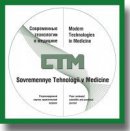
Modern Rehabilitation Technologies of Patients with Motor Disorders at an Early Rehabilitation of Stroke (Review)
Cerebral stroke is one of the leading disability causes among adult population worldwide. The number of post-stroke patients, who need rehabilitation including motor recovery, keeps growing annually. Standard motor rehabilitation techniques have a limited effect on recovering extremity motor defunctionalization. In this regard, in recent years, new technologies of post-stroke rehabilitation are being suggested. The present review summarizes the existing literature data on current techniques applied in patients with motor disorders at an early rehabilitation period of cerebral stroke. The current modern technologies are divided into the methods based on “interhemispheric inhibition” theory (repetitive transcranial magnetic stimulation, transcranial direct current stimulation), and on “mirror neurons” theory (virtual reality systems and brain–computer interfaces). The authors present the neurophysiological causes and feasible protocols of using the techniques in clinical practice, the clinical research findings due to the initial severity level of motor disorders and stroke age, as well as the factors contributing to the motor rehabilitation efficiency when using these methods.
- GBD 2019 Stroke Collaborators. Global, regional, and national burden of stroke and its risk factors, 1990–2019: a systematic analysis for the Global Burden of Disease Study 2019. Lancet Neurol 2021; 20(10): 795–820, https://doi.org/10.1016/s1474-4422(21)00252-0.
- Feigin V.L., Brainin M., Norrving B., Martins S., Sacco R.L., Hacke W., Fisher M., Pandian J., Lindsay P. World Stroke Organization (WSO): Global Stroke Fact Sheet 2022. Int J Stroke 2022; 17(1): 18–29, https://doi.org/10.1177/17474930211065917.
- Katan M., Luft A. Global burden of stroke. Semin Neurol 2018; 38(2): 208–211, https://doi.org/10.1055/s-0038-1649503.
- Hatem S.M., Saussez G., Della Faille M., Prist V., Zhang X., Dispa D., Bleyenheuft Y. Rehabilitation of motor function after stroke: a multiple systematic review focused on techniques to stimulate upper extremity recovery. Front Hum Neurosci 2016; 10: 442, https://doi.org/10.3389/fnhum.2016.00442.
- Wang Z.R., Wang P., Xing L., Mei L.P., Zhao J., Zhang T. Leap motion-based virtual reality training for improving motor functional recovery of upper limbs and neural reorganization in subacute stroke patients. Neural Regen Res 2017; 12(11): 1823–1831, https://doi.org/10.4103/1673-5374.219043.
- Twitchell T.E. The restoration of motor function following hemiplegia in man. Brain 1951; 74(4): 443–480, https://doi.org/10.1093/brain/74.4.443.
- Haghighi F.M., Kordi Yoosefinejad A., Razeghi M., Shariat A., Bagheri Z., Rezaei K. The effect of high-frequency repetitive transcranial magnetic stimulation on functional indices of affected upper limb in patients with subacute stroke. J Biomed Phys Eng 2021; 11(2): 175–184, https://doi.org/10.31661/jbpe.v0i0.879.
- Baniqued P.D.E., Stanyer E.C., Awais M., Alazmani A., Jackson A.E., Mon-Williams M.A., Mushtaq F., Holt R.J. Brain–computer interface robotics for hand rehabilitation after stroke: a systematic review. J Neuroeng Rehabil 2021; 18(1): 15, https://doi.org/10.1186/s12984-021-00820-8.
- van Lieshout E.C.C., van der Worp H.B., Visser-Meily J.M.A., Dijkhuizen R.M. Timing of repetitive transcranial magnetic stimulation onset for upper limb function after stroke: a systematic review and meta-analysis. Front Neurol 2019; 10: 1269, https://doi.org/10.3389/fneur.2019.01269.
- Dobkin B.H. Clinical practice. Rehabilitation after stroke. N Engl J Med 2005; 352(16): 1677–1684, https://doi.org/10.1056/nejmcp043511.
- Grefkes C., Fink G.R. Recovery from stroke: current concepts and future perspectives. Neurol Res Pract 2020; 2: 17, https://doi.org/10.1186/s42466-020-00060-6.
- Ward N.S., Cohen L.G. Mechanisms underlying recovery of motor function after stroke. Arch Neurol 2004; 61(12): 1844–1848, https://doi.org/10.1001/archneur.61.12.1844.
- Murase N., Duque J., Mazzocchio R., Cohen L.G. Influence of interhemispheric interactions on motor function in chronic stroke. Ann Neurol 2004; 55(3): 400–409, https://doi.org/10.1002/ana.10848.
