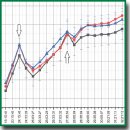
Effect of the Peptide Calcium Channel Blocker ω-hexatoxin-Hv1a on Cell Death during Ischemia/Reperfusion in vitro
Apoptosis and necrosis during reperfusion after ischemia are key mechanisms at the cellular level leading to damage. The development of pathological conditions is preceded by intracellular calcium ion overload both at the stage of ischemia and at the stage of reperfusion. In this regard, one of the strategies aimed at reducing damage during ischemia/reperfusion is associated with the use of calcium channel blockers.
The aim of the study was to study the effect of a peptide toxin, a calcium channel blocker ω-hexatoxin-Hv1a, on different types of epithelial cell death during in vitro reconstruction of ischemia/reperfusion conditions characteristic of organ transplantation.
Materials and Methods. In this study, we used CHO-K1 epithelial cell culture. Changes in apoptosis, necrosis, cell index, and calcium ion concentration were assessed when modeling ischemia/reperfusion processes in vitro with the addition of a calcium channel blocker toxin. Ischemic and reperfusion injury was achieved by oxygen and nutrient deprivation followed by reperfusion in a complete nutrient medium. The measurements were performed using a multimodal plate reader-fluorimeter.
Results. An increase in apoptosis, necrosis, and the concentration of calcium ions was recorded when modeling ischemia/reperfusion processes. A decrease in the level of apoptosis and necrosis, as well as the concentration of calcium ions to a physiological level or a level close to physiological, was noted when the toxin was added at a concentration of 50 nM at the reperfusion stage. The cell index showed a faster restoration in the presence of the toxin.
Conclusion. The experimental data confirm the hypothesis of a beneficial effect of peptide calcium channel blockers on the state of epithelial cells during reperfusion after ischemia and can be considered for further study as a strategy for organ adaptation before reperfusion.
- Simonis G., Strasser R.H., Ebner B. Reperfusion injury in acute myocardial infarction. Crit Care 2012; 16(2): A22, https://doi.org/10.1186/cc11280.
- Heusch G. Myocardial ischaemia–reperfusion injury and cardioprotection in perspective. Nat Rev Cardiol 2020; 17(22): 773–789, https://doi.org/10.1038/s41569-020-0403-y.
- Guo Z.H., Li F., Wang W.Z. The mechanisms of brain ischemic insult and potential protective interventions. Neurosci Bull 2009; 25(3): 139–152, https://doi.org/10.1007/s12264-009-0104-3.
- Al-Mufti F., Amuluru K., Roth W., Nuoman R., El-Ghanem M., Meyers P.M. Cerebral ischemic reperfusion injury following recanalization of large vessel occlusions. Neurosurgery 2018; 82(6): 781–789, https://doi.org/10.1093/neuros/nyx341.
- Bonventre J.V., Yang L. Cellular pathophysiology of ischemic acute kidney injury. J Clin Invest 2011; 121(11): 4210–4221, https://doi.org/10.1172/jci45161.
- Hesketh E.E., Czopek A., Clay M., Borthwick G., Ferenbach D., Kluth D., Highes J. Renal ischaemia reperfusion injury: a mouse model of injury and regeneration. J Vis Exp 2014; 88: 51816, https://doi.org/10.3791/51816.
- Jassem W., Fuggle S.V., Rela M., Koo D.D.H., Heaton N.D. The role of mitochondria in ischemia/reperfusion injury. Transplantation 2002; 73(4): 493–499, https://doi.org/10.1097/00007890-200202270-00001.
- Dar W.A., Sullivan E., Bynon J.S., Eltzschig H., Ju C. Ischaemia reperfusion injury in liver transplantation: cellular and molecular mechanisms. Liver Int 2019; 39(5): 788–801, https://doi.org/10.1111/liv.14091.
- Salvadori M., Rosso G., Bertoni E. Update on ischemia-reperfusion injury in kidney transplantation: pathogenesis and treatment. World J Transplant 2015; 5(2): 52–67, https://doi.org/10.5500/wjt.v5.i2.52.
- Ponticelli C. Ischaemia-reperfusion injury: a major protagonist in kidney transplantation. Nephrol Dial Transplant 2014; 29(6): 1134–1140, https://doi.org/10.1093/ndt/gfu244.
- Smith S.F., Hosgood S.A., Nicholson M.L. Ischemia-reperfusion injury in renal transplantation: 3 key signaling pathways in tubular epithelial cells. Kidney Int 2019; 95(1): 50–56, https://doi.org/10.1016/j.kint.2018.10.009.
- Saren G., Wong A., Lu Y.B., Baciu C., Zhou W., Zamel R., Soltanieh S., Sugihara J., Liu M. Ischemia-reperfusion injury in a simulated lung transplant setting differentially regulates transcriptomic profiles between human lung endothelial and epithelial cells. Cells 2021; 10(10): 2713, https://doi.org/10.3390/cells10102713.
- Saat T.C., van den Akker E.K., IJzermans J.N.M., Dor F.J.M.F., de Bruin R.W.F. Improving the outcome of kidney transplantation by ameliorating renal ischemia reperfusion injury: lost in translation? J Transl Med 2016; 14: 20, https://doi.org/10.1186/s12967-016-0767-2.
