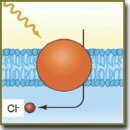
Оптогенетика: перспективы в области биомедицинских исследований (обзор)
Оптогенетические молекулы, фотохромные переключатели и генетически кодируемые биосенсоры произвели революцию в современных исследованиях функций нервной системы и других клеток биологических организмов. Эти подходы обеспечили беспрецедентные возможности для регистрации активности и дистанционной модуляции функций специфических нейронов и буквально пролили свет на механизмы функционирования нейронных сетей мозга.
В настоящее время используются разные классы светочувствительных биосенсоров для неинвазивного анализа концентраций внутриклеточных ионов и активности ферментов. Разнообразие этих молекулярных конструкций быстро возрастает и расширяются сферы их использования для визуализации и анализа белков и функций клеток. В этом обзоре обсуждаются инструментальные подходы, обеспечивающие дистанционный анализ активности рецепторов, ионных каналов и синаптических сетей, а также перспективы использования оптогенетических методов для биомедицинских исследований.
- Deisseroth K., Feng G., Majewska A.K., Miesenböck G., Ting A., Schnitzer M.J. Next-generation optical technologies for illuminating genetically targeted brain circuits. J Neurosci 2006; 26(41): 10380–10386, https://doi.org/10.1523/JNEUROSCI.3863-06.2006.
- Nagel G., Szellas T., Huhn W., Kateriya S., Adeishvili N., Berthold P., Ollig D., Hegemann P., Bamberg E. Channelrhodopsin-2, a directly light-gated cation-selective membrane channel. Proc Natl Acad Sci USA 2003; 100(24): 13940–13945, https://doi.org/10.1073/pnas.1936192100.
- Boyden E.S., Zhang F., Bamberg E., Nagel G., Deisseroth K. Millisecond-timescale, genetically targeted optical control of neural activity. Nat Neurosci 2005; 8(9): 1263–1268, https://doi.org/10.1038/nn1525.
- Li X., Gutierrez D.V., Hanson M.G., Han J., Mark M.D., Chiel H., Hegemann P., Landmesser L.T., Herlitze S. Fast noninvasive activation and inhibition of neural and network activity by vertebrate rhodopsin and green algae channelrhodopsin. Proc Natl Acad Sci USA 2005; 102(49): 17816–17821, https://doi.org/10.1073/pnas.0509030102.
- Lima S.Q., Miesenböck G. Remote control of behavior through genetically targeted photostimulation of neurons. Cell 2005; 121(1): 141–152, https://doi.org/10.1016/j.cell.2005.02.004.
- Jin L., Han Z., Platisa J., Wooltorton J.R.A., Cohen L.B., Pieribone V.A. Single action potentials and subthreshold electrical events imaged in neurons with a fluorescent protein voltage probe. Neuron 2012; 75(5): 779–785, https://doi.org/10.1016/j.neuron.2012.06.040.
- Cao G., Platisa J., Pieribone V.A., Raccuglia D., Kunst M., Nitabach M.N. Genetically targeted optical electrophysiology in intact neural circuits. Cell 2013; 154(4): 904–913, https://doi.org/10.1016/j.cell.2013.07.027.
- Marvin J.S., Borghuis B.G., Tian L., Cichon J., Harnett M.T., Akerboom J., Gordus A., Renninger S.L., Chen T.-W., Bargmann C.I., Orger M.B., Schreiter E.R., Demb J.B., Gan W.-B., Hires S.A., Looger L.L. An optimized fluorescent probe for visualizing glutamate neurotransmission. Nat Methods 2013; 10(2): 162–170, https://doi.org/10.1038/nmeth.2333.
- Bilan D.S., Pase L., Joosen L., Gorokhovatsky A.Y., Ermakova Y.G., Gadella T.W.J., Grabher C., Schultz C., Lukyanov S., Belousov V.V. HyPer-3: a genetically encoded H2O2 probe with improved performance for ratiometric and fluorescence lifetime imaging. ACS Chem Biol 2013; 8(3): 535–542, https://doi.org/10.1021/cb300625g.
- Berg J., Hung Y.P., Yellen G. A genetically encoded fluorescent reporter of ATP: ADP ratio. Nat Methods 2009; 6(2): 161–166, https://doi.org/10.1038/NMETH.1288.
