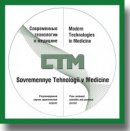
Современные перспективы разработки материалов для стабилизирующих вмешательств на позвоночнике с применением спондилодеза (обзор)
Проведена оценка эффективности применения различных пластических материалов, наиболее часто используемых для стабилизирующих вмешательств на позвоночнике с применением спондилодеза; аутотрансплантатов; алло- и ксенотрансплантатов; материалов синтетического и биологического происхождения; факторов роста; мультипотентных мезенхимальных стромальных клеток; трансплантатов, изготовленных с помощью трехмерного моделирования. Выявленные при анализе литературных данных недостатки и преимущества пластических материалов позволят наметить дальнейшие перспективные пути исследований, учитывать не только свойства биосовместимости и биоинтеграции, но и скорость репаративной регенерациии с трансформацией непосредственно в костную ткань.
- Werner B.C., Li X., Shen F.H. Stem cells in preclinical spine studies. Spine J 2014; 14(3): 542–551, https://doi.org/10.1016/j.spinee.2013.08.031.
- Amin S., Achenbach S.J., Atkinson E.J., Khosla S., Melton L.J. 3rd. Trends in fracture incidence: a population-based study over 20 years. J Bone Miner Res 2014; 29(3): 581–589, https://doi.org/10.1002/jbmr.2072.
- van Vugt T.A., Geurts J., Arts J.J. Clinical application of antimicrobial bone graft substitute in osteomyelitis treatment: a systematic review of different bone graft substitutes available in clinical treatment of osteomyelitis. Biomed Res Int 2016; 2016: 6984656, https://doi.org/10.1155/2016/6984656.
- Grémare A., Guduric V., Bareille R., Heroguez V., Latour S., L’heureux N., Fricain J.C., Catros S., Le Nihouannen D. Characterization of printed PLA scaffolds for bone tissue engineering. J Biomed Mater Res A 2018; 106(4): 887–894, https://doi.org/10.1002/jbm.a.36289.
- Oryan A., Alidadi S., Moshiri A., Maffulli N. Bone regenerative medicine: classic options, novel strategies, and future directions. J Orthop Surg Res 2014; 9(1): 18, https://doi.org/10.1186/1749-799X-9-18.
- Ehrler D.M., Vaccaro A.R. The use of allograft bone in lumbar spine surgery. Clin Orthop Relat Res 2000; 371: 38–45, https://doi.org/10.1097/00003086-200002000-00005.
- Badylak S.F., Gilbert T.W. Immune response to biologic scaffold materials. Semin Immunol 2008; 20(2): 109–116, https://doi.org/10.1016/j.smim.2007.11.003.
- Brydone A.S., Meek D., Maclaine S. Bone grafting, orthopaedic biomaterials, and the clinical need for bone engineering. Proc Inst Mech Eng H 2010; 224(12): 1329–1343, https://doi.org/10.1243/09544119jeim770.
- Athanasiou V.T., Papachristou D.J., Panagopoulos A., Saridis A., Scopa C.D., Megas P. Histological comparison of autograft, allograft-DBM, xenograft, and synthetic grafts in a trabecular bone defect: an experimental study in rabbits. Med Sci Monit 2010; 16(1): BR24–31.
- Parikh S.N. Bone graft substitutes: past, present, future. J Postgrad Med 2002; 48(2): 142–148.
- Рерих B.B., Аветисян A.P., Зайдман A.M., Ластевский А.Д., Батаев В.А., Никулина А.А. Остеоинтеграция гидроксиапатитовых гранул в телах поясничных позвонков в эксперименте. Хирургия позвоночника 2013; 4: 43–51, https://doi.org/10.14531/ss2013.4.43-51.
- Becker S., Maissen O., Ponomarev I., Stoll T., Rahn B., Wilke I. Osteopromotion by a beta-tricalcium phosphate/bone marrow hybrid implant for use in spine surgery. Spine 2006; 31(1): 11–17, https://doi.org/10.1097/01.brs.0000192762.40274.57.