- Du J., Yang F., Hu J., Hu J., Xu Q., Cong N., Zhang Q., Liu L., Mantini D., Zhang Z., Lu G., Liu X. Effects of high- and low-frequency repetitive transcranial magnetic stimulation on motor recovery in early stroke patients: evidence from a randomized controlled trial with clinical, neurophysiological and functional imaging assessments. Neuroimage Clin 2019; 21: 101620, https://doi.org/10.1016/j.nicl.2018.101620.
- Kobayashi M., Pascual-Leone A. Transcranial magnetic stimulation in neurology. Lancet Neurol 2003; 2(3): 145–456, https://doi.org/10.1016/s1474-4422(03)00321-1.
- Adeyemo B.O., Simis M., Macea D.D., Fregni F. Systematic review of parameters of stimulation, clinical trial design characteristics, and motor outcomes in non-invasive brain stimulation in stroke. Front Psychiatry 2012; 3: 88, https://doi.org/10.3389/fpsyt.2012.00088.
- Hummel F.C., Cohen L.G. Non-invasive brain stimulation: a new strategy to improve neurorehabilitation after stroke? Lancet Neurol 2006; 5(8): 708–712, https://doi.org/10.1016/s1474-4422(06)70525-7.
- Volz L.J., Vollmer M., Michely J., Fink G.R., Rothwell J.C., Grefkes C. Time-dependent functional role of the contralesional motor cortex after stroke. Neuroimage Clin 2017; 16: 165–174, https://doi.org/10.1016/j.nicl.2017.07.024.
- Luk K.Y., Ouyang H.X., Pang M.Y.C. Low-frequency rTMS over contralesional M1 increases ipsilesional cortical excitability and motor function with decreased interhemispheric asymmetry in subacute stroke: a randomized controlled study. Neural Plast 2022; 2022: 3815357, https://doi.org/10.1155/2022/3815357.
- Xu J., Branscheidt M., Schambra H., Steiner L., Widmer M., Diedrichsen J., Goldsmith J., Lindquist M., Kitago T., Luft A.R., Krakauer J.W., Celnik P.A.; SMARTS Study Group. Rethinking interhemispheric imbalance as a target for stroke neurorehabilitation. Ann Neurol 2019; 85(4): 502–513, https://doi.org/10.1002/ana.25452.
- Klomjai W., Katz R., Lackmy-Vallée A. Basic principles of transcranial magnetic stimulation (TMS) and repetitive TMS (rTMS). Ann Phys Rehabil Med 2015; 58(4): 208–213, https://doi.org/10.1016/j.rehab.2015.05.005.
- Chervyakov A.V., Poydasheva A.G., Korzhova J.E., Suponeva N.A., Chernikova L.A., Piradov M.A. Repetitive transcranial magnetic stimulation in neurology and psychiatry. Zhurnal nevrologii i psihiatrii imeni S.S. Korsakova 2015; 115(12): 7–18, https://doi.org/10.17116/jnevro20151151127-18.
- Lefaucheur J.P., Aleman A., Baeken C., Benninger D.H., Brunelin J., Di Lazzaro V., Filipović S.R., Grefkes C., Hasan A., Hummel F.C., Jääskeläinen S.K., Langguth B., Leocani L., Londero A., Nardone R., Nguyen J.P., Nyffeler T., Oliveira-Maia A.J., Oliviero A., Padberg F., Palm U., Paulus W., Poulet E., Quartarone A., Rachid F., Rektorová I., Rossi S., Sahlsten H., Schecklmann M., Szekely D., Ziemann U. Evidence-based guidelines on the therapeutic use of repetitive transcranial magnetic stimulation (rTMS): an update (2014–2018). Clin Neurophysiol 2020; 131(2): 474–528, https://doi.org/10.1016/j.clinph.2019.11.002.
- Matsuura A., Onoda K., Oguro H., Yamaguchi S. Magnetic stimulation and movement-related cortical activity for acute stroke with hemiparesis. Eur J Neurol 2015; 22(12): 1526–1532, https://doi.org/10.1111/ene.12776.
- Du J., Tian L., Liu W., Hu J., Xu G., Ma M., Fan X., Ye R., Jiang Y., Yin Q., Zhu W., Xiong Y., Yang F., Liu X. Effects of repetitive transcranial magnetic stimulation on motor recovery and motor cortex excitability in patients with stroke: a randomized controlled trial. Eur J Neurol 2016; 23(11): 1666–1672, https://doi.org/10.1111/ene.13105.
