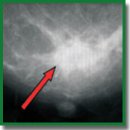
Современные методы оценки кислородного статуса и состояния микроциркуляции биотканей: оптическая диффузионная спектроскопия (обзор)
Проблема изучения кислородного статуса и микроциркуляции биотканей представляет особый интерес для многих направлений медицинской науки, поскольку одной из причин развития гипоксии как типового патофизиологического процесса является микроциркуляторный «сбой», связанный с нарушением нормальной анатомии капиллярной стенки, изменением реологических свойств крови, ускорением или замедлением микрокровотока. Современные методы медицинской визуализации позволяют изучить процессы жизнедеятельности биосистем на самых разных уровнях организации: от органов и тканей до молекулярного состава веществ. Благодаря внедрению в клиническую практику методов функционального биоимиджинга появилась возможность наблюдать в режиме реального времени за процессами перемещения веществ в организме, мониторить параметры кровотока, оценивать уровень гипоксии, более детально характеризовать метаболизм и при этом вовремя проводить корректировку патологических состояний.
В обзоре представлены основные достоинства и недостатки таких методов имиджинговых исследований, как функциональная магнитно-резонансная томография в режиме BOLD, позитронно-эмиссионная томография, оптический имиджинг, лазерная доплеровская флоуметрия, транскутанная оксиметрия. Особое внимание уделено перспективной неинвазивной методике прижизненного изучения содержания веществ в биотканях — оптической диффузионной спектроскопии.
Принцип оптической диффузионной спектроскопии основан на способности тканевых хромофоров (оксигемоглобин, дезоксигемоглобин, жирные кислоты, коллаген) поглощать диффузно-рассеянный свет определенной длины волны. С учетом коэффициентов поглощения хромофоров рассчитывают их концентрации. Оптическая диффузионная спектроскопия внедряется в клиническую практику для диагностики степени малигнизации опухолей, оценки васкуляризации при реконструктивных операциях, диагностики гипоксических состояний тканей, интраоперационного мониторинга параметров кровотока, определения уровня гипоксии при сахарном диабете. С ее помощью представляется возможным определение и уточнение показаний к кожно-пластическим операциям и, возможно, к разработке новых способов кожной пластики.
- Копицын Д.С., Бескоровайный А.В., Котелев М.С., Новиков А.А., Иванов Е.В., Винокуров Е.В. Методы оптического биоимиджинга в исследованиях онкологических заболеваний. Башкирский химический журнал 2013; 4: 64–71.
- Meleshina А.V., Cherkasova Е.I., Sergeeva Е.А., Kleshnin М.S., Turchin I.V., Kiseleva Е.V., Dashinimaev E.V., Shirmanova М.V., Lukyanov S.А., Zagaynova Е.V. The study of the interaction of mesenchymal stem cells and the tumor using the methods of fluorescent bioimaging. Sovremennye tehnologii v medicine 2012; 4: 7–16.
- Meleshina A.V., Cherkasova E.I., Shirmanova M.V., Khrapichev A.A., Dudenkova V.V., Zagaynova E.V. Modern techniques for stem cells in vivo imaging (review). Sovremennye tehnologii v medicine 2015; 7(4): 174–188, https://doi.org/10.17691/stm2015.7.4.24.
- Kozubek M. Challenges and benchmarks in bioimage analysis. Adv Anat Embryol Cell Biol 2016; 219: 231–262, https://doi.org/10.1007/978-3-319-28549-8_9.
- Karsy M., Gillespie D.L., Horn K.P., Burrell L.D., Yap J.T., Jensen R.L. Correlation of glioma proliferation and hypoxia by luciferase, magnetic resonance, and positron emission tomography imaging. Methods Mol Biol 2018; 1742: 301–320, https://doi.org/10.1007/978-1-4939-7665-2_26.
- Wicks E.C., Menezes L.J., Barnes A., Mohiddin S.A., Sekhri N., Porter J.C., Booth H.L., Garrett E., Patel R.S., Pavlou M., Groves A.M., Elliott P.M. Diagnostic accuracy and prognostic value of simultaneous hybrid 18F-fluorodeoxyglucose positron emission tomography/magnetic resonance imaging in cardiac sarcoidosis. Eur Heart J Cardiovasc Imaging 2018; 19(7): 757–767, https://doi.org/10.1093/ehjci/jex340.