- Daculsi G., Uzel A.P., Weiss P., Goyenvalle E., Aguado E. Developments in injectable multiphasic biomaterials. The performance of microporous biphasic calcium phosphate granules and hydrogels. J Mater Sci Mater Med 2009; 21(3): 855–861, https://doi.org/10.1007/s10856-009-3914-y.
- Uchida A., Araki N., Shinto Y., Yoshikawa H., Kurisaki E., Ono K. The use of calcium hydroxyapatite ceramic in bone tumour surgery. J Bone Joint Surg Br 1990; 72(2): 298–302, https://doi.org/10.1302/0301-620x.72b2.2155908.
- Albrektsson T., Johansson C. Osteoinduction, osteoconduction and osseointegration. Eur Spine J 2001; 10(Suppl 2): S96–S101, https://doi.org/10.1007/s005860100282.
- Gothard D., Smith E.L., Kanczler J.M., Rashidi H., Qutachi O., Henstock J., Rotherham M., El Haj A., Shakesheff K.M., Oreffo R.O. Tissue engineered bone using select growth factors: a comprehensive review of animal studies and clinical translation studies in man. Eur Cell Mater 2014; 28: 166–207, https://doi.org/10.22203/ecm.v028a13.
- Pape H.C., Evans A., Kobbe P. Autologous bone graft: properties and techniques. J Orthop Trauma 2010; 24(Suppl 1): S36–S40, https://doi.org/10.1097/bot.0b013e3181cec4a1.
- Greenwald A.S., Boden S.D., Goldberg V.M., Khan Y., Laurencin C.T., Rosier R.N.; American Academy of Orthopaedic Surgeons. The Committee on Biological Implants. Bone-graft substitutes: facts, fictions, and applications. J Bone Joint Surg Am 2001; 83-A(Suppl 2 Pt 2): 98–103, https://doi.org/10.2106/00004623-200100022-00007.
- Mauffrey C., Madsen M., Bowles R.J., Seligson D. Bone graft harvest site options in orthopaedic trauma: a prospective in vivo quantification study. Injury 2012; 43(3): 323–326, https://doi.org/10.1016/j.injury.2011.08.029.
- Iyer R.R., Tuite G.F., Meoded A., Carey C.C., Rodriguez L.F. A modified technique for occipitocervical fusion using compressed iliac crest allograft results in a high rate of fusion in the pediatric population. World Neurosurg 2017; 107: 342–350, https://doi.org/10.1016/j.wneu.2017.07.172.
- Goyal T., Sankineani S.R., Tripathy S.K. Local distal radius bone graft versus iliac crest bone graft for scaphoid nonunion: a comparative study. Musculoskelet Surg 2013; 97(2): 109–114, https://doi.org/10.1007/s12306-012-0219-y.
- Ebraheim N.A., Elgafy H., Xu R. Bone-graft harvesting from iliac and fibular donor sites: techniques and complications. J Am Acad Orthop Surg 2001; 9(3): 210–218, https://doi.org/10.5435/00124635-200105000-00007.
- Kim D.H., Rhim R., Li L., Martha J., Swaim B.H., Banco R.J., Jenis L.G., Tromanhauser S.G. Prospective study of iliac crest bone graft harvest site pain and morbidity. Spine J 2009; 9(11): 886–892, https://doi.org/10.1016/j.spinee.2009.05.006.
- Christensen B.B. Autologous tissue transplantations for osteochondral repair. Dan Med J 2016; 63(4): B5236.
- Dimar J.R. 2nd, Glassman S.D., Burkus J.K., Pryor P.W., Hardacker J.W., Carreon L.Y. Two-year fusion and clinical outcomes in 224 patients treated with a single-level instrumented posterolateral fusion with iliac crest bone graft. Spine J 2009; 9(11): 880–885, https://doi.org/10.1016/j.spinee.2009.03.013.
- Zamborsky R., Svec A., Bohac M., Kilian M., Kokavec M. Infection in bone allograft transplants. Exp Clin Transplant 2016; 14(5): 484–490.