- Kim W.S., Kwon B.S., Seo H.G., Park J., Paik N.J. Low-frequency repetitive transcranial magnetic stimulation over contralesional motor cortex for motor recovery in subacute ischemic stroke: a randomized sham-controlled trial. Neurorehabil Neural Repair 2020; 34(9): 856–867, https://doi.org/10.1177/1545968320948610.
- Huang Y.Z., Lin L.F., Chang K.H., Hu C.J., Liou T.H., Lin Y.N. Priming with 1-Hz repetitive transcranial magnetic stimulation over contralesional leg motor cortex does not increase the rate of regaining ambulation within 3 months of stroke: a randomized controlled trial. Am J Phys Med Rehabil 2018; 97(5): 339–345, https://doi.org/10.1097/phm.0000000000000850.
- Kim J., Yim J. Effects of high-frequency repetitive transcranial magnetic stimulation combined with task-oriented mirror therapy training on hand rehabilitation of acute stroke patients. Med Sci Monit 2018; 24: 743–750, https://doi.org/10.12659/msm.905636.
- Guan Y.Z., Li J., Zhang X.W., Wu S., Du H., Cui L.Y., Zhang W.H. Effectiveness of repetitive transcranial magnetic stimulation (rTMS) after acute stroke: a one-year longitudinal randomized trial. CNS Neurosci Ther 2017; 23(12): 940–946, https://doi.org/10.1111/cns.12762.
- Tang Z., Han K., Wang R., Zhang Y., Zhang H. Excitatory repetitive transcranial magnetic stimulation over the ipsilesional hemisphere for upper limb motor function after stroke: a systematic review and meta-analysis. Front Neurol 2022; 13: 918597, https://doi.org/10.3389/fneur.2022.918597.
- Long H., Wang H., Zhao C., Duan Q., Feng F., Hui N., Mao L., Liu H., Mou X., Yuan H. Effects of combining high- and low-frequency repetitive transcranial magnetic stimulation on upper limb hemiparesis in the early phase of stroke. Restor Neurol Neurosci 2018; 36(1): 21–30, https://doi.org/10.3233/rnn-170733.
- Bajaj S., Housley S.N., Wu D., Dhamala M., James G.A., Butler A.J. Dominance of the unaffected hemisphere motor network and its role in the behavior of chronic stroke survivors. Front Hum Neurosci 2016; 10: 650, https://doi.org/10.3389/fnhum.2016.00650.
- McCambridge A.B., Stinear J.W., Byblow W.D. Revisiting interhemispheric imbalance in chronic stroke: a tDCS study. Clin Neurophysiol 2018; 129(1): 42–50, https://doi.org/10.1016/j.clinph.2017.10.016.
- Salehi Dehno N., Kamali F., Shariat A., Jaberzadeh S. Comparison of transcallosal inhibition between hemispheres and its relationship with motor behavior in patients with severe upper extremity impairment after subacute stroke. J Stroke Cerebrovasc Dis 2022; 31(6): 106469, https://doi.org/10.1016/j.jstrokecerebrovasdis.2022.106469.
- Wang Q., Zhang D., Zhao Y.Y., Hai H., Ma Y.W. Effects of high-frequency repetitive transcranial magnetic stimulation over the contralesional motor cortex on motor recovery in severe hemiplegic stroke: a randomized clinical trial. Brain Stimul 2020; 13(4): 979–986, https://doi.org/10.1016/j.brs.2020.03.020.
- Huang Y.Z., Edwards M.J., Rounis E., Bhatia K.P., Rothwell J.C. Theta burst stimulation of the human motor cortex. Neuron 2005; 45(2): 201–206, https://doi.org/10.1016/j.neuron.2004.12.033.
- Goldsworthy M.R., Pitcher J.B., Ridding M.C. The application of spaced theta burst protocols induces long-lasting neuroplastic changes in the human motor cortex. Eur J Neurosci 2012; 35(1): 125–134, https://doi.org/10.1111/j.1460-9568.2011.07924.x.
- Di Lazzaro V., Pilato F., Dileone M., Profice P., Oliviero A., Mazzone P., Insola A., Ranieri F., Meglio M., Tonali P.A., Rothwell J.C. The physiological basis of the effects of intermittent theta burst stimulation of the human motor cortex. J Physiol 2008; 586(16): 3871–3879, https://doi.org/10.1113/jphysiol.2008.152736.
- Nicolo P., Magnin C., Pedrazzini E., Plomp G., Mottaz A., Schnider A., Guggisberg A.G. Comparison of neuroplastic responses to cathodal transcranial direct current stimulation and continuous theta burst stimulation in subacute stroke. Arch Phys Med Rehabil 2018; 99(5): 862–872.e1, https://doi.org/10.1016/j.apmr.2017.10.026.