- Кремнева Е.И., Коновалов Р.Н., Кротенкова М.В. Функциональная магнитно-резонансная томография. Анналы клинической и экспериментальной неврологии 2011; 5(1): 30–34.
- Селиверстова Е.В., Селиверстов Ю.А., Коновалов Р.Н., Иллариошкин С.Н. Функциональная магнитно-резонансная томография покоя: новые возможности изучения физиологии и патологии мозга. Анналы клинической и экспериментальной неврологии 2013; 7(4): 39–44.
- Шахова Н.М., Балалаева И.В., Геликонов В.М., Геликонов Г.В., Загайнова Е.В., Каменский В.А., Орлова А.Г., Сергеева Е.А., Турчин И.В. Разномасштабный оптический биоимиджинг: использование в биомедицине и перспективы развития. Альманах клинической медицины 2008; 17–1: 121–124.
- Дуванский В.А., Князев М.В., Осин В.Л., Краев Г.П. Технологии оптического биоимиджинга в визуализации гастроинтестинальных неоплазий. Лазерная медицина 2016; 20(3): 103–104.
- Marques D., Miranda A., Silva A.G., Munro P.R.T., De Beule P.A.A. On the influence of lipid-induced optical anisotropy for the bioimaging of exo- or endocytosis with interference microscopic imaging. J Microsc 2018; 270(2): 150–155, https://doi.org/10.1111/jmi.12668.
- Турчин И.В. Методы оптической биомедицинской визуализации: от субклеточных структур до тканей и органов. Успехи физических наук 2016; 186(5): 550–567, https://doi.org/10.3367/ufnr.2015.12.037734.
- Малышев И.Ю., Монастырская Е.А., Смирин Б.В., Манухина Е.Б. Гипоксия и оксид азота. Вестник Российской академии медицинских наук 2000; 9: 44–48.
- Reginelli A., Iacobellis F., Berritto D., Gagliardi G., Di Grezia G., Rossi M., Fonio P., Grassi R. Mesenteric ischemia: the importance of differential diagnosis for the surgeon. BMC Surg 2013; 13(Suppl 2): S51, https://doi.org/10.1186/1471-2482-13-s2-s51.
- Баширов Р.А., Малов А.А., Зиганшина Л.Ф., Халиуллина К.К. Радионуклидная оценка скелетных мышц у пациентов с хронической артериальной недостаточностью нижних конечностей. Практическая медицина 2016; 4–1(96): 44–47.
- Jia Y., Wang R.K. Optical micro-angiography images structural and functional cerebral blood perfusion in mice with cranium left intact. J Biophotonics 2011; 4(1–2): 57–63, https://doi.org/10.1002/jbio.201000001.
- Asai K., Nakamura H., Watabe T., Nishida T., Sakaguchi M., Hatazawa J., Yoshimine T., Kishima H. X-ray angiography perfusion imaging with an intra-arterial injection: comparative study with 15O-gas/water positron emission tomography. J Neurointerv Surg 2017; 10(8): 780–783, http://dx.doi.org/10.1136/neurintsurg-2017-013487.
- Johns C.S., Swift A.J., Hughes P.J.C., Ohno Y., Schiebler M., Wild J.M. Pulmonary MR angiography and perfusion imaging — a review of methods and applications. Eur J Radiol 2017; 86: 361–370, https://doi.org/10.1016/j.ejrad.2016.10.003.
- Gallis K., Kasprzak P.M., Cucuruz B., Kopp R. Evaluation of visible spinal arteries on computed tomography angiography before and after branched stent graft repair for thoracoabdominal aortic aneurysm. J Vasc Surg 2017; 65(6): 1577–1583, https://doi.org/10.1016/j.jvs.2016.10.118.
- de Bruin A.F., Kornmann V.N., van der Sloot K., van Vugt J.L., Gosselink M.P., Smits A., Van Ramshorst B., Boerma E.C., Noordzij P.G., Boerma D., van Iterson M. Sidestream dark field imaging of the serosal microcirculation during gastrointestinal surgery. Colorectal Dis 2016; 18(3): 103–110, https://doi.org/10.1111/codi.13250.