- Müller M.A., Frank A., Briel M., Valderrabano V., Vavken P., Entezari V., Mehrkens A. Substitutes of structural and non-structural autologous bone grafts in hindfoot arthrodeses and osteotomies: a systematic review. BMC Musculoskelet Disord 2013; 14(1): 59, https://doi.org/10.1186/1471-2474-14-59.
- Smith C.A., Richardson S.M., Eagle M.J., Rooney P., Board T., Hoyland J.A. The use of a novel bone allograft wash process to generate a biocompatible, mechanically stable and osteoinductive biological scaffold for use in bone tissue engineering. J Tissue Eng Regen Med 2015; 9(5): 595–604, https://doi.org/10.1002/term.1934.
- Kim Y., Rodriguez A.E., Nowzari H. The risk of prion infection through bovine grafting materials. Clin Implant Dent Relat Res 2016; 18(6): 1095–1102, https://doi.org/10.1111/cid.12391.
- Mirabet V., Álvarez M., Luis-Hidalgo M., Galán J., Puig N., Larrea L., Arbona C. Detection of hepatitis B virus in bone allografts from donors with occult hepatitis B infection. Cell Tissue Bank 2017; 18(3): 335–341, https://doi.org/10.1007/s10561-017-9644-3.
- Ward W.G., Heise E., Boles C., Kiger D., Gautreaux M., Rushing J., Smith B.P., Bullard D. Human leukocyte antigen sensitization after structural cortical allograft implantations. Clin Orthop Relat Res 2005; 435: 31–35, https://doi.org/10.1097/01.blo.0000165848.43820.98.
- Gomes K.U., Carlini J.L., Biron C., Rapoport A., Dedivitis R.A. Use of allogeneic bone graft in maxillary reconstruction for installation of dental implants. J Oral Maxillofac Surg 2008; 66(11): 2335–2338, https://doi.org/10.1016/j.joms.2008.06.006.
- Allman A., McPherson T., Badylak S., Merrill L., Kallakury B., Sheehan C., Raeder R., Metzger D. Xenogeneic extracellular matrix grafts elicit a TH2-restricted immune response. Transplantation 2001; 71(11): 1631–1640, https://doi.org/10.1097/00007890-200106150-00024.
- Brown B.N., Valentin J.E., Stewart-Akers A.M., McCabe G.P., Badylak S.F. Macrophage phenotype and remodeling outcomes in response to biologic scaffolds with and without a cellular component. Biomaterials 2009; 30(8): 1482–1491, https://doi.org/10.1016/j.biomaterials.2008.11.040.
- Valentin J.E., Stewart-Akers A.M., Gilbert T.W., Badylak S.F. Macrophage participation in the degradation and remodeling of extracellular matrix scaffolds. Tissue Eng Part A 2009; 15(7): 1687–1694, https://doi.org/10.1089/ten.tea.2008.0419.
- Keating J.F., McQueen M.M. Substitutes for autologous bone graft in orthopaedic trauma. J Bone Joint Surg Br 2001; 8 3(1): 3–8, https://doi.org/10.1302/0301-620x.83b1.11952.
- Malinin T., Temple H.T. Comparison of frozen and freeze-dried particulate bone allografts. Cryobiology 2007; 55(2): 167–170, https://doi.org/10.1016/j.cryobiol.2007.05.007.
- Kurien T., Pearson R.G., Scammell B.E. Bone graft substitutes currently available in orthopaedic practice: the evidence for their use. Bone Joint J 2013; 95-B(5): 583–597, https://doi.org/10.1302/0301-620x.95b5.30286.
- Elder B.D., Eleswarapu S.V., Athanasiou K.A. Extraction techniques for the decellularization of tissue engineered articular cartilage constructs. Biomaterials 2009; 30(22): 3749–3756, https://doi.org/10.1016/j.biomaterials.2009.03.050.
- Vavken P., Joshi S., Murray M.M. TRITON-X is most effective among three decellularization agents for ACL tissue engineering. J Orthop Res 2009; 27(12): 1612–1618, https://doi.org/10.1002/jor.20932.