- Volz L.J., Rehme A.K., Michely J., Nettekoven C., Eickhoff S.B., Fink G.R., Grefkes C. Shaping early reorganization of neural networks promotes motor function after stroke. Cereb Cortex 2016; 26(6): 2882–2894, https://doi.org/10.1093/cercor/bhw034.
- Lin L.F., Chang K.H., Huang Y.Z., Lai C.H., Liou T.H., Lin Y.N. Simultaneous stimulation in bilateral leg motor areas with intermittent theta burst stimulation to improve functional performance after stroke: a feasibility pilot study. Eur J Phys Rehabil Med 2019; 55(2): 162–168, https://doi.org/10.23736/s1973-9087.18.05245-0.
- Meng Y., Zhang D., Hai H., Zhao Y.Y., Ma Y.W. Efficacy of coupling intermittent theta-burst stimulation and 1 Hz repetitive transcranial magnetic stimulation to enhance upper limb motor recovery in subacute stroke patients: a randomized controlled trial. Restor Neurol Neurosci 2020; 38(1): 109–118, https://doi.org/10.3233/rnn-190953.
- Nyffeler T., Vanbellingen T., Kaufmann B.C., Pflugshaupt T., Bauer D., Frey J., Chechlacz M., Bohlhalter S., Müri R.M., Nef T., Cazzoli D. Theta burst stimulation in neglect after stroke: functional outcome and response variability origins. Brain 2019; 142(4): 992–1008, https://doi.org/10.1093/brain/awz029.
- Paulus W. Transcranial electrical stimulation (tES – tDCS; tRNS, tACS) methods. Neuropsychol Rehabil 2011; 21(5): 602–617, https://doi.org/10.1080/09602011.2011.557292.
- Klomjai W., Lackmy-Vallée A., Roche N., Pradat-Diehl P., Marchand-Pauvert V., Katz R. Repetitive transcranial magnetic stimulation and transcranial direct current stimulation in motor rehabilitation after stroke: an update. Ann Phys Rehabil Med 2015; 58(4): 220–224, https://doi.org/10.1016/j.rehab.2015.05.006.
- Nitsche M.A., Paulus W. Excitability changes induced in the human motor cortex by weak transcranial direct current stimulation. J Physiol 2000; 527 Pt 3(Pt 3): 633–639, https://doi.org/10.1111/j.1469-7793.2000.t01-1-00633.x.
- Nitsche M.A., Fricke K., Henschke U., Schlitterlau A., Liebetanz D., Lang N., Henning S., Tergau F., Paulus W. Pharmacological modulation of cortical excitability shifts induced by transcranial direct current stimulation in humans. J Physiol 2003; 553(Pt 1): 293–301, https://doi.org/10.1113/jphysiol.2003.049916.
- Chang M.C., Kim D.Y., Park D.H. Enhancement of cortical excitability and lower limb motor function in patients with stroke by transcranial direct current stimulation. Brain Stimul 2015; 8(3): 561–566, https://doi.org/10.1016/j.brs.2015.01.411.
- Bornheim S., Croisier J.L., Maquet P., Kaux J.F. Transcranial direct current stimulation associated with physical-therapy in acute stroke patients — a randomized, triple blind, sham-controlled study. Brain Stimul 2020; 13(2): 329–336, https://doi.org/10.1016/j.brs.2019.10.019.
- Boasquevisque D.D.S., Servinsckins L., de Paiva J.P.Q., Dos Santos D.G., Soares P., Pires D.S., Meltzer J.A., Plow E.B., de Freitas P.F., Speciali D.S., Lopes P., Peres M.F.P., Silva G.S., Lacerda S., Conforto A.B. Contralesional cathodal transcranial direct current stimulation does not enhance upper limb function in subacute stroke: a pilot randomized clinical trial. Neural Plast 2021; 2021: 8858394, https://doi.org/10.1155/2021/8858394.
- Klomjai W., Aneksan B., Pheungphrarattanatrai A., Chantanachai T., Choowong N., Bunleukhet S., Auvichayapat P., Nilanon Y., Hiengkaew V. Effect of single-session dual-tDCS before physical therapy on lower-limb performance in sub-acute stroke patients: a randomized sham-controlled crossover study. Ann Phys Rehabil Med 2018; 61(5): 286–291, https://doi.org/10.1016/j.rehab.2018.04.005.