- van Elteren H.A., Ince C., Tibboel D., Reiss I.K., de Jonge R.C. Cutaneous microcirculation in preterm neonates: comparison between sidestream dark field (SDF) and incident dark field (IDF) imaging. J Clin Monit Comput 2015; 29(5): 543–548, https://doi.org/10.1007/s10877-015-9708-5.
- Bajory Z., Szabó A., Deák G., Varga R., Pajor L. Orthogonal polarization spectral imaging: a novel tool for examination of microcirculatory changes in the testis. J Androl 2012; 33(3): 499–504, https://doi.org/10.2164/jandrol.111.013599.
- van Zijderveld R., Ince C., Schlingemann R.O. Orthogonal polarization spectral imaging of conjunctival microcirculation. Graefes Arch Clin Exp Ophthalmol 2014; 252(5): 773–779, https://doi.org/10.1007/s00417-014-2603-9.
- Kawada K., Hasegawa S., Wada T., Takahashi R., Hisamori S., Hida K., Sakai Y. Evaluation of intestinal perfusion by ICG fluorescence imaging in laparoscopic colorectal surgery with DST anastomosis. Surg Endosc 2017; 31(3): 1061–1069, https://doi.org/10.1007/s00464-016-5064-x.
- Lim C., Malek A., Martins R., Petillon S., Boulate G., Hentati H., De’Angelis N., Brunetti F., Salloum C., Laurent A., Compagnon P., Azoulay D. Real-time assessment of intestinal viability using indocyanine green fluorescent imaging (with video). J Visc Surg 2015; 152(1): 71–72, https://doi.org/10.1016/j.jviscsurg.2014.09.008.
- Raabe A., Beck J., Seifert V. Technique and image quality of intraoperative indocyanine green angiography during aneurysm surgery using surgical microscope integrated near-infrared video technology. Zentralbl Neurochir 2005; 66(1): 1–8, https://doi.org/10.1055/s-2004-836223.
- Moon H.S., Joo S.P., Seo B.R., Jang J.W., Kim J.H., Kim T.S. Value of indocyanine green videoangiography in deciding the completeness of cerebrovascular surgery. J Korean Neurosurg Soc 2013; 53(6): 349–355, https://doi.org/10.3340/jkns.2013.53.6.349.
- Dashti R., Laakso A., Niemelä M., Porras M., Hernesniemi J. Microscope-integrated near-infrared indocyanine green videoangiography during surgery of intracranial aneurysms: the Helsinki experience. Surg Neurol 2009; 71(5): 543–550, https://doi.org/10.1016/j.surneu.2009.01.027.
- Мавликеев М.О., Титова А.А., Гудз Д.О., Деев Р.В. Современные методы исследования ангиогенеза в клинической практике. Наука молодых — Eruditio Juvenium 2017; 5(1): 110–123.
- Wang Z., Cai Y., Liang Y., Zhou X., Yan S., Dan D., Piero R. Bianco, Lei M., Yao B. Single shot, three-dimensional fluorescence microscopy with a spatially rotating point spread function. Biomed Opt Express 2017; 8(12): 5493–5506, https://doi.org/10.1364/boe.8.005493.
- Washington C.W., Zipfel G.J., Chicoine M.R., Derdeyn C.P., Rich K.M., Moran C.J., Cross D.T., Dacey R.G. Jr. Comparing indocyanine green videoangiography to the gold standard of intraoperative digital subtraction angiography used in aneurysm surgery. J Neurosurg 2013; 118(2): 420–427, https://doi.org/10.3171/2012.10.jns11818.
- Alander J.T., Kaartinen I., Laakso A., Pätilä T., Spillmann T., Tuchin V.V., Venermo M., Välisuo P. A review of indocyanine green fluorescent imaging in surgery. Int J Biomed Imaging 2012; 2012: 940585, https://doi.org/10.1155/2012/940585.
- Dong L., Zhang X., Liang F., Yu X., Yang T., Li L. Prognostic value of oxygen challenge test for patients with cardiogenic shock receiving extracorporeal membrane oxygenation. Zhonghua Wei Zhong Bing Ji Jiu Yi Xue 2017; 29(12): 1102–1106.