- Tantama M., Martínez-François J.R., Mongeon R., Yellen G. Imaging energy status in live cells with a fluorescent biosensor of the intracellular ATP-to-ADP ratio. Nat Commun 2013; 4: 2550, https://doi.org/10.1038/ncomms3550.
- Chen T.-W., Wardill T.J., Sun Y., Pulver S.R., Renninger S.L., Baohan A., Schreiter E.R., Kerr R.A., Orger M.B., Jayaraman V., Looger L.L., Svoboda K., Kim D.S. Ultrasensitive fluorescent proteins for imaging neuronal activity. Nature 2013; 499(7458): 295–300, https://doi.org/ 10.1038/nature12354.
- Miyawaki A., Griesbeck O., Heim R., Tsien R.Y. Dynamic and quantitative Ca2+ measurements using improved cameleons. Proc Natl Acad Sci USA 1999; 96(5): 2135–2140, https://doi.org/10.1073/pnas.96.5.2135.
- Miyawaki A., Llopis J., Heim R., McCaffery J.M., Adams J.A., Ikura M., Tsien R.Y. Fluorescent indicators for Ca2+ based on green fluorescent proteins and calmodulin. Nature 1997; 388(6645): 882–887, https://doi.org/10.1038/42264.
- Kuner T., Augustine G.J. A genetically encoded ratiometric indicator for chloride: capturing chloride transients in cultured hippocampal neurons. Neuron 2000; 27(3): 447–459, https://doi.org/10.1016/S0896-6273(00)00056-8.
- Markova O., Mukhtarov M., Real E., Jacob Y., Bregestovski P. Genetically encoded chloride indicator with improved sensitivity. J Neurosci Methods 2008; 170(1): 67–76, https://doi.org/10.1016/j.jneumeth.2007.12.016.
- Mukhtarov M., Markova O., Real E., Jacob Y., Buldakova S., Bregestovski P. Monitoring of chloride and activity of glycine receptor channels using genetically encoded fluorescent sensors. Philos Trans A Math Phys Eng Sci 2008; 366(1880): 3445–3462, https://doi.org/10.1098/rsta.2008.0133.
- Arosio D., Ricci F., Marchetti L., Gualdani R., Albertazzi L., Beltram F. Simultaneous intracellular chloride and pH measurements using a GFP-based sensor. Nat Methods 2010; 7(7): 516–518, https://doi.org/10.1038/nmeth.1471.
- Mukhtarov M., Liguori L., Waseem T., Rocca F., Buldakova S., Arosio D., Bregestovski P. Calibration and functional analysis of three genetically encoded Cl–/pH sensors. Front Mol Neurosci 2013; 6: 9, https://doi.org/10.3389/fnmol.2013.00009.
- Sankaranarayanan S., De Angelis D., Rothman J.E., Ryan T.A. The use of pHluorins for optical measurements of presynaptic activity. Biophys J 2000; 79(4): 2199–2208, https://doi.org/10.1016/S0006-3495(00)76468-X.
- Imamura H., Nhat K.P.H., Togawa H., Saito K., Iino R., Kato-Yamada Y., Nagai T., Noji H. Visualization of ATP levels inside single living cells with fluorescence resonance energy transfer-based genetically encoded indicators. Proc Natl Acad Sci USA 2009; 106(37): 15651–15656, https://doi.org/10.1073/pnas.0904764106.
- Kumar N., Bhalla V., Kumar M. Resonance energy transfer-based fluorescent probes for Hg2+, Cu2+ and Fe2+/Fe3+ ions. Analyst 2014; 139(3): 543–558, https://doi.org/10.1039/c3an01896b.
- Hess G.P., Grewer C. Development and application of caged ligands for neurotransmitter receptors in transient kinetic and neuronal circuit mapping studies. Methods Enzymol 1998; 291: 443–473, https://doi.org/10.1016/S0076-6879(98)91028-X.
- Lester H.A., Krouse M.E., Nass M.M., Wassermann N.H., Erlanger B.F. Light-activated drug confirms a mechanism of ion channel blockade. Nature 1979; 280(5722): 509–510, https://doi.org/10.1038/280509a0.