- Zhao H., Alam A., Soo A.P., George A.J.T., Ma D. Ischemia-reperfusion injury reduces long term renal graft survival: mechanism and beyond. EBioMedicine 2018; 28: 31–42, https://doi.org/10.1016/j.ebiom.2018.01.025.
- Capuzzimati M., Hough O., Liu M. Cell death and ischemia-reperfusion injury in lung transplantation. J Heart Lung Transplant 2022; 41(8): 1003–1013, https://doi.org/10.1016/j.healun.2022.05.013.
- Thomas F., Wu J., Thomas J.M. Apoptosis and organ transplantation. Curr Opin Organ Transplant 2000; 5(1): 35–41, https://doi.org/10.1097/00075200-200003000-00007.
- Bulent G. Ischemia reperfusion injury in kidney transplantation. In: Kidney transplantation — new perspectives. Trzcinska M. (editor). IntechOpen; 2011; p. 213–222, https://doi.org/10.5772/18289.
- Kosieradzki M., Rowiński W. Ischemia/reperfusion injury in kidney transplantation: mechanisms and prevention. Transplant Proc 2008; 40(10): 3279–3288, https://doi.org/10.1016/j.transproceed.2008.10.004.
- Inserte J., Hernando V., Garcia-Dorado D. Contribution of calpains to myocardial ischaemia/reperfusion injury. Cardiovasc Res 2012; 96(1): 23–31, https://doi.org/10.1093/cvr/cvs232.
- Peng T.I., Jou M.J. Oxidative stress caused by mitochondrial calcium overload. Ann N Y Acad Sci 2010; 1201: 183–188, https://doi.org/10.1111/j.1749-6632.2010.05634.x.
- Li C., Jackson R.M. Reactive species mechanisms of cellular hypoxia-reoxygenation injury. Am J Physiol Cell Physiol 2002; 282(2): C227–C241, https://doi.org/10.1152/ajpcell.00112.2001.
- Tang S.P., Mao X.L., Chen Y.H., Yan L.L., Ye L.P., Li S.W. Reactive oxygen species induce fatty liver and ischemia-reperfusion injury by promoting inflammation and cell death. Front Immunol 2022; 13: 870239, https://doi.org/10.3389/fimmu.2022.870239.
- Kalogeris T., Baines C.P., Krenz M., Korthuis R.J. Cell biology of ischemia/reperfusion injury. Int Rev Cell Mol Biol 2012; 298: 229–317, https://doi.org/10.1016/b978-0-12-394309-5.00006-7.
- Philipponnet C., Aniort J., Garrouste C., Kemeny J.L., Heng A.E. Ischemia reperfusion injury in kidney transplantation: a case report. Medicine (Baltimore) 2018; 97(52): e13650, https://doi.org/10.1097/md.0000000000013650.
- Radajewska A., Krzywonos-Zawadzka A., Bil-Luli I. Recent methods of kidney storage and therapeutic possibilities of transplant kidney. Biomedicines 2022; 10(5): 1013, https://doi.org/10.3390/biomedicines10051013.
- Sahara H., Yamada K. Medical gas (carbon monoxide, hydrogen sulfide) therapy prolongs survival of the fully MHC-disparate lung graft from brain-dead donors in miniature swine. J Heart Lung Transplant 2014; 33(4): S49–S50, https://doi.org/10.1016/j.healun.2014.01.164.
- Bernhardt W.M., Gottmann U., Doyon F., Buchholz B., Campean V., Schödel J., Reisenbuechler A., Klaus S., Arend M., Flippin L., Willam C., Wiesener M.S., Yard B., Warnecke C., Eckardt K.U. Donor treatment with a PHD-inhibitor activating HIFs prevents graft injury and prolongs survival in an allogenic kidney transplant model. Proc Natl Acad Sci U S A 2009; 106(50): 21276–21281, https://doi.org/10.1073/pnas.0903978106.
- Baldari S., Di Rocco G., Trivisonno A., Samengo D., Pani G., Toietta G. Promotion of survival and engraftment of transplanted adipose tissue-derived stromal and vascular cells by overexpression of manganese superoxide dismutase. Int J Mol Sci 2016; 17(7): 1082, https://doi.org/10.3390/ijms17071082.
- Saber M., Eimani H., Soleimani Mehranjani M., Shahverdi A., Momeni H.R., Fathi R., Tavana S. The effect of Verapamil on ischaemia/reperfusion injury in mouse ovarian tissue transplantation. Biomed Pharmacother 2018; 108: 1313–1319, https://doi.org/10.1016/j.biopha.2018.09.130.
- Fansa I., Altug M.E., Melek I., Ucar E., Kontas T., Akcora B., Atik E., Duman T. The neuroprotective and anti-inflammatory effects of diltiazem in spinal cord ischaemia–reperfusion injury. Int J Med Res 2009; 37(2): 520–533, https://doi.org/10.1177/147323000903700228.