- Gui L., Chan S.A., Breuer C.K., Niklason L.E. Novel utilization of serum in tissue decellularization. Tissue Eng Part C Methods 2010; 16(2): 173–184, https://doi.org/10.1089/ten.tec.2009.0120.
- Fölsch C., Mittelmeier W., Bilderbeek U., Timmesfeld N., von Garrel T., Matter P.H. Effect of storage temperature on allograft bone. Transfus Med Hemother 2012; 39(1): 36–40, https://doi.org/10.1159/000335647.
- Sandhu H.S., Khan S.N., Suh D.Y., Boden S.D. Demineralized bone matrix, bone morphogenetic proteins, and animal models of spine fusion: an overview. Eur Spine J 2001; 10(Suppl 2): S122–S131, https://doi.org/10.1007/s005860100303.
- Fernandez de Grado G., Keller L., Idoux-Gillet Y., Wagner Q., Musset A.M., Benkirane-Jessel N., Bornert F., Offner D. Bone substitutes: a review of their characteristics, clinical use, and perspectives for large bone defects management. J Tissue Eng 2018; 9: 2041731418776819, https://doi.org/10.1177/2041731418776819.
- Miyazaki M., Tsumura H., Wang J.C., Alanay A. An update on bone substitutes for spinal fusion. Eur Spine J 2009; 18(6): 783–799, https://doi.org/10.1007/s00586-009-0924-x.
- Buser Z., Brodke D.S., Youssef J.A., Rometsch E., Park J.B., Yoon S.T., Wang J.C., Meisel H.J. Allograft versus demineralized bone matrix in instrumented and noninstrumented lumbar fusion: a systematic review. Global Spine J 2018; 8(4): 396–412, https://doi.org/10.1177/2192568 217735342.
- Cornell C.N., Lane J.M. Current understanding of osteoconduction in bone regeneration. Clin Orthop Relat Res 1998; 355(Suppl): S267–S273, https://doi.org/10.1097/00003086-199810001-00027.
- Кирилова И.А. Деминерализованный костный трансплантат как стимулятор остеогенеза: современные концепции. Хирургия позвоночника 2004; 3: 105–110.
- Ivchenko V.K., Fadeev G., Pikaliuk V.S., Opalinskaia L. The stimulation of reparative regeneration with demineralized bone matrix in puncture osteoplasty operations for bone cysts in children. Vestnik hirurgii im. I.I. Grekova 1994; 153(7–12): 83–86.
- Kinney R.C., Ziran B.H., Hirshorn K., Schlatterer D., Ganey T. Demineralized bone matrix for fracture healing: fact or fiction? J Orthop Trauma 2010; 24(Suppl 1): S52–S55, https://doi.org/10.1097/bot.0b013e3181d07ffa.
- Campana V., Milano G., Pagano E., Barba M., Cicione C., Salonna G., Lattanzi W., Logroscino G. Bone substitutes in orthopaedic surgery: from basic science to clinical practice. J Mater Sci Mater Med 2014; 25(10): 2445–2461, https://doi.org/10.1007/s10856-014-5240-2.
- Folkman J., Hochberg M. Self-regulation of growth in three dimensions. J Exp Med 1973; 138(4): 745–753, https://doi.org/10.1084/jem.138.4.745.
- Lokmic Z., Stillaert F., Morrison W.A., Thompson E.W., Mitchell G.M. An arteriovenous loop in a protected space generates a permanent, highly vascular, tissue-engineered construct. FASEB J 2007; 21(2): 511–522, https://doi.org/10.1096/fj.06-6614com.
- Bigham A.S., Dehghani S.N., Shafiei Z., Torabi Nezhad S. Xenogenic demineralized bone matrix and fresh autogenous cortical bone effects on experimental bone healing: radiological, histopathological and biomechanical evaluation. J Orthop Traumatol 2008; 9(2): 73–80, https://doi.org/10.1007/s10195-008-0006-6.