- Volgraf M., Gorostiza P., Szobota S., Helix M.R., Isacoff E.Y., Trauner D. Reversibly caged glutamate: a photochromic agonist of ionotropic glutamate receptors. J Am Chem Soc 2007; 129(2): 260–261, https://doi.org/10.1021/ja067269o.
- Beharry A.A., Woolley G.A. Azobenzene photoswitches for biomolecules. Chem Soc Rev 2011; 40(8): 4422–4437, https://doi.org/10.1039/c1cs15023e.
- Volgraf M., Gorostiza P., Numano R., Kramer R.H., Isacoff E.Y., Trauner D. Allosteric control of an ionotropic glutamate receptor with an optical switch. Nat Chem Biol 2006; 2(1): 47–52, https://doi.org/10.1038/nchembio756.
- Numano R., Szobota S., Lau A.Y., Gorostiza P., Volgraf M., Roux B., Trauner D., Isacoff E.Y. Nanosculpting reversed wavelength sensitivity into a photoswitchable iGluR. Proc Natl Acad Sci USA 2009; 106(16): 6814–6819, https://doi.org/10.1073/pnas.0811899106.
- Sandoz G., Levitz J., Kramer R.H., Isacoff E.Y. Optical control of endogenous proteins with a photoswitchable conditional subunit reveals a role for TREK1 in GABAB signaling. Neuron 2012; 74(6): 1005–1014, https://doi.org/10.1016/j.neuron.2012.04.026.
- Tochitsky I., Banghart M.R., Mourot A., Yao J.Z., Gaub B., Kramer R.H., Trauner D. Optochemical control of genetically engineered neuronal nicotinic acetylcholine receptors. Nat Chem 2012; 4(2): 105–111, https://doi.org/10.1038/nchem.1234.
- Li H., Avery L., Denk W., Hess G.P. Identification of chemical synapses in the pharynx of Caenorhabditis elegans. Proc Natl Acad Sci USA 1997; 94(11): 5912–5916, https://doi.org/10.1073/pnas.94.11.5912.
- Lancaster B., Hu H., Gibb B., Storm J.F. Kinetics of ion channel modulation by cAMP in rat hippocampal neurones. J Physiol 2006; 576(2): 403–417, https://doi.org/10.1113/jphysiol.2006.115295.
- Dibattista M., Amjad A., Maurya D.K., Sagheddu C., Montani G., Tirindelli R., Menini A. Calcium-activated chloride channels in the apical region of mouse vomeronasal sensory neurons. J Gen Physiol 2012; 140(1): 3–15, https://doi.org/10.1085/jgp.201210780.
- Matsuzaki M., Hayama T., Kasai H., Ellis-Davies G.C.R. Two-photon uncaging of gamma-aminobutyric acid in intact brain tissue. Nat Chem Biol 2010; 6(4): 255–257, https://doi.org/10.1038/nchembio.321.
- Bort G., Gallavardin T., Ogden D., Dalko P.I. From one-photon to two-photon probes: “caged” compounds, actuators, and photoswitches. Angew Chem Int Ed Engl 2013; 52(17): 4526–4537, https://doi.org/10.1002/anie.201204203.
- Bloodgood B.L., Sabatini B.L. Neuronal activity regulates diffusion across the neck of dendritic spines. Science 2005; 310(5749): 866–869, https://doi.org/10.1126/science.1114816.
- Noguchi J., Nagaoka A., Watanabe S., Ellis-Davies G.C.R., Kitamura K., Kano M., Matsuzaki M., Kasai H. In vivo two-photon uncaging of glutamate revealing the structure-function relationships of dendritic spines in the neocortex of adult mice. J Physiol 2011; 589(10): 2447–2457, https://doi.org/10.1016/j.neures.2011.07.891.
- Govindarajan A., Israely I., Huang S.Y., Tonegawa S. The dendritic branch is the preferred integrative unit for protein synthesis-dependent LTP. Neuron 2011; 69(1): 132–146, https://doi.org/10.1016/j.neuron.2010.12.008.
- Rau H. Spectroscopic properties of organic azo compounds. Angew Chem Int Ed Engl 1973; 12(3): 224–235, https://doi.org/10.1002/anie.197302241.