- Lefaucheur J.P., Antal A., Ayache S.S., Benninger D.H., Brunelin J., Cogiamanian F., Cotelli M., De Ridder D., Ferrucci R., Langguth B., Marangolo P., Mylius V., Nitsche M.A., Padberg F., Palm U., Poulet E., Priori A., Rossi S., Schecklmann M., Vanneste S., Ziemann U., Garcia-Larrea L., Paulus W. Evidence-based guidelines on the therapeutic use of transcranial direct current stimulation (tDCS). Clin Neurophysiol 2017; 128(1): 56–92, https://doi.org/10.1016/j.clinph.2016.10.087.
- Hesse S., Waldner A., Mehrholz J., Tomelleri C., Pohl M., Werner C. Combined transcranial direct current stimulation and robot-assisted arm training in subacute stroke patients: an exploratory, randomized multicenter trial. Neurorehabil Neural Repair 2011; 25(9): 838–846, https://doi.org/10.1177/1545968311413906.
- Achacheluee S.T., Rahnama L., Karimi N., Abdollahi I., Arslan S.A., Jaberzadeh S. The effect of unihemispheric concurrent dual-site transcranial direct current stimulation of primary motor and dorsolateral prefrontal cortices on motor function in patients with sub-acute stroke. Front Hum Neurosci 2018; 12: 441, https://doi.org/10.3389/fnhum.2018.00441.
- Chen J.L., Schipani A., Schuch C.P., Lam H., Swardfager W., Thiel A., Edwards J.D. Does cathodal vs. sham transcranial direct current stimulation over contralesional motor cortex enhance upper limb motor recovery post-stroke? A systematic review and meta-analysis. Front Neurol 2021; 12: 626021, https://doi.org/10.3389/fneur.2021.626021.
- Elsner B., Kwakkel G., Kugler J., Mehrholz J. Transcranial direct current stimulation (tDCS) for improving capacity in activities and arm function after stroke: a network meta-analysis of randomised controlled trials. J Neuroeng Rehabil 2017; 14(1): 95, https://doi.org/10.1186/s12984-017-0301-7.
- Van Hoornweder S., Vanderzande L., Bloemers E., Verstraelen S., Depestele S., Cuypers K., Dun K.V., Strouwen C., Meesen R. The effects of transcranial direct current stimulation on upper-limb function post-stroke: a meta-analysis of multiple-session studies. Clin Neurophysiol 2021; 132(8): 1897–1918, https://doi.org/10.1016/j.clinph.2021.05.015.
- Chow A.D., Shin J., Wang H., Kellawan J.M., Pereira H.M. Influence of transcranial direct current stimulation dosage and associated therapy on motor recovery post-stroke: a systematic review and meta-analysis. Front Aging Neurosci 2022; 14: 821915, https://doi.org/10.3389/fnagi.2022.821915.
- Wong P.L., Yang Y.R., Tang S.C., Huang S.F., Wang R.Y. Comparing different montages of transcranial direct current stimulation on dual-task walking and cortical activity in chronic stroke: double-blinded randomized controlled trial. BMC Neurol 2022; 22(1): 119, https://doi.org/10.1186/s12883-022-02644-y.
- Navarro-López V., Del Valle-Gratacós M., Fernández-Matías R., Carratalá-Tejada M., Cuesta-Gómez A., Molina-Rueda F. The long-term maintenance of upper limb motor improvements following transcranial direct current stimulation combined with rehabilitation in people with stroke: a systematic review of randomized sham-controlled trials. Sensors (Basel) 2021; 21(15): 5216, https://doi.org/10.3390/s21155216.
- Fitzgerald P.B., Brown T.L., Daskalakis Z.J., Chen R., Kulkarni J. Intensity-dependent effects of 1 Hz rTMS on human corticospinal excitability. Clin Neurophysiol 2002; 113(7): 1136–1141, https://doi.org/10.1016/s1388-2457(02)00145-1.
- Miclaus R., Roman N., Caloian S., Mitoiu B., Suciu O., Onofrei R.R., Pavel E., Neculau A. Non-immersive virtual reality for post-stroke upper extremity rehabilitation: a small cohort randomized trial. Brain Sci 2020; 10(9): 655, https://doi.org/10.3390/brainsci10090655.
- Zrenner C., Desideri D., Belardinelli P., Ziemann U. Real-time EEG-defined excitability states determine efficacy of TMS-induced plasticity in human motor cortex. Brain Stimul 2018; 11(2): 374–389, https://doi.org/10.1016/j.brs.2017.11.016.
- Pineda J.A. The functional significance of mu rhythms: translating “seeing” and “hearing” into “doing”. Brain Res Rev 2005; 50(1): 57–68, https://doi.org/10.1016/j.brainresrev.2005.04.005.