- Pastorino L., Dellacasa E., Scaglione S., Giulianelli M., Sbrana F., Vassalli M., Ruggiero C. Oriented collagen nanocoatings for tissue engineering. Colloids Surf B Biointerfaces 2014; 114: 372–378, https://doi.org/10.1016/j.colsurfb.2013.10.026.
- Hamilton P.T., Jansen M.S., Ganesan S., Benson R.E., Hyde-Deruyscher R., Beyer W.F., Gile J.C., Nair S.A., Hodges J.A., Grøn H. Improved bone morphogenetic protein-2 retention in an injectable collagen matrix using bifunctional peptides. PLoS One 2013; 8(8): e70715, https://doi.org/10.1371/journal.pone.0070715.
- Amruthwar S.S., Janorkar A.V. In vitro evaluation of elastin-like polypeptide-collagen composite scaffold for bone tissue engineering. Dent Mater 2013; 29(2): 211–220, https://doi.org/10.1016/j.dental.2012.10.003.
- Thula T.T., Rodriguez D.E., Lee M.H., Pendi L., Podschun J., Gower L.B. In vitro mineralization of dense collagen substrates: a biomimetic approach toward the development of bone-graft materials. Acta Biomater 2011; 7(8): 3158–3169, https://doi.org/10.1016/j.actbio.2011.04.014.
- Inzana J.A., Olvera D., Fuller S.M., Kelly J.P., Graeve O.A., Schwarz E.M., Kates S.L., Awad H.A. 3D printing of composite calcium phosphate and collagen scaffolds for bone regeneration. Biomaterials 2014; 35(13): 4026–4034, https://doi.org/10.1016/j.biomaterials.2014.01.064.
- Perez R.A., Kim M., Kim T.H., Kim J.H., Lee J.H., Park J.H., Knowles J.C., Kim H.W. Utilizing core-shell fibrous collagen-alginate hydrogel cell delivery system for bone tissue engineering. Tissue Eng Part A 2014; 20(1–2): 103–114, https://doi.org/10.1089/ten.tea.2013.0198.
- Aravamudhan A., Ramos D.M., Nip J., Harmon M.D., James R., Deng M., Laurencin C.T., Yu X., Kumbar S.G. Cellulose and collagen derived micro-nano structured scaffolds for bone tissue engineering. J Biomed Nanotechnol 2013; 9(4): 719–731, https://doi.org/10.1166/jbn.2013.1574.
- LogithKumar R., KeshavNarayan A., Dhivya S., Chawla A., Saravanan S., Selvamurugan N. A review of chitosan and its derivatives in bone tissue engineering. Carbohydr Polym 2016; 151: 172–188, https://doi.org/10.1016/j.carbpol.2016.05.049.
- Venkatesan J., Anil S., Kim S.K., Shim M.S. Chitosan as a vehicle for growth factor delivery: various preparations and their applications in bone tissue regeneration. Int J Biol Macromol 2017; 104(Pt B): 1383–1397, https://doi.org/10.1016/j.ijbiomac.2017.01.072.
- Лекишвили М.В., Балберкин А.В., Колондаев А.Ф., Васильев М.Г., Баранецкий А.Л., Буклемишев Ю.В. Первый опыт применения в клинике костной патологии биокомпозиционного материала «Остеоматрикс». Вестник травматологии и ортопедии им. Н.Н. Приорова 2002; 4: 80–83.
- Дунаев М.В., Китаев В.А., Матавкина М.В., Дружинин А.Е., Бубнов А.С. Сравнительный анализ и клинический опыт использования остеопластических материалов на основе недеминерализованного костного коллагена и искусственного гидроксиапатита при закрытии костных дефектов в амбулаторной хирургической стоматологии. Вестник Российской академии медицинских наук 2014; 69(7–8): 112–120, https://doi.org/10.15690/vramn.v69i7-8.1117.
- Сирак С.В., Козиева И.Э., Мартиросян А.К. Клинико-экспериментальное использование остеопластических материалов в сочетании с электомагнитным излучением для ускорения регенерации костных дефектов челюстей. Фундаментальные исследования 2013; 5–2: 389–393.