- Bao M., Huang W., Zhao Y., Fang X., Zhang Y., Gao F., Huang D., Wang B., Shi G. Verapamil alleviates myocardial ischemia/reperfusion injury by attenuating oxidative stress via activation of SIRT1. Front Pharmacol 2022; 13: 822640, https://doi.org/10.3389/fphar.2022.822640.
- Søfteland J.M., Oltean M. Intestinal ischemia-reperfusion injury and calcium channel blockers: getting to the core of the problem. J Invest Surg 2021; 34(7): 808–809, https://doi.org/10.1080/08941939.2020.1714823.
- Nawrot D.A., Ozer L.Y., Al Haj Zen A. A novel high content angiogenesis assay reveals that Lacidipine, L-type calcium channel blocker, induces in vitro vascular lumen expansion. Int J Mol Sci 2022; 23(9): 4891, https://doi.org/10.3390/ijms23094891.
- Gao X., Stanger K., Kaluarachchi H., Maurer T., Ciepla P., Chalouni C., Franke Y., Hannoush R.N. Cellular uptake of a cystine-knot peptide and modulation of its intracellular trafficking. Sci Rep 2016; 6: 35179, https://doi.org/10.1038/srep35179.
- Deplazes E. Molecular simulations of disulfide-rich venom peptides with ion channels and membranes. Molecules 2017; 22(3): 362, https://doi.org/10.3390/molecules22030362.
- Funk C.D., Ardakani A. A novel strategy to mitigate the hyperinflammatory response to COVID-19 by targeting leukotrienes. Front Pharmacol 2020; 11: 1214, https://doi.org/10.3389/fphar.2020.01214.
- Bajaj S., Han J. Venom-derived peptide modulators of cation-selective channels: friend, foe or frenemy. Front Pharmacol 2019; 10: 58, https://doi.org/10.3389/fphar.2019.00058.
- Oliveira K.M., Lavor M.S., Silva C.M., Fukushima F.B., Rosado I.R., Silva J.F., Martins B.C., Guimarães L.B., Gomez M.V., Melo M.M., Melo E.G. Omega-conotoxin MVIIC attenuates neuronal apoptosis in vitro and improves significant recovery after spinal cord injury in vivo in rats. Int J Clin Exp Pathol 2014; 7(7): 3524–3536.
- Yamada K., Teraoka T., Morita S., Hasegawa T., Nabeshima T. ω-conotoxin GVIA protects against ischemia-induced neuronal death in the Mongolian gerbil but not against quinolinic acid-induced neurotoxicity in the rat. Neuropharmacology 1994; 33(2): 251–254, https://doi.org/10.1016/0028-3908(94)90016-7.
- Wenger R.H., Kurtcuoglu V., Scholz C.C., Marti H.H., Hoogewijs D. Frequently asked questions in hypoxia research. Hypoxia (Auckl) 2015; 3: 35–43, https://doi.org/10.2147/hp.s92198.
- Gallardo Bolaños J.M., Miró Morán Á., Balao da Silva C.M., Morillo Rodríguez A., Plaza Dávila M., Aparicio I.M., Tapia J.A., Ortega Ferrusola C., Peña F.J. Autophagy and apoptosis have a role in the survival or death of stallion spermatozoa during conservation in refrigeration. PLoS One 2012; 7(1): e30688, https://doi.org/10.1371/journal.pone.0030688.
- Fonteriz R.I., de la Fuente S., Moreno A., Lobatón C.D., Montero M., Alvarez J. Monitoring mitochondrial [Ca2+] dynamics with rhod-2, ratiometric pericam and aequorin. Cell Calcium 2010; 48(1): 61–69, https://doi.org/10.1016/j.ceca.2010.07.001.
- Ke N., Wang X., Xu X., Abassi Y.A. The xCELLigence system for real-time and label-free monitoring of cell viability. Methods Mol Biol 2011; 740: 33–43, https://doi.org/10.1007/978-1-61779-108-6_6.
- Kahn J., Schemmer P. Control of ischemia-reperfusion injury in liver transplantation: potentials for increasing the donor pool. Visc Med 2018; 34(6): 444–448, https://doi.org/10.1159/000493889.
- Wang L., Li J., He S., Liu Y., Chen H., He S., Yin M., Zou D., Chen S., Luo T., Yu X., Wan X., Huang S., Guo Z., He X. Resolving the graft ischemia-reperfusion injury during liver transplantation at the single cell resolution. Cell Death Dis 2021; 12(6): 589, https://doi.org/10.1038/s41419-021-03878-3.
- Iurova E., Beloborodov E., Tazintseva E., Fomin A., Shutov A., Slesarev S., Saenko Y., Saenko Y. Arthropod toxins inhibiting Ca2+ and Na+ channels prevent AC 1001 H3 peptide-induced apoptosis. J Pept Sci 2021; 27(1): e3288, https://doi.org/10.1002/psc.3288.
- Soares R.O.S., Losada D.M., Jordani M.C., Évora P., Castro-e-Silva O. Ischemia/reperfusion injury revisited: an overview of the latest pharmacological strategies. Int J Mol Sci 2019; 20(20): 5034, https://doi.org/10.3390/ijms20205034.