- Raposio E., Bertozzi N., Moretti R., Grignaffini E., Grieco M.P. Laser Doppler flowmetry and transcutaneous oximetry in chronic skin ulcers: a comparative evaluation. Wounds 2017; 29(7): 190–195.
- Trinks T.P., Blake D.F., Young D.A., Thistlethwaite K., Vangaveti V.N. Transcutaneous oximetry measurements of the leg: comparing different measuring equipment and establishing values in healthy young adults. Diving Hyperb Med 2017; 47(2): 82–87.
- Khripun A.I., Shurygin S.N., Priamikov A.D., Mironkov A.B., Abashin M.V. Intestinal microcirculation in health and in acute impairment of mesenteric blood flow. Angiol Sosud Khir 2010; 16(3): 34–38.
- Kouadio A.A., Jordana F., Koffi N.J., Le Bars P., Soueidan A. The use of laser Doppler flowmetry to evaluate oral soft tissue blood flow in humans: a review. Arch Oral Biol 2018; 86: 58–71, https://doi.org/10.1016/j.archoralbio.2017.11.009.
- Binzoni T., Martelli F. Study on the mathematical relationship existing between single-photon laser-Doppler flowmetry and diffuse correlation spectroscopy with static background. J Opt Soc Am A Opt Image Sci Vis 2017; 34(12): 2096–2101, https://doi.org/10.1364/josaa.34.002096.
- Hsiu H., Hu H.F., Tsai H.C. Differences in laser-Doppler indices between skin-surface measurement sites in subjects with diabetes. Microvasc Res 2018; 115: 1–7, https://doi.org/10.1016/j.mvr.2017.07.004.
- Ehling J., Lammers T., Kiessling F. Non-invasive imaging for studying anti-angiogenic therapy effects. Thromb Haemost 2013; 109(3): 375–390, https://doi.org/10.1160/th12-10-0721.
- Quaranta M., Borisov S.M., Klimant I. Indicators for optical oxygen sensors. Bioanal Rev 2012; 4(2–4): 115–157, https://doi.org/10.1007/s12566-012-0032-y.
- Keller A. Noninvasive tissue oximetry for flap monitoring: an initial study. J Reconstr Microsurg 2007; 23(212): 189–197, https://doi.org/10.1055/s-2007-974655.
- Rao R., Saint-Cyr M., Ma A.M., Bowling M., Hatef D.A., Andrews V., Xie X.J., Zogakis T., Rohrich R. Prediction of post-operative necrosis after mastectomy: a pilot study utilizing optical diffusion imaging spectroscopy. World J Surg Oncol 2009; 7(1): 91, https://doi.org/10.1186/1477-7819-7-91.
- Zimnyakov D.A., Tuchin V.V. Optical tomography of tissues. Quantum Electronics 2002; 32(10): 849–867, https://doi.org/10.1070/qe2002v032n10abeh002307.
- Durduran T., Choe R., Baker W., Yodh A. Diffuse optics for tissue monitoring and tomography. Rep Prog Phys 2010; 73(7): 076701, https://doi.org/10.1088/0034-4885/73/7/076701.
- Konovalov A., Genina E., Bashkatov A. Diffuse optical mammotomography: state-of-the-art and prospects. Journal of Biomedical Photonics & Engineering 2016; 2(2): 020202-1, https://doi.org/10.18287/jbpe16.02.020202.
- Chen W., Wang X., Wang B., Wang Y., Zhang Y., Zhao H., Gao F. Lock-in-photon-counting-based highly-sensitive and large-dynamic 164 imaging system for continuous-wave diffuse optical tomography. Biomed Opt Express 2016; 7(2): 499–511, https://doi.org/10.1364/boe.7.000499.
- Pham T., Hornung R., Ha H., Burney T., Serna D., Powell L., Brenner M., Tromberg B. Noninvasive monitoring of hemodynamic stress using quantitative near-infrared frequency-domain photon migration spectroscopy. J Biomed Opt 2002; 7(1): 34–44, https://doi.org/10.1117/1.1427046.
- Yamada Y., Okawa S. Diffuse optical tomography: present status and its future. Optical Review 2014; 21(3): 185–205, https://doi.org/10.1007/s10043-014-0028-7.