- Красножен В.Н., Покровская Е.М., Михалин А.Н. Клиническое обоснование применения полимерных имплантов в восстановлении костных дефектов околоносовых пазух. Российская ринология 2013; 21(2): 12–13.
- Покровская Е.М. Использование полимерных имплантов в реконструктивной хирургии околоносовых пазух (экспериментальное исследование). Известия Самарского научного центра Российской академии наук. Социальные, гуманитарные, медико-биологические науки 2014; 16(5–4): 1415–1417.
- Patlolla A., Arinzeh T.L. Evaluating apatite formation and osteogenic activity of electrospun composites for bone tissue engineering. Biotechnol Bioeng 2014; 111(5): 1000–1017, https://doi.org/10.1002/bit.25146.
- Galois L., Mainard D., Delagoutte J.P. Beta-tricalcium phosphate ceramic as a bone substitute in orthopaedic surgery. Int Orthop 2002; 26(2): 109–115, https://doi.org/10.1007/s00264-001-0329-x.
- Gaasbeek R.D., Toonen H.G., van Heerwaarden R.J., Buma P. Mechanism of bone incorporation of beta-TCP bone substitute in open wedge tibial osteotomy in patients. Biomaterials 2005; 26(33): 6713–6719, https://doi.org/10.1016/j.biomaterials.2005.04.056.
- Koerten H.K., van der Meulen J. Degradation of calcium phosphate ceramics. J Biomed Mater Res 1999; 44(1): 78–86, https://doi.org/10.1002/(sici)1097-4636(199901)44:178::aid-jbm93.0.co;2-6 .
- Malhotra A., Habibovic P. Calcium phosphates and angiogenesis: implications and advances for bone regeneration. Trends Biotechnol 2016; 34(12): 983–992, https://doi.org/10.1016/j.tibtech.2016.07.005.
- Bohner M. Calcium orthophosphates in medicine: from ceramics to calcium phosphate cements. Injury 2000; 31(Suppl 4): 37–47, https://doi.org/10.1016/s0020-1383(00)80022-4.
- Chazono M., Tanaka T., Komaki H., Fujii K. Bone formation and bioresorption after implantation of injectable beta-tricalcium phosphate granules-hyaluronate complex in rabbit bone defects. J Biomed Mater Res A 2004; 70(4): 542–549, https://doi.org/10.1002/jbm.a.30094.
- Spivak J.M., Hasharoni A. Use of hydroxyapatite in spine surgery. Eur Spine J 2001; 10(Suppl 2): S197–S204, https://doi.org/10.1007/s005860100286.
- Bansal R., Patil S., Chaubey K.K., Thakur R.K., Goyel P. Clinical evaluation of hydroxyapatite and β-tricalcium phosphate composite graft in the treatment of intrabony periodontal defect: a clinico-radiographic study. J Indian Soc Periodontol 2014; 18(5): 610–617, https://doi.org/10.4103/0972-124x.142455.
- Rahaman M.N., Day D.E., Bal B.S., Fu Q., Jung S.B., Bonewald L.F., Tomsia A.P. Bioactive glass in tissue engineering. Acta Biomater 2011; 7(6): 2355–2373, https://doi.org/10.1016/j.actbio.2011.03.016.
- El-Fiqi A., Lee J.H., Lee E.J., Kim H.W. Collagen hydrogels incorporated with surface-aminated mesoporous nanobioactive glass: improvement of physicochemical stability and mechanical properties is effective for hard tissue engineering. Acta Biomater 2013; 9(12): 9508–9521, https://doi.org/10.1016/j.actbio.2013.07.036.
- Silva A.R., Paula A.C., Martins T.M., Goes A.M., Pereria M.M. Synergistic effect between bioactive glass foam and a perfusion bioreactor on osteogenic differentiation of human adipose stem cells. J Biomed Mater Res A 2014; 102(3): 818–827, https://doi.org/10.1002/jbm.a.34758.
- van Gestel N.A., Geurts J., Hulsen D.J., van Rietbergen B., Hofmann S., Arts J.J. Clinical applications of S53P4 bioactive glass in bone healing and osteomyelitic treatment: a literature review. Biomed Res Int 2015; 2015: 684826, https://doi.org/10.1155/2015/684826.