- Bartels E., Wassermann N.H., Erlanger B.F. Photochromic activators of the acetylcholine receptor. Proc Natl Acad Sci USA 1971; 68(8): 1820–1823, https://doi.org/10.1073/pnas.68.8.1820.
- Bartels-Bernal E., Rosenberry T.L., Chang H.W. A membrane activation cycle induced by sulfhydryl reagents after affinity labeling of the acetylcholine receptor of electroplax. Mol Pharmacol 1976; 12(5): 813–819.
- Lester H.A., Krouse M.E., Nass M.M., Wassermann N.H., Erlanger B.F. A covalently bound photoisomerizable agonist: comparison with reversibly bound agonists at Electrophorus electroplaques. J Gen Physiol 1980; 75(2): 207–232, https://doi.org/10.1085/jgp.75.2.207.
- Stein M., Middendorp S.J., Carta V., Pejo E., Raines D.E., Forman S.A., Sigel E., Trauner D. Azo-propofols: photochromic potentiators of GABAA receptors. Angew Chem Int Ed Engl 2012; 51(42): 10500–10504, https://doi.org/10.1002/anie.201205475.
- Yue L., Pawlowski M., Dellal S.S., Xie A., Feng F., Otis T.S., Bruzik K.S., Qian H., Pepperberg D.R. Robust photoregulation of GABAA receptors by allosteric modulation with a propofol analogue. Nat Commun 2012; 3: 1095, https://doi.org/10.1038/ncomms2094.
- Szobota S., Gorostiza P., Del Bene F., Wyart C., Fortin D.L., Kolstad K.D., Tulyathan O., Volgraf M., Numano R., Aaron H.L., Scott E.K., Kramer R.H., Flannery J., Baier H., Trauner D., Isacoff E.Y. Remote control of neuronal activity with a light-gated glutamate receptor. Neuron 2007; 54(4): 535–545, https://doi.org/10.1016/j.neuron.2007.05.010.
- Banghart M., Borges K., Isacoff E., Trauner D., Kramer R.H. Light-activated ion channels for remote control of neuronal firing. Nat Neurosci 2004; 7(12): 1381–1386, https://doi.org/10.1038/nn1356.
- Chambers J.J., Banghart M.R., Trauner D., Kramer R.H. Light-induced depolarization of neurons using a modified Shaker K+ channel and a molecular photoswitch. J Neurophysiol 2006; 96(5): 2792–2796, https://doi.org/10.1152/jn.00318.2006.
- Fortin D.L., Dunn T.W., Fedorchak A., Allen D., Montpetit R., Banghart M.R., Trauner D., Adelman J.P., Kramer R.H. Optogenetic photochemical control of designer K+ channels in mammalian neurons. J Neurophysiol 2011; 106(1): 488–496, https://doi.org/10.1152/jn.00251.2011.
- Janovjak H., Szobota S., Wyart C., Trauner D., Isacoff E.Y. A light-gated, potassium-selective glutamate receptor for the optical inhibition of neuronal firing. Nat Neurosci 2010; 13(8): 1027–1032, https://doi.org/10.1038/nn.2589.
- Gorostiza P., Isacoff E.Y. Optical switches for remote and noninvasive control of cell signaling. Science 2008; 322(5900): 395–399, https://doi.org/10.1126/science.1166022.
- Miesenböck G. Optogenetic control of cells and circuits. Annu Rev Cell Dev Biol 2011; 27: 731–758, https://doi.org/10.1146/annurev-cellbio-100109-104051.
- Nagel G., Ollig D., Fuhrmann M., Kateriya S., Musti A.M., Bamberg E., Hegemann P. Channelrhodopsin-1: a light-gated proton channel in green algae. Science 2002; 296(5577): 2395–2398, https://doi.org/10.1126/science.1072068.
- Herlitze S., Landmesser L.T. New optical tools for controlling neuronal activity. Curr Opin Neurobiol 2007; 17(1): 87–94, https://doi.org/10.1016/j.conb.2006.12.002.
- Deisseroth K. Optogenetics. Nat Methods 2011; 8(1): 26–29, https://doi.org/10.1038/nmeth.f.324.