- Ban H.Y., Schweiger M., Kavuri V.C., Cochran J.M., Xie L., Busch D.R., Katrašnik J., Pathak S., Chung S.H., Lee K., Choe R., Czerniecki B.J., Arridge S.R., Yodh A.G. Heterodyne frequency-domain multispectral diffuse optical tomography of breast cancer in the parallel-plane transmission geometry. Med Phys 2016; 43(7): 4383–4485, https://doi.org/10.1118/1.4953830.
- Liu Y., Su J., Lin Z.-J., Teng S., Rhoden A., Pantong N., Liu H. Reconstructions for continuous-wave diffuse optical tomography by a globally convergent method. Journal of Applied Mathematics and Physics 2014; 2(5): 204–213, https://doi.org/10.4236/jamp.2014.25025.
- Kienle A., Lilge L., Patterson M., Hibst R., Steiner R., Wilson B. Spatially resolved absolute diffuse reflectance measurements for noninvasive determination of the optical scattering and absorption coefficients of biological tissue. Appl Opt 1996; 35(13): 2304–2314, https://doi.org/10.1364/ao.35.002304.
- Потлов А.Ю. Алгоритм локализации областей патологических нарушений в оптической структуре биологических тканей посредством импульсной диффузионной оптической томографии. Инженерный вестник Дона 2016; 2. URL: http://ivdon.ru/uploads/article/pdf/IVD_36_Potlov.pdf_3366240381.pdf.
- Proskurin S.G. Using late arriving photons for diffuse optical tomography of biological objects. Quantum Electronics 2011; 41(5): 402–406, https://doi.org/10.1070/qe2011v041n05abeh014597.
- Nichols M.G., Hull E.L., Foster T.H. Design and testing of a white-light, steady-state diffuse reflectance spectrometer for determination of optical properties of highly scattering systems. Appl Opt 1997; 36(1): 93–104, https://doi.org/10.1364/ao.36.000093.
- Pham T., Coquoz O., Fishkin J., Anderson E., Tromberg B. Broad bandwidth frequency domain instrument for quantitative tissue optical spectroscopy. Rev Sci Instrum 2000; 71(6): 2500–2513, https://doi.org/10.1063/1.1150665.
- Taroni P., Comelli D., Farina A., Pifferi A., Kienle A. Time-resolved diffuse optical spectroscopy of small tissue samples. Opt Express 2007; 15(6): 3301–3311, https://doi.org/10.1364/oe.15.003301.
- O’Sullivan T.D., Cerussi A.E., Cuccia D.J., Tromberg B.J. Diffuse optical imaging using spatially and temporally modulated light. J Biomed Opt 2012; 17(7): 071311, https://doi.org/10.1117/1.jbo.17.7.071311.
- Gibson A., Dehghani H. Diffuse optical imaging. Philos Trans A Math Phys Eng Sci 2009; 367(1900): 3055–3072, https://doi.org/10.1098/rsta.2009.0080.
- Kleshnin M., Orlova A., Kirillin M., Golubiatnikov G., Turchin I. A technique for measuring oxygen saturation in biological tissues based on diffuse optical spectroscopy. SPIE Proceedings Diffuse Optical Spectroscopy and Imaging VI 2017, https://doi.org/10.1117/12.2284378.
- Orlova A.G., Turchin I.V., Plehanov V.I., Shakhova N.M., Fiks I.I., Kleshnin M.I., Konuchenko N.Yu., Kamensky V.A. Frequency-domain diffuse optical tomography with single source-detector pair for breast cancer detection. Laser Phys Lett 2008; 5(4): 321–327, https://doi.org/10.1002/lapl.200710131.
- Fat. Hemoglobin. Melanin. URL: http://omlc.org/spectra/index.html.
- Shah N., Cerussi A., Eker C., Espinoza J., Butler J., Fishkin J., Hornung R., Tromberg B. Noninvasive functional optical spectroscopy of human breast tissue. Proc Natl Acad Sci USA 2001; 98(8): 4420–4425, https://doi.org/10.1073/pnas.071511098.
- Uddin K.M.S., Mostafa A., Anastasio M., Zhu Q. Two step imaging reconstruction using truncated pseudoinverse as a preliminary estimate in ultrasound guided diffuse optical tomography. Biomed Opt Express 2017; 8(12): 5437–5449, https://doi.org/10.1364/boe.8.005437.