- Baur D., Galevska D., Hussain S., Cohen L.G., Ziemann U., Zrenner C. Induction of LTD-like corticospinal plasticity by low-frequency rTMS depends on pre-stimulus phase of sensorimotor μ-rhythm. Brain Stimul 2020; 13(6): 1580–1587, https://doi.org/10.1016/j.brs.2020.09.005.
- Lee J., Lee A., Kim H., Shin M., Yun S.M., Jung Y., Chang W.H., Kim Y.H. Different brain connectivity between responders and nonresponders to dual-mode noninvasive brain stimulation over bilateral primary motor cortices in stroke patients. Neural Plast 2019; 2019: 3826495, https://doi.org/10.1155/2019/3826495.
- Pineda J.A. Sensorimotor cortex as a critical component of an 'extended' mirror neuron system: does it solve the development, correspondence, and control problems in mirroring? Behav Brain Funct 2008; 4: 47, https://doi.org/10.1186/1744-9081-4-47.
- Marshall P.J., Meltzoff A.N. Neural mirroring systems: exploring the EEG μ rhythm in human infancy. Dev Cogn Neurosci 2011; 1(2): 110–123, https://doi.org/10.1016/j.dcn.2010.09.001.
- Filimon F., Rieth C.A., Sereno M.I., Cottrell G.W. Observed, executed, and imagined action representations can be decoded from ventral and dorsal areas. Cereb Cortex 2015; 25(9): 3144–3158, https://doi.org/10.1093/cercor/bhu110.
- Bajaj S., Butler A.J., Drake D., Dhamala M. Brain effective connectivity during motor-imagery and execution following stroke and rehabilitation. Neuroimage Clin 2015; 8: 572–582, https://doi.org/10.1016/j.nicl.2015.06.006.
- Hardwick R.M., Caspers S., Eickhoff S.B., Swinnen S.P. Neural correlates of action: comparing meta-analyses of imagery, observation, and execution. Neurosci Biobehav Rev 2018; 94: 31–44, https://doi.org/10.1016/j.neubiorev.2018.08.003.
- Levin M.F., Weiss P.L., Keshner E.A. Emergence of virtual reality as a tool for upper limb rehabilitation: incorporation of motor control and motor learning principles. Phys Ther 2015; 95(3): 415–425, https://doi.org/10.2522/ptj.20130579.
- Mekbib D.B., Zhao Z., Wang J., Xu B., Zhang L., Cheng R., Fang S., Shao Y., Yang W., Han J., Jiang H., Zhu J., Ye X., Zhang J., Xu D. Proactive motor functional recovery following immersive virtual reality-based limb mirroring therapy in patients with subacute stroke. Neurotherapeutics 2020; 17(4): 1919–1930, https://doi.org/10.1007/s13311-020-00882-x.
- Xiao X., Lin Q., Lo W.L., Mao Y.R., Shi X.C., Cates R.S., Zhou S.F., Huang D.F., Li L. Cerebral reorganization in subacute stroke survivors after virtual reality-based training: a preliminary study. Behav Neurol 2017; 2017: 6261479, https://doi.org/10.1155/2017/6261479.
- James G.A., Lu Z.L., VanMeter J.W., Sathian K., Hu X.P., Butler A.J. Changes in resting state effective connectivity in the motor network following rehabilitation of upper extremity poststroke paresis. Top Stroke Rehabil 2009; 16(4): 270–281, https://doi.org/10.1310/tsr1604-270.
- Enzinger C., Dawes H., Johansen-Berg H., Wade D., Bogdanovic M., Collett J., Guy C., Kischka U., Ropele S., Fazekas F., Matthews P.M. Brain activity changes associated with treadmill training after stroke. Stroke 2009; 40(7): 2460–2467, https://doi.org/10.1161/strokeaha.109.550053.
- Ögün M.N., Kurul R., Yaşar M.F., Turkoglu S.A., Avci Ş., Yildiz N. Effect of leap motion-based 3D immersive virtual reality usage on upper extremity function in ischemic stroke patients. Arq Neuropsiquiatr 2019; 77(10): 681–688, https://doi.org/10.1590/0004-282x20190129.
- Maier M., Rubio Ballester B., Duff A., Duarte Oller E., Verschure P.F.M.J. Effect of specific over nonspecific VR-based rehabilitation on poststroke motor recovery: a systematic meta-analysis. Neurorehabil Neural Repair 2019; 33(2): 112–129, https://doi.org/10.1177/1545968318820169.