- Airan R.D., Thompson K.R., Fenno L.E., Bernstein H., Deisseroth K. Temporally precise in vivo control of intracellular signalling. Nature 2009; 458(7241): 1025–1029, https://doi.org/10.1038/nature07926.
- Zhang F., Prigge M., Beyrière F., Tsunoda S.P., Mattis J., Yizhar O., Hegemann P., Deisseroth K. Red-shifted optogenetic excitation: a tool for fast neural control derived from Volvox carteri. Nat Neurosci 2008; 11(6): 631–633, https://doi.org/10.1038/nn.2120.
- Govorunova E.G., Spudich E.N., Lane C.E., Sineshchekov O.A., Spudich J.L. New channelrhodopsin with a red-shifted spectrum and rapid kinetics from Mesostigma viride. MBio 2011; 2(3): e00115–e00111, https://doi.org/10.1128/mBio.00115-11.
- Lin J.Y., Lin M.Z., Steinbach P., Tsien R.Y. Characterization of engineered channelrhodopsin variants with improved properties and kinetics. Biophys J 2009; 96(5): 1803–1814, https://doi.org/10.1016/j.bpj.2008.11.034.
- Lin J.Y. A user’s guide to channelrhodopsin variants: features, limitations and future developments. Exp Physiol 2011; 96(1): 19–25, https://doi.org/10.1113/expphysiol.2009.051961.
- Packer A.M., Peterka D.S., Hirtz J.J., Prakash R., Deisseroth K., Yuste R. Two-photon optogenetics of dendritic spines and neural circuits. Nat Methods 2012; 9(12): 1202–1205, https://doi.org/10.1038/nmeth.2249.
- Lin J.Y., Knutsen P.M., Muller A., Kleinfeld D., Tsien R.Y. ReaChR: a red-shifted variant of channelrhodopsin enables deep transcranial optogenetic excitation. Nat Neurosci 2013; 16(10): 1499–1508, https://doi.org/10.1038/nn.3502.
- Oesterhelt D., Stoeckenius W. Rhodopsin-like protein from the purple membrane of Halobacterium halobium. Nat New Biol 1971; 233(39): 149–152, https://doi.org/10.1038/newbio233149a0.
- Oesterhelt D., Stoeckenius W. Functions of a new photoreceptor membrane. Proc Natl Acad Sci USA 1973; 70(10): 2853–2857, https://doi.org/10.1073/pnas.70.10.2853.
- Lanyi J.K., Weber H.J. Spectrophotometric identification of the pigment associated with light-driven primary sodium translocation in Halobacterium halobium. J Biol Chem 1980; 255(1): 243–250.
- Schobert B., Lanyi J.K. Halorhodopsin is a light-driven chloride pump. J Biol Chem 1982; 257(17): 10306–10313.
- Zhang F., Wang L.-P., Brauner M., Liewald J.F., Kay K., Watzke N., Wood P.G., Bamberg E., Nagel G., Gottschalk A., Deisseroth K. Multimodal fast optical interrogation of neural circuitry. Nature 2007; 446(7136): 633–639, https://doi.org/10.1038/nature05744.
- Smedemark-Margulies N., Trapani J.G. Tools, methods, and applications for optophysiology in neuroscience. Front Mol Neurosci2013; 6: 18, https://doi.org/10.3389/fnmol.2013.00018.
- Diester I., Kaufman M.T., Mogri M., Pashaie R., Goo W., Yizhar O., Ramakrishnan C., Deisseroth K., Shenoy K.V. An optogenetic toolbox designed for primates. Nat Neurosci 2011; 14(3): 387–397, https://doi.org/10.1038/nn.2749.
- Fenno L., Yizhar O., Deisseroth K. The development and application of optogenetics. Annu Rev Neurosci 2011; 34: 389–412, https://doi.org/10.1146/annurev-neuro-061010-113817.
- Han X., Chow B.Y., Zhou H., Klapoetke N.C., Chuong A., Rajimehr R., Yang A., Baratta M.V., Winkle J., Desimone R., Boyden E.S. A high-light sensitivity optical neural silencer: development and application to optogenetic control of non-human primate cortex. Front Syst Neurosci 2011; 5: 18, https://doi.org/10.3389/fnsys.2011.00018.