- Kleshnin M.S., Orlova A.G., Kirillin M.Yu., Golubyatnikov G.Yu., Turchin I.V. Method of measuring blood oxygenation based on spectroscopy of diffusely scattered light. Quantum Electronics 2017; 47(4): 355–360, https://doi.org/10.1070/qel16284.
- Bevilacqua F., Berger A., Cerussi A., Jakubowski D., Tromberg B. Broadband absorption spectroscopy in turbid media by combined frequency-domain and steady-state methods. Appl Opt 2000; 39(34): 6498–6507, https://doi.org/10.1364/ao.39.006498.
- Esenaliev R.O., Karabutov A.A., Oraevsky A.A. Sensitivity of laser opto-acoustic imaging in detection of small deeply embedded tumors. IEEE J Sel Top Quantum Electron 1999; 5(4): 981–988, https://doi.org/10.1109/2944.796320.
- Carpenter C.M., Pogue B.W., Jiang S., Dehghani H., Wang X., Paulsen K.D., Wells W.A., Forero J., Kogel C., Weaver J.B., Poplack S.P., Kaufman P.A. Image-guided optical spectroscopy provides molecular-specific information in vivo: MRI-guided spectroscopy of breast cancer hemoglobin, water, and scatterer size. Opt Lett 2007; 32(8): 933–935, https://doi.org/10.1364/ol.32.000933.
- Bentz B.Z., Wu T.C., Gaind V., Webb K.J. Diffuse optical localization of blood vessels and 3D printing for guiding oral surgery. Appl Opt 2017; 56(23): 6649–6654, https://doi.org/10.1364/ao.56.006649.
- Payette J.R., Kohlenberg E., Leonardi L., Pabbies A., Kerr P., Liu K.Z., Sowa M.G. Assessment of skin flaps using optically based methods for measuring blood flow and oxygenation. Plast Reconstr Surg 2005; 115(2): 539–546, https://doi.org/10.1097/01.prs.0000148415.54546.ca.
- Colwell A.S., Wright L., Karanas Y. Near-infrared spectroscopy measures tissue oxygenation in free flaps for breast reconstruction. Plast Reconstr Surg 2008; 121(5): 344e–345e, https://doi.org/10.1097/prs.0b013e31816b11e5.
- Colwell A.S., Craft R.O. Near-infrared spectroscopy in autologous breast reconstruction. Clin Plast Surg 2011; 38(2): 301–307, https://doi.org/10.1016/j.cps.2011.03.014.
- Lin S., Nguyen M., Chen C., Colakoglu S., Curtis M., Tobias A.M., Lee B.T. Tissue oximetry monitoring in microsurgical breast reconstruction to decrease flap loss. Plast Reconstr Surg 2011; 127(3): 1080–1085, https://doi.org/10.1097/prs.0b013e31820436cb.
- Repež A., Oroszy D., Arnez Z.M. Continuous postoperative monitoring of cutaneous free flaps using near infrared spectroscopy. J Plast Reconstr Aesthet Surg 2008; 61(1): 71–77, https://doi.org/10.1016/j.bjps.2007.04.003.
- Whitaker I.S., Pratt G.F., Rozen W.M., Cairns S.A., Barrett M.D., Hiew L.Y., Cooper M.A., Leaper D.J. Near infrared spectroscopy for monitoring flap viability following breast reconstruction. J Reconstr Microsurg 2012; 28(3): 149–154, https://doi.org/10.1055/s-0031-1296030.
- Scheufler O., Exner K., Andresen R. Investigation of TRAM flap oxygenation and perfusion by near-infrared reflection spectroscopy and color-coded duplex sonography. Plast Reconstr Surg 2004; 113(1): 141–152, https://doi.org/10.1097/01.prs.0000095940.96294.a5.
- Heise H.M., Lampen P., Stücker M. Reflectance spectroscopy can quantify cutaneous haemoglobin oxygenation by oxygen uptake from the atmosphere after epidermal barrier disruption. Skin Res Technol 2003; 9(4): 295–298, https://doi.org/10.1034/j.1600-0846.2003.00036.x.
