner A., Pidoux I., Webber C., Rossier Y., Billinghurst R.C., Poole A.R. Excessive degradation of type II collagen in articular cartilage in equine osteochondrosis. J Orthop Res 2002; 20(6): 1282–1289, https://doi.org/10.1016/S0736-0266(02)00053-0.
- Yin J., Xia Y. Proteoglycan concentrations in healthy and diseased articular cartilage by Fourier transform infrared imaging and principal component regression. Spectrochim Acta A Mol Biomol Spectrosc 2014; 133: 825–830, https://doi.org/10.1016/j.saa.2014.05.092.
- Yin J., Xia Y. Macromolecular concentrations in bovine nasal cartilage by Fourier transform infrared imaging and principal component regression. Appl Spectrosc 2010; 64(11): 1199–1208, https://doi.org/10.1366/000370210793335124.
- Baykal D., Irrechukwu O., Lin P.C., Fritton K., Spencer R.G., Pleshko N. Nondestructive assessment of engineered cartilage constructs using near-infrared spectroscopy. Appl Spectrosc 2010; 64(10): 1160–1166, https://doi.org/10.1366/000370210792973604.
- Gordetsov A.S. Infrared spectroscopy of biological fluids and tissues. Sovremennye tehnologii v medicine 2010; 1: 84.
- Kogan L.P., Kislitsyn D.I., Krasnikova O.V., Medyanik I.A., Gordetsov A.S. Diagnosis of a disease using the values of statistical functionals calculated from infrared spectroscopic parameters of blood. Sovremennye tehnologii v medicine 2017; 9(4): 25–35, https://doi.org/10.17691/stm2017.9.4.03.
- Querido W., Kandel S., Pleshko N. Applications of vibrational spectroscopy for analysis of connective tissues. Molecules 2021; 26(4): 922, https://doi.org/10.3390/molecules26040922.
- Potter K., Kidder L.H., Levin I.W., Lewis E.N., Spencer R.G. Imaging of collagen and proteoglycan in cartilage sections using Fourier transform infrared spectral imaging. Arthritis Rheum 2001; 44(4): 846–855, https://doi.org/10.1002/1529-0131 (200104)44:4846::aid-anr1413.0.co;2-e.
- Camacho N.P., West P., Torzilli P.A., Mendelsohn R. FTIR microscopic imaging of collagen and proteoglycan in bovine cartilage. Biopolymers 2001; 62(1): 1–8, https://doi.org/10.1002/1097-0282 (2001)62:11::aid-bip103.0.co;2-o.
- Ramakrishnan N., Xia Y., Bidthanapally A. Polarized IR microscopic imaging of articular cartilage. Phys Med Biol 2007; 52(15): 4601–4614, https://doi.org/10.1088/0031-9155/52/15/016.
- Farndale R.W., Buttle D.J., Barrett A.J. Improved quantitation and discrimination of sulphated glycosaminoglycans by use of dimethylmethylene blue. Biochim Biophys Acta 1986; 883(2): 173–177, https://doi.org/10.1016/0304-4165(86)90306-5.
- Rodionova O.E. Interval’nyy metod obrabotki rezul’tatov mnogokanal’nykh eksperimentov. Dis. … dokt. fiz.-mat. nauk [Interval method for processing the results of multichannel experiments. DSc Thesis]. Moscow; 2008.
- Edwards H.G.M., Farwell D.W., Holder J.M., Lawson E.E. Fourier-transform Raman spectroscopy of ivory: II. Spectroscopic analysis and assignments. J Mol Struct 1997; 435(1): 49–58, https://doi.org/10.1016/s0022-2860(97)00122-1.
- Manoharan R., Wang Y., Feld M.S. Histochemical analysis of biological tissues using Raman spectroscopy. Spectrochim Acta A Mol Biomol Spectrosc 1996; 52(2): 215–249, https://doi.org/10.1016/0584-8539(95)01573-6.
- Cael J.J., Isaac D.H., Blackwell J., Koenig J.L., Atkins E.D.T., Sheehan J.K. Polarized infrared spectra of crystalline glycosaminoglycans. Carbohydr Res 1976; 50(2): 169–179, https://doi.org/10.1016/s0008-6215(00)83848-3.
- Servaty R., Schiller J., Binder H., Arnold K. Hydration of polymeric components of cartilage — an infrared spectroscopic study on hyaluronic acid and chondroitin sulfate. Int J Biol Macromol 2001; 28(2): 121–127, https://doi.org/10.1016/s0141-8130(00)00161-6.
- Bank R.A., Krikken M., Beekman B., Stoop R., Maroudas A., Lafeber F.P., te Koppele J.M. A simplified measurement of degraded collagen in tissues: application in healthy, fibrillated and osteoarthritic cartilage. Matrix Biol 1997; 16(5): 233–243, https://doi.org/10.1016/s0945-053x(97)90012-3.
- Peters T.J., Smillie I.S. Studies on chemical composition of menisci from the human knee-joint. Proc R Soc Med 1971; 64(3): 261–262, https://doi.org/10.1177/003591577106400310.
- Ghosh P., Taylor T.K.F., Horsburgh B.A. The composition and protein metabolism in the immature rabbit intervertebral disc. Cell Tissue Res 1975; 163(2): 223–238, https://doi.org/10.1007/bf00221729.
- Mizuno H., Roy A.K., Vacanti C.A., Kojima K., Ueda M., Bonassar L.J. Tissue-engineered composites of anulus fibrosus and nucleus pulposus for intervertebral disc replacement. Spine (Phila Pa 1976) 2004; 29(12): 1290–1298, https://doi.org/10.1097/01.brs.0000128264.46510.27.
- Fermor H.L., McLure S.W.D., Taylor S.D., Russell S.L., Williams S., Fisher J., Ingham E. Biological, biochemical and biomechanical characterisation of articular cartilage from the porcine, bovine and ovine hip and knee. Biomed Mater Eng 2015; 25(4): 381–395, https://doi.org/10.3233/bme-151533.
- Goldberg R.L., Kolibas L.M. An improved method for determining proteoglycans synthesized by chondrocytes in culture. Connect Tissue Res 1990; 24(3–4): 265–275, https://doi.org/10.3109/03008209009152154.
- Buckwalter J.A., Roughley P.J., Rosenberg L.C. Age-related changes in cartilage proteoglycans: quantitative electron microscopic studies. Microsc Res Tech 1994; 28(5): 398–408, https://doi.org/10.1002/jemt.1070280506.
- Warren S. A critical analysis of the 1,9-dimethylmethylene blue assay for sulfated glycosaminoglycans in synovial fluid. MSc Thesis. Guelph, Ontario: University of Guelph; 2000.