- Leppäranta O., Vaahtio M., Peltola T., Zhang D., Hupa L., Hupa M., Ylänen H., Salonen J.I., Viljanen M.K., Eerola E. Antibacterial effect of bioactive glasses on clinically important anaerobic bacteria in vitro. J Mater Sci Mater Med 2008; 19(2): 547–551, https://doi.org/10.1007/s10856-007-3018-5.
- Zhang D., Leppäranta O., Munukka E., Ylänen H., Viljanen M.K., Eerola E., Hupa M., Hupa L. Antibacterial effects and dissolution behavior of six bioactive glasses. J Biomed Mater Res A 2010; 93(2): 475–483, https://doi.org/10.1002/jbm.a.32564.
- Félix Lanao R.P., Jonker A.M., Wolke J.G., Jansen J.A., van Hest J.C., Leeuwenburgh S.C. Physicochemical properties and applications of poly(lactic-co-glycolic acid) for use in bone regeneration. Tissue Eng Part B Rev 2013; 19(4): 380–390, https://doi.org/10.1089/ten.teb.2012.0443.
- Yoshioka T., Kawazoe N., Tateishi T., Chen G. In vitro evaluation of biodegradation of poly (lactic-co-glycolic acid) sponges. Biomaterials 2008; 29(24–25): 3438–3443, https://doi.org/10.1016/j.biomaterials.2008.04.011.
- Félix Lanao R.P., Leeuwenburgh S.C., Wolke J.G., Jansen J.A. In vitro degradation rate of apatitic calcium phosphate cement with incorporated PLGA microspheres. Acta Biomater 2011; 7(9): 3459–3468, https://doi.org/10.1016/j.actbio.2011.05.036.
- Wang J., Yu X. Preparation, characterization and in vitro analysis of novel structured nanofibrous scaffolds for bone tissue engineering. Acta Biomater 2010; 6(8): 3004–3012, https://doi.org/10.1016/j.actbio.2010.01.045.
- Polo-Corrales L., Latorre-Esteves M., Ramirez-Vick J.E. Scaffold design for bone regeneration. J Nanosci Nanotechnol 2014; 14(1): 15–56, https://doi.org/10.1166/jnn.2014.9127.
- Hannink G., Arts J.J. Bioresorbability, porosity and mechanical strength of bone substitutes: what is optimal for bone regeneration? Injury 2011; 42(Suppl 2): S22–S25, https://doi.org/10.1016/j.injury.2011.06.008.
- Neman J., Hambrecht A., Cadry C., Jandial R. Stem cell-mediated osteogenesis: therapeutic potential for bone tissue engineering. Biologics 2012; 6: 47–57, https://doi.org/10.2147/btt.s22407.
- Karageorgiou V., Kaplan D. Porosity of 3D biomaterial scaffolds and osteogenesis. Biomaterials 2005; 26(27): 5474–5491, https://doi.org/10.1016/j.biomaterials.2005.02.002.
- Tsuruga E., Takita H., Itoh H., Wakisaka Y., Kuboki Y. Pore size of porous hydroxyapatite as the cell-substratum controls BMP-induced osteogenesis. J Biochem 1997; 121(2): 317–324, https://doi.org/10.1093/oxfordjournals.jbchem.a021589.
- Itälä A.I., Ylänen H.O., Ekholm C., Karlsson K.H., Aro H.T. Pore diameter of more than 100 micron is not requisite for bone ingrowth in rabbits. J Biomed Mater Res 2001; 58(6): 679–683, https://doi.org/10.1002/jbm.1069.
- Blokhuis T.J., Termaat M.F., den Boer F.C., Patka P., Bakker F.C., Haarman H.J. Properties of calcium phosphate ceramics in relation to their in vivo behavior. J Trauma 2000; 48(1): 179–186, https://doi.org/10.1097/00005373-200001000-00037.
