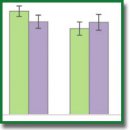
Application of Magnetic Particles for Fast Determination of Immunoreactive Fraction of 68Ga-Labelled VHH Antibodies to PD-L1
Quantification of the immunoreactive fraction (IRF) of radioactive isotope-labeled antibodies or their fragments is necessary to assess the specific activity of radiopharmaceuticals. Traditionally, cells expressing the target molecules on their surface are used to determine IRF, but such analysis is time-consuming and has difficulties with standardization.
The aim of the study was to develop a fast and reliable method for quantitative determination of IRF by 68Ga-labeled VHH antibodies to PD-L1 based on the use of magnetic particles coated with antigen molecules.
Materials and Methods. Commercially available magnetic particles coated with protein A have been used in our study. The antigen conjugated with the Fc fragment (PD-L1-Fc) was immobilized on the particles. The IRF value of 68Ga radionuclide-labeled nanobodies (VHH) against PD-L1 (68Ga-VHH-PD-L1) was determined using magnetic particles coated with antigen molecules and cells expressing the antigen on their surface. When VHH antibodies were conjugated to 68Ga radionuclide, protein molecules were modified using bifunctional chelating agents: tetraazacyclododecanetetraacetic acid (DOTA) or deferoxamine (DFO). The magnitude of IRF was defined as the ratio of radioactivity specifically bound to particles or cells to the total radioactivity added to the sample.
Results. The specificity of the 68Ga-VHH-PD-L1 radioimmunoconjugate binding to the antigen-coated magnetic particles has been proved. Some special aspects, which should be taken into consideration when using this method, have been established. The comparison of the IRF estimates using the antigen-expressing cells and magnetic particles has not revealed any significant differences in the results obtained in our study. Nevertheless, the presented method based on magnetic particles with immobilized antigen molecules requires only 15 min to determine the radioimmunoconjugate IRF, which is of fundamental importance for the routine assessment of the specificity of radiopharmaceuticals containing short-lived isotopes.
- Lindmo T., Boven E., Cuttitta F., Fedorko J., Bunn P.A. Jr. Determination of the immunoreactive fraction of radiolabeled monoclonal antibodies by linear extrapolation to binding at infinite antigen excess. J Immunol Methods 1984; 72(1): 77–89, https://doi.org/10.1016/0022-1759(84)90435-6.
- Bian H.J., Chen Z.N., Deng J.L. Direct technetium-99m labeling of anti-hepatoma monoclonal antibody fragment: a radioimmunoconjugate for hepatocellular carcinoma imaging. World J Gastroenterol 2000; 6(3): 348–352, https://doi.org/10.3748/wjg.v6.i3.348.
- Burvenich I.J., Parakh S., Gan H.K., Lee F.T., Guo N., Rigopoulos A., Lee S.T., Gong S., O’Keefe G.J., Tochon-Danguy H., Kotsuma M., Hasegawa J., Senaldi G., Scott A.M. Molecular imaging and quantitation of EphA2 expression in xenograft models with 89Zr-DS-8895a. J Nucl Med 2016; 57(6): 974–980, https://doi.org/10.2967/jnumed.115.169839.
- Kristensen L.K., Fröhlich C., Christensen C., Melander M.C., Poulsen T.T., Galler G.R., Lantto J., Horak I.D., Kragh M., Nielsen C.H., Kjaer A. CD4+ and CD8a+ PET imaging predicts response to novel PD-1 checkpoint inhibitor: studies of Sym021 in syngeneic mouse cancer models. Theranostics 2019; 9(26): 8221–8238, https://doi.org/10.7150/thno.37513.
- Pruszynski M., D’Huyvetter M., Bruchertseifer F., Morgenstern A., Lahoutte T. Evaluation of an anti-HER2 nanobody labeled with 225Ac for targeted α-particle therapy of cancer. Mol Pharm 2018; 15(4): 1457–1466, https://doi.org/10.1021/acs.molpharmaceut.7b00985.
- Bellaye P.S., Moreau M., Raguin O., Oudot A., Bernhard C., Vrigneaud J.M., Dumont L., Vandroux D., Denat F., Cochet A., Brunotte F., Collin B. Radiolabeled F(ab’)2-cetuximab for theranostic purposes in colorectal and skin tumor-bearing mice models. Clin Transl Oncol 2018; 20(12): 1557–1570, https://doi.org/10.1007/s12094-018-1886-4.
- Mattes M.J. Limitations of the Lindmo method in determining antibody immunoreactivity. Int J Cancer 1995; 61(2): 286–288, https://doi.org/10.1002/ijc.2910610224.
- Dux R., Kindler-Röhrborn A., Lennartz K., Rajewsky M.F. Determination of immunoreactive fraction and kinetic parameters of a radiolabeled monoclonal antibody in the absence of antigen excess. J Immunol Methods 1991; 144(2): 175–183, https://doi.org/10.1016/0022-1759(91)90084-s.
- Konishi S., Hamacher K., Vallabhajosula S., Kothari P., Bastidas D., Bander N., Goldsmith S. Determination of immunoreactive fraction of radiolabeled monoclonal antibodies: what is an appropriate method? Cancer Biother Radiopharm 2004; 19(6): 706–715, https://doi.org/10.1089/cbr.2004.19.706.