- Gerits A., Farivar R., Rosen B.R., Wald L.L., Boyden E.S., Vanduffel W. Optogenetically induced behavioral and functional network changes in primates. Curr Biol 2012; 22(18): 1722–1726, https://doi.org/10.1016/j.cub.2012.07.023.
- Welberg L. Techniques: optogenetic control in monkey brains. Nat Rev Neurosci 2012; 13(9): 603, https://doi.org/10.1038/nrn3331.
- Busskamp V., Duebel J., Balya D., Fradot M., Viney T.J., Siegert S., Groner A.C., Cabuy E., Forster V., Seeliger M., Biel M., Humphries P., Paques M., Mohand-Said S., Trono D., Deisseroth K., Sahel J.A., Picaud S., Roska B. Genetic reactivation of cone photoreceptors restores visual responses in retinitis pigmentosa. Science 2010; 329(5990): 413–417, https://doi.org/10.1126/science.1190897.
- Steinbeck J.A., Choi S.J., Mrejeru A., Ganat Y., Deisseroth K., Sulzer D., Mosharov E.V., Studer L. Optogenetics enables functional analysis of human embryonic stem cell-derived grafts in a Parkinson’s disease model. Nat Biotechnol 2015; 33(2): 204–209, https://doi.org/10.1038/nbt.3124.
- Deer T.R., Krames E., Mekhail N., Pope J., Leong M., Stanton-Hicks M., Golovac S., Kapural L., Alo K., Anderson J., Foreman R.D., Caraway D., Narouze S., Linderoth B., Buvanendran A., Feler C., Poree L., Lynch P., McJunkin T., Swing T., Staats P., Liem L., Williams K. The appropriate use of neurostimulation: new and evolving neurostimulation therapies and applicable treatment for chronic pain and selected disease states. Neuromodulation 2014; 17(6): 599–615, https://doi.org/10.1111/ner.12204.
- Capelle H.H., Krauss J.K. Neuromodulation in dystonia: current aspects of deep brain stimulation. Neuromodulation 2009; 12(1): 8–21, https://doi.org/10.1111/j.1525-1403.2009.00183.x.
- Liu K.-D., Shan D.-E., Kuo T.B.J., Yang C.C.H. The effects of bilateral stimulation of the subthalamic nucleus on heart rate variability in patients with Parkinson’s disease. J Neurol 2013; 260(7): 1714–1723, https://doi.org/10.1007/s00415-013-6849-7.
- Witt K., Daniels C., Reiff J., Krack P., Volkmann J., Pinsker M.O., Krause M., Tronnier V., Kloss M., Schnitzler A., Wojtecki L., Bötzel K., Danek A., Hilker R., Sturm V., Kupsch A., Karner E., Deuschl G. Neuropsychological and psychiatric changes after deep brain stimulation for Parkinson’s disease: a randomised, multicentre study. Lancet Neurol 2008; 7(7): 605–614, https://doi.org/10.1016/S1474-4422(08)70114-5.
- Covington H.E. 3rd, Lobo M.K., Maze I., Vialou V., Hyman J.M., Zaman S., LaPlant Q., Mouzon E., Ghose S., Tamminga C.A., Neve R.L., Deisseroth K., Nestler E.J. Antidepressant effect of optogenetic stimulation of the medial prefrontal cortex. J Neurosci 2010; 30(48): 16082–16090, https://doi.org/10.1523/JNEUROSCI.1731-10.2010.
- Haubensak W., Kunwar P.S., Cai H., Ciocchi S., Wall N.R., Ponnusamy R., Biag J., Dong H.-W., Deisseroth K., Callaway E.M., Fanselow M.S., Lüthi A., Anderson D.J. Genetic dissection of an amygdala microcircuit that gates conditioned fear. Nature 2010; 468(7321): 270–276, https://doi.org/10.1038/nature09553.
- Williams J.C., Denison T. From optogenetic technologies to neuromodulation therapies. Sci Transl Med 2013; 5(177): 177ps6, https://doi.org/10.1126/scitranslmed.3003100.