- Малыхина И.Ф., Неробеев А.И., Добродеев А.С., Вербо Е.В., Гарелик Е.И., Салихов К.С. Тканевая оксиметрия: оценка жизнеспособности свободных лоскутов при реконструкции головы и шеи. Вопросы реконструктивной и пластической хирургии 2015; 2(53): 11–24.
- Cong W., Intes X., Wang G. Optical tomographic imaging for breast cancer detection. J Biomed Opt 2017; 22(9): 1–6, https://doi.org/10.1117/1.jbo.22.9.096011.
- Potlov A.Yu., Frolov S.V., Proskurin S.G. Localization of inhomogeneities in diffuse optical tomography based on late arriving photons. Optics and Spectroscopy 2016; 120(1): 9–19, https://doi.org/10.1134/s0030400x1601015x.
- Konovalov A.B., Vlasov V.V., Kalintsev A.G., Kravtsenyuk O.V., Lyubimov V.V. Time-domain diffuse optical tomography using analytic statistical characteristics of photon trajectories. Quantum Electronics 2006; 36(11): 1048–1055, https://doi.org/10.1070/qe2006v036n11abeh013302.
- Yazdi H.S., O’Sullivan T.D., Leproux A., Hill B., Durkin A., Telep S., Lam J., Yazdi S.S., Police A.M., Carroll R.M., Combs F.J., Strömberg T., Yodh A.G., Tromberg B.J. Mapping breast cancer blood flow index, composition, and metabolism in a human subject using combined diffuse optical spectroscopic imaging and diffuse correlation spectroscopy. J Biomed Opt 2017; 22(4): 45003, https://doi.org/10.1117/1.jbo.22.4.045003.
- Boas D.A., Brooks D.H., Miller E.L., DiMarzio C.A., Kilmer M., Gaudette R.J., Zhang Q. Imaging the body with diffuse optical tomography. IEEE Signal Processing Magazine 2001; 18(6): 57–75, https://doi.org/10.1109/79.962278.
- Масленникова А.В., Орлова А.Г., Пряникова Т.И., Костеников Н.А., Виноградова Ю.Н., Денисенко А.Н. Клиническое значение и методы диагностики опухолевой гипоксии. Вопросы онкологии 2011; 57(4): 413–420.
- Масленникова А.В., Голубятников Г.Ю., Орлова А.Г., Плеханов В.И., Артифексова А.А., Шахова Н.М., Каменский В.А., Турчин И.В. Неинвазивный оптический метод оценки кислородного статуса новообразований молочной железы. Опухоли женской репродуктивной системы 2010; 1: 5–10.
- Ruiz J., Nouizi F., Cho J., Zheng J., Li Y., Chen J.H., Su M.Y., Gulsen G. Breast density quantification using structured-light-based diffuse optical tomography simulations. Appl Opt 2017; 56(25): 7146–7157, https://doi.org/10.1364/ao.56.007146.
- Cerussi A., Shah N., Hsiang D., Durkin A., Butler J., Tromberg B.J. In vivo absorption, scattering, and physiologic properties of 58 malignant breast tumors determined by broadband diffuse optical spectroscopy. J Biomed Opt 2006; 11(4): 044005, https://doi.org/10.1117/1.2337546.
- Zhu Q., Huang M., Chen N., Zarfos K., Jagjivan B., Kane M., Hedge P., Kurtzman S.H. Ultrasound-guided optical tomographic imaging of malignant and benign breast lesions: initial clinical results of 19 cases. Neoplasia 2003; 5(5): 379–388, https://doi.org/10.1016/s1476-5586(03)80040-4.
- Cerussi A.E., Tanamai V.W., Hsiang D., Butler J., Mehta R.S., Tromberg B.J. Diffuse optical spectroscopic imaging correlates with final pathological response in breast cancer neoadjuvant chemotherapy. Philos Trans A Math Phys Eng Sci 2011; 369(1955): 4512–4530, https://doi.org/10.1098/rsta.2011.0279.
- Jiang S., Pogue B.W., Kaufman P.A., Gui J., Jermyn M., Frazee T.E., Poplack S.P., DiFlorio-Alexander R., Wells W.A., Paulsen K.D. Predicting breast tumor response to neoadjuvant chemotherapy with diffuse optical spectroscopic tomography prior to treatment. Clin Cancer Res 2014; 20(23): 6006–6015, https://doi.org/10.1158/1078-0432.ccr-14-1415.