- Türkbey T.A., Kutlay S., Gök H. Clinical feasibility of Xbox KinectTM training for stroke rehabilitation: a single-blind randomized controlled pilot study. J Rehabil Med 2017; 49(1): 22–29, https://doi.org/10.2340/16501977-2183.
- Cano-Mañas M.J., Collado-Vázquez S., Rodríguez Hernández J., Muñoz Villena A.J., Cano-de-la-Cuerda R. Effects of video-game based therapy on balance, postural control, functionality, and quality of life of patients with subacute stroke: a randomized controlled trial. J Healthc Eng 2020; 2020: 5480315, https://doi.org/10.1155/2020/5480315.
- Saposnik G., Cohen L.G., Mamdani M., Pooyania S., Ploughman M., Cheung D., Shaw J., Hall J., Nord P., Dukelow S., Nilanont Y., De Los Rios F., Olmos L., Levin M., Teasell R., Cohen A., Thorpe K., Laupacis A., Bayley M.; Stroke Outcomes Research Canada. Efficacy and safety of non-immersive virtual reality exercising in stroke rehabilitation (EVREST): a randomised, multicentre, single-blind, controlled trial. Lancet Neurol 2016; 15(10): 1019–1027, https://doi.org/10.1016/s1474-4422(16)30121-1.
- Choi J.H., Han E.Y., Kim B.R., Kim S.M., Im S.H., Lee S.Y., Hyun C.W. Effectiveness of commercial gaming-based virtual reality movement therapy on functional recovery of upper extremity in subacute stroke patients. Ann Rehabil Med 2014; 38(4): 485–93, https://doi.org/10.5535/arm.2014.38.4.485.
- Cannell J., Jovic E., Rathjen A., Lane K., Tyson A.M., Callisaya M.L., Smith S.T., Ahuja K.D., Bird M.L. The efficacy of interactive, motion capture-based rehabilitation on functional outcomes in an inpatient stroke population: a randomized controlled trial. Clin Rehabil 2018; 32(2): 191–200, https://doi.org/10.1177/0269215517720790.
- Kim W.S., Cho S., Park S.H., Lee J.Y., Kwon S., Paik N.J. A low cost Kinect-based virtual rehabilitation system for inpatient rehabilitation of the upper limb in patients with subacute stroke: a randomized, double-blind, sham-controlled pilot trial. Medicine (Baltimore) 2018; 97(25): e11173, https://doi.org/10.1097/md.0000000000011173.
- Kim W.S., Cho S., Ku J., Kim Y., Lee K., Hwang H.J., Paik N.J. Clinical application of virtual reality for upper limb motor rehabilitation in stroke: review of technologies and clinical evidence. J Clin Med 2020; 9(10): 3369, https://doi.org/10.3390/jcm9103369.
- Brunner I., Skouen J.S., Hofstad H., Aßmus J., Becker F., Sanders A.M., Pallesen H., Qvist Kristensen L., Michielsen M., Thijs L., Verheyden G. Virtual reality training for upper extremity in subacute stroke (VIRTUES): a multicenter RCT. Neurology 2017; 89(24): 2413–2421, https://doi.org/10.1212/wnl.0000000000004744.
- de Rooij I.J.M., van de Port I.G.L., Punt M., Abbink-van Moorsel P.J.M., Kortsmit M., van Eijk R.P.A., Visser-Meily J.M.A., Meijer J.G. Effect of virtual reality gait training on participation in survivors of subacute stroke: a randomized controlled trial. Phys Ther 2021; 101(5): pzab051, https://doi.org/10.1093/ptj/pzab051.
- Xie H., Zhang H., Liang H., Fan H., Zhou J., Lo W.L.A., Li L. A novel glasses-free virtual reality rehabilitation system on improving upper limb motor function among patients with stroke: a feasibility pilot study. Med Nov Technol Devices 2021; 11: 100069, https://doi.org/10.1016/j.medntd.2021.100069.
- Kiper P., Szczudlik A., Agostini M., Opara J., Nowobilski R., Ventura L., Tonin P., Turolla A. Virtual reality for upper limb rehabilitation in subacute and chronic stroke: a randomized controlled trial. Arch Phys Med Rehabil 2018; 99(5): 834–842.e4, https://doi.org/10.1016/j.apmr.2018.01.023.
- Rogers J.M., Duckworth J., Middleton S., Steenbergen B., Wilson P.H. Elements virtual rehabilitation improves motor, cognitive, and functional outcomes in adult stroke: evidence from a randomized controlled pilot study. J Neuroeng Rehabil 2019; 16(1): 56, https://doi.org/10.1186/s12984-019-0531-y.