- Sharma S.K., Lyashchenko S.K., Park H.A., Pillarsetty N., Roux Y., Wu J., Poty S., Tully K.M., Poirier J.T., Lewis J.S. A rapid bead-based radioligand binding assay for the determination of target-binding fraction and quality control of radiopharmaceuticals. Nucl Med Biol 2019; 71: 32–38, https://doi.org/10.1016/j.nucmedbio.2019.04.005.
- Vu T.N., Wills Q.F., Kalari K.R., Niu N., Wang L., Pawitan Y., Rantalainen M. Isoform-level gene expression patterns in single-cell RNA-sequencing data. Bioinformatics 2018; 34(14): 2392–2400, https://doi.org/10.1093/bioinformatics/bty100.
- Mouriaux F., Zaniolo K., Bergeron M.A., Weidmann C., De La Fouchardière A., Fournier F., Droit A., Morcos M.W., Landreville S., Guérin S.L. Effects of long-term serial passaging on the characteristics and properties of cell lines derived from uveal melanoma primary tumors. Invest Ophthalmol Vis Sci 2016; 57(13): 5288–5301, https://doi.org/10.1167/iovs.16-19317.
- Zalutsky M.R., Zhao X.G., Alston K.L., Bigner D. High-level production of alpha-particle-emitting (211)At and preparation of (211)At-labeled antibodies for clinical use. J Nucl Med 2001; 42(10): 1508–1515.
- Andersen L. Development of an analytical method for the determination of the antigen binding capacity of radiolabeled antibodies. MSc Thesis. Trondheim: Norwegian University of Science and Technology; 2012.
- Pirovano G., Ordonez A.A., Jain S.K., Reiner T., Carroll L.S., Pillarsetty N.V.K. Rapid detection of SARS-CoV-2 using a radiolabeled antibody. Nucl Med Biol 2021; 98–99: 69–75, https://doi.org/10.1016/j.nucmedbio.2021.05.002.
- Wang X., Teng F., Kong L., Yu J. PD-L1 expression in human cancers and its association with clinical outcomes. Onco Targets Ther 2016; 9: 5023–5039, https://doi.org/10.2147/ott.s105862.
- Sun C., Mezzadra R., Schumacher T.N. Regulation and function of the PD-L1 checkpoint. Immunity 2018; 48(3): 434–452, https://doi.org/10.1016/j.immuni.2018.03.014.
- Doroshow D.B., Bhalla S., Beasley M.B., Sholl L.M., Kerr K.M., Gnjatic S., Wistuba I.I., Rimm D.L., Tsao M.S., Hirsch F.R. PD-L1 as a biomarker of response to immune-checkpoint inhibitors. Nat Rev Clin Oncol 2021; 18(6): 345–362, https://doi.org/10.1038/s41571-021-00473-5.
- Jung H.I., Jeong D., Ji S., Ahn T.S., Bae S.H., Chin S., Chung J.C., Kim H.C., Lee M.S., Baek M.J. Overexpression of PD-L1 and PD-L2 is associated with poor prognosis in patients with hepatocellular carcinoma. Cancer Res Treat 2017; 49(1): 246–254, https://doi.org/10.4143/crt.2016.066.
- Rezaeeyan H., Hassani S.N., Barati M., Shahjahani M., Saki N. PD-1/PD-L1 as a prognostic factor in leukemia. J Hematopathol 2017; 10(1): 17–24, https://doi.org/10.1007/s12308-017-0293-z.
- Núñez Abad M., Calabuig-Fariñas S., Lobo de Mena M., Torres-Martínez S., García González C., García García J.Á., Iranzo González-Cruz V., Camps Herrero C. Programmed death-ligand 1 (PD-L1) as immunotherapy biomarker in breast cancer. Cancers (Basel) 2022; 14(2): 307, https://doi.org/10.3390/cancers14020307.
- Lv G., Sun X., Qiu L., Sun Y., Li K., Liu Q., Zhao Q., Qin S., Lin J. PET imaging of tumor PD-L1 expression with a highly specific nonblocking single-domain antibody. J Nucl Med 2020; 61(1): 117–122, https://doi.org/10.2967/jnumed.119.226712.
- Liu Q., Jiang L., Li K., Li H., Lv G., Lin J., Qiu L. Immuno-PET imaging of 68Ga-labeled nanobody Nb109 for dynamic monitoring the PD-L1 expression in cancers. Cancer Immunol Immunother 2021; 70(6): 1721–1733, https://doi.org/10.1007/s00262-020-02818-y.
- Qin S., Yu Y., Guan H., Yang Y., Sun F., Sun Y., Zhu J., Xing L., Yu J., Sun X. A preclinical study: correlation between PD-L1 PET imaging and the prediction of therapy efficacy of MC38 tumor with 68Ga-labeled PD-L1 targeted nanobody. Aging (Albany NY) 2021; 13(9): 13006–13022, https://doi.org/10.18632/aging.202981.
- Yu S., Xiong G., Zhao S., Tang Y., Tang H., Wang K., Liu H., Lan K., Bi X., Duan S. Nanobodies targeting immune checkpoint molecules for tumor immunotherapy and immunoimaging (review). Int J Mol Med 2021; 47(2): 444–454, https://doi.org/10.3892/ijmm.2020.4817.
- Kumar M., Khan I., Sinha S. Nature of immobilization surface affects antibody specificity to placental alkaline phosphatase. J Immunoassay Immunochem 2015; 36(4): 405–413, https://doi.org/10.1080/15321819.2014.973117.