- Anderson P.G., Kalli S., Sassaroli A., Krishnamurthy N., Makim S.S., Graham R.A., Fantini S. Optical mammography in patients with breast cancer undergoing neoadjuvant chemotherapy. Acad Radiol 2017; 24(10): 1240–1255, https://doi.org/10.1016/j.acra.2017.03.020.
- Maslennikova A.V., Orlova A.G., Golubiatnikov G.Y., Kamensky V.A., Shakhova N.M., Babaev A.A., Snopova L.B., Ivanova I.P., Plekhanov V.I., Prianikova T.I., Turchin I.V. Comparative study of tumor hypoxia by diffuse optical spectroscopy and immunohistochemistry in two tumor models. J Biophotonics 2010; 3(12): 743–751, https://doi.org/10.1002/jbio.201000060.
- Orlova A.G., Maslennikova A.V., Golubyatnikov G.Y., Kamensky V.A., Shakhova N.M., Plekhanov V.I., Turchin I.V., Snopova L.B., Ivanova I.P., Babaev A.A., Pryanikova T.I. Noninvasive estimation of the oxygen status of experimental tumors by diffuse optical spectroscopy. Biophysics 2011; 56(2): 304–308, https://doi.org/10.1134/s0006350911020230.
- Orlova A.G., Kirillin M.Yu., Volovetsky A.В., Shilyagina N.Yu., Sergeeva E.A., Golubiatnikov G.Yu., Turchin I.V. Diffuse optical spectroscopy monitoring of oxygen state and hemoglobin concentration during SKBR-3 tumor model growth. Laser Physics Letters 2016, 14(1): 015601, https://doi.org/10.1088/1612-202x/aa4fc1.
- Niu S., Zhu Q., Jiang Y., Zhu J., Xiao M., You S., Zhou W., Xiao Yu. Correlations among ultrasound-guided diffuse optical tomography, microvessel density, and breast cancer prognosis. J Ultrasound Med 2017; 37(4): 833–842, https://doi.org/10.1002/jum.14416.
- Biswal N.C., Xu Y., Zhu Q. Imaging tumor oxyhemoglobin and deoxyhemoglobin concentrations with ultrasound-guided diffuse optical tomography. Technol Cancer Res Treat 2011; 10(5): 417–429, https://doi.org/10.7785/tcrt.2012.500219.
- Piao D., Ramadan M., Park A., Bartels K.E., Patel S.G. Freehand diffuse optical spectroscopy imaging for intraoperative identification of major venous and arterial vessels underlying peritoneal fat: an in vivo demonstration in a pig model. J Biomed Opt 2017; 22(10): 1–4, https://doi.org/10.1117/1.jbo.22.10.100503.
- Khalil M.A., Kim H.K., Kim I.K., Flexman M., Dayal R., Shrikhande G., Hielscher A.H. Dynamic diffuse optical tomography imaging of peripheral arterial disease. Biomed Opt Express 2012; 3(9): 2288–2298, https://doi.org/10.1364/boe.3.002288.
- Sujatha N., Anand B.S.S., Nivetha K.B., Narayanamurthy V.B., Seshadri V., Poddar R. Assessment of microcirculatory hemoglobin levels in normal and diabetic subjects using diffuse reflectance spectroscopy in the visible region — a pilot study. Journal of Applied Spectroscopy 2015; 82(3): 432–437, https://doi.org/10.1007/s10812-015-0125-9.
- Papazoglou E.S., Weingarten M.S., Zubkov L., Zhu L., Tyagi S., Pourezaei K. Near infrared diffuse optical tomography: improving the quality of care in chronic wounds of patients with diabetes. Biomed Instrum Technol 2007; 41(1): 83–87, https://doi.org/10.2345/0899-8205(2007)41[83:nidoti]2.0.co;2.
- Zhao H., Gao F., Tanikawa Y., Homma K., Yamada Y. Time-resolved diffuse optical tomographic imaging for the provision of both anatomical and functional information about biological tissue. Appl Opt 2005; 44(10): 1905–1916, https://doi.org/10.1364/ao.44.001905.