- Rodríguez-Hernández M., Criado-Álvarez J.J., Corregidor-Sánchez A.I., Martín-Conty J.L., Mohedano-Moriano A., Polonio-López B. Effects of virtual reality-based therapy on quality of life of patients with subacute stroke: a three-month follow-up randomized controlled trial. Int J Environ Res Public Health 2021; 18(6): 2810, https://doi.org/10.3390/ijerph18062810.
- Graimann B., Allison B., Pfurtscheller G. Brain–computer interfaces: a gentle introduction. The Frontiers Collection. Springer-Verlag Berlin Heidelberg; 2010; p. 1–27, https://doi.org/10.1007/978-3-642-02091-9_1.
- Frolov A.A., Mokienko O., Lyukmanov R., Biryukova E., Kotov S., Turbina L., Nadareyshvily G., Bushkova Y. Post-stroke rehabilitation training with a motor-imagery-based brain-computer interface (BCI)-controlled hand exoskeleton: a randomized controlled multicenter trial. Front Neurosci 2017; 11: 400, https://doi.org/10.3389/fnins.2017.00400.
- Su F., Xu W. Enhancing brain plasticity to promote stroke recovery. Front Neurol 2020; 11: 554089, https://doi.org/10.3389/fneur.2020.554089.
- Liang W.D., Xu Y., Schmidt J., Zhang L.X., Ruddy K.L. Upregulating excitability of corticospinal pathways in stroke patients using TMS neurofeedback; a pilot study. Neuroimage Clin 2020; 28: 102465, https://doi.org/10.1016/j.nicl.2020.102465.
- Sebastián-Romagosa M., Cho W., Ortner R., Murovec N., Von Oertzen T., Kamada K., Allison B.Z., Guger C. Brain computer interface treatment for motor rehabilitation of upper extremity of stroke patients — a feasibility study. Front Neurosci 2020; 14: 591435, https://doi.org/10.3389/fnins.2020.591435.
- Wu Q., Yue Z., Ge Y., Ma D., Yin H., Zhao H., Liu G., Wang J., Dou W., Pan Y. Brain functional networks study of subacute stroke patients with upper limb dysfunction after comprehensive rehabilitation including BCI training. Front Neurol 2020; 10: 1419, https://doi.org/10.3389/fneur.2019.01419.
- Biasiucci A., Leeb R., Iturrate I., Perdikis S., Al-Khodairy A., Corbet T., Schnider A., Schmidlin T., Zhang H., Bassolino M., Viceic D., Vuadens P., Guggisberg A.G., Millán J.D.R. Brain-actuated functional electrical stimulation elicits lasting arm motor recovery after stroke. Nat Commun 2018; 9(1): 2421, https://doi.org/10.1038/s41467-018-04673-z.
- Bhagat N.A., Yozbatiran N., Sullivan J.L., Paranjape R., Losey C., Hernandez Z., Keser Z., Grossman R., Francisco G.E., O'Malley M.K., Contreras-Vidal J.L. Neural activity modulations and motor recovery following brain-exoskeleton interface mediated stroke rehabilitation. Neuroimage Clin 2020; 28: 102502, https://doi.org/10.1016/j.nicl.2020.102502.
- Kumari R., Janković M.M., Costa A., Savić A.M., Konstantinović L., Djordjević O., Vucković A. Short term priming effect of brain-actuated muscle stimulation using bimanual movements in stroke. Clin Neurophysiol 2022; 138: 108–121, https://doi.org/10.1016/j.clinph.2022.03.002.
- Ramos-Murguialday A., Curado M.R., Broetz D., Yilmaz Ö., Brasil F.L., Liberati G., Garcia-Cossio E., Cho W., Caria A., Cohen L.G., Birbaumer N. Brain–machine interface in chronic stroke: randomized trial long-term follow-up. Neurorehabil Neural Repair 2019; 33(3): 188–198, https://doi.org/10.1177/1545968319827573.
- Hashimoto Y., Kakui T., Ushiba J., Liu M., Kamada K., Ota T. Portable rehabilitation system with brain-computer interface for inpatients with acute and subacute stroke: a feasibility study. Assist Technol 2022; 34(4): 402–410, https://doi.org/10.1080/10400435.2020.1836067.
- Zhao C.G., Ju F., Sun W., Jiang S., Xi X., Wang H., Sun X.L., Li M., Xie J., Zhang K., Xu G.H., Zhang S.C., Mou X., Yuan H. Effects of training with a brain-computer interface-controlled robot on rehabilitation outcome in patients with subacute stroke: a randomized controlled trial. Neurol Ther 2022; 11(2): 679–695, https://doi.org/10.1007/s40120-022-00333-z.