- Wagner F.B., Truccolo W., Wang J., Nurmikko A.V. Spatiotemporal dynamics of optogenetically induced and spontaneous seizure transitions in primary generalized epilepsy. J Neurophysiol 2015; 113(7): 2321–2341, https://doi.org/10.1152/jn.01040.2014.
- Rossi M.A., Calakos N., Yin H.H. Spotlight on movement disorders: what optogenetics has to offer. Mov Disord 2015; 30(5): 624–631, https://doi.org/10.1002/mds.26184.
- Tønnesen J., Sørensen A.T., Deisseroth K., Lundberg C., Kokaia M. Optogenetic control of epileptiform activity. Proc Natl Acad Sci USA 2009; 106(29): 12162–12167, https://doi.org/10.1073/pnas.0901915106.
- Sukhotinsky I., Chan A.M., Ahmed O.J., Rao V.R., Gradinaru V., Ramakrishnan C., Deisseroth K., Majewska A.K., Cash S.S. Optogenetic delay of status epilepticus onset in an in vivo rodent epilepsy model. PLoS One 2013; 8(4): e62013, https://doi.org/10.1371/journal.pone.0062013.
- Krook-Magnuson E., Armstrong C., Oijala M., Soltesz I. On-demand optogenetic control of spontaneous seizures in temporal lobe epilepsy. Nat Commun 2013; 4: 1376, https://doi.org/10.1038/ncomms2376.
- Ledri M., Madsen M.G., Nikitidou L., Kirik D., Kokaia M. Global optogenetic activation of inhibitory interneurons during epileptiform activity. J Neurosci 2014; 34(9): 3364–3377, https://doi.org/10.1523/JNEUROSCI.2734-13.2014.
- Sahel J.-A., Roska B. Gene therapy for blindness. Annu Rev Neurosci 2013; 36: 467–488, https://doi.org/10.1146/annurev-neuro-062012-170304.
- Bi A., Cui J., Ma Y.P., Olshevskaya E., Pu M., Dizhoor A.M., Pan Z.H. Ectopic expression of a microbial-type rhodopsin restores visual responses in mice with photoreceptor degeneration. Neuron 2006; 50(1): 23–33, https://doi.org/10.1016/j.neuron.2006.02.026.
- Tomita H., Sugano E., Isago H., Hiroi T., Wang Z., Ohta E., Tamai M. Channelrhodopsin-2 gene transduced into retinal ganglion cells restores functional vision in genetically blind rats. Exp Eye Res 2010; 90(3): 429–436, https://doi.org/10.1016/j.exer.2009.12.006.
- Macé E., Caplette R., Marre O., Sengupta A., Chaffiol A., Barbe P., Desrosiers M., Bamberg E., Sahel J.-A., Picaud S., Duebel J., Dalkara D. Targeting channelrhodopsin-2 to ON-bipolar cells with vitreally administered AAV restores ON and OFF visual responses in blind mice. Mol Ther 2015; 23(1): 7–16, https://doi.org/10.1038/mt.2014.154.
- Gaub B.M., Berry M.H., Holt A.E., Isacoff E.Y., Flannery J.G. Optogenetic vision restoration using rhodopsin for enhanced sensitivity. Mol Ther 2015; 23(10): 1562–1571, https://doi.org/10.1038/mt.2015.121.
- Bennett J., Ashtari M., Wellman J., Marshall K.A., Cyckowski L.L., Chung D.C., McCague S., Pierce E.A., Chen Y., Bennicelli J.L., Zhu X., Ying G.S., Sun J., Wright J.F., Auricchio A., Simonelli F., Shindler K.S., Mingozzi F., High K.A., Maguire A.M. AAV2 gene therapy readministration in three adults with congenital blindness. Sci Transl Med 2012; 4(120): 120ra15, https://doi.org/10.1126/scitranslmed.3002865.
- Polosukhina A., Litt J., Tochitsky I., Nemargut J., Sychev Y., De Kouchkovsky I., Huang T., Borges K., Trauner D., Van Gelder R.N., Kramer R.H. Photochemical restoration of visual responses in blind mice. Neuron 2012; 75(2): 271–282, https://doi.org/10.1016/j.neuron.2012.05.022.