- Qi X., Pei P., Zhu M., Du X., Xin C., Zhao S., Li X., Zhu Y. Three dimensional printing of calcium sulfate and mesoporous bioactive glass scaffolds for improving bone regeneration in vitro and in vivo. Sci Rep 2017; 7(1): 42556, https://doi.org/10.1038/srep42556.
- Lee J.W., Kang K.S., Lee S.H., Kim J.Y., Lee B.K., Cho D.W. Bone regeneration using a microstereolithography-produced customized poly(propylene fumarate)/diethyl fumarate photopolymer 3D scaffold incorporating BMP-2 loaded PLGA microspheres. Biomaterials 2011; 32(3): 744–752, https://doi.org/10.1016/j.biomaterials.2010.09.035.
- Wiria F.E., Leong K.F., Chua C.K., Liu Y. Poly-epsilon-caprolactone/hydroxyapatite for tissue engineering scaffold fabrication via selective laser sintering. Acta Biomater 2007; 3(1): 1–12, https://doi.org/10.1016/j.actbio.2006.07.008.
- Fielding G.A., Bandyopadhyay A., Bose S. Effects of silica and zinc oxide doping on mechanical and biological properties of 3D printed tricalcium phosphate tissue engineering scaffolds. Dent Mater 2012; 28(2): 113–122, https://doi.org/10.1016/j.dental.2011.09.010.
- Park S.A., Lee S.H., Kim W.D. Fabrication of porous polycaprolactone/hydroxyapatite (PCL/HA) blend scaffolds using a 3D plotting system for bone tissue engineering. Bioprocess Biosyst Eng 2011; 34(4): 505–513, https://doi.org/10.1007/s00449-010-0499-2.
- Lieder R., Sigurjonsson O.E. The effect of recombinant human interleukin-6 on osteogenic differentiation and YKL-40 expression in human, bone marrow-derived mesenchymal stem cells. Biores Open Access 2014; 3(1): 29–34, https://doi.org/10.1089/biores.2013.0035.
- Bueno E.M., Glowacki J. Cell-free and cell-based approaches for bone regeneration. Nat Rev Rheumatol 2009; 5(12): 685–697, https://doi.org/10.1038/nrrheum.2009.228.
- Kiernan C.H., Wolvius E.B., Brama P.A.J., Farrell E. The immune response to allogeneic differentiated mesenchymal stem cells in the context of bone tissue engineering. Tissue Eng Part B Rev 2018; 24(1): 75–83, https://doi.org/10.1089/ten.teb.2017.0175.
- Granero-Moltó F., Weis J.A., Miga M.I., Landis B., Myers T.J., O’Rear L., Longobardi L., Jansen E.D., Mortlock D.P., Spagnoli A. Regenerative effects of transplanted mesenchymal stem cells in fracture healing. Stem Cells 2009; 27(8): 1887–1898, https://doi.org/10.1002/stem.103.
- Bartholomew A., Polchert D., Szilagyi E., Douglas G.W., Kenyon N. Mesenchymal stem cells in the induction of transplantation tolerance. Transplantation 2009; 87 (9 Suppl): S55–S57, https://doi.org/10.1097/tp.0b013e3181a287e6.
- Le Blanc K., Frassoni F., Ball L., Locatelli F., Roelofs H., Lewis I., Lanino E., Sundberg B., Bernardo M.E., Remberger M., Dini G., Egeler R.M., Bacigalupo A., Fibbe W., Ringdén O.; Developmental Committee of the European Group for Blood and Marrow Transplantation. Mesenchymal stem cells for treatment of steroid-resistant, severe, acute graft-versus-host disease: a phase II study. Lancet 2008; 371(9624): 1579–1586, https://doi.org/10.1016/s0140-6736(08)60690-x.
- Qin Y., Guan J., Zhang C. Mesenchymal stem cells: mechanisms and role in bone regeneration. Postgrad Med J 2014; 90(1069): 643–647, https://doi.org/10.1136/postgradmedj-2013-132387.
- Tang Z.B., Cao J.K., Wen N., Wang H.B., Zhang Z.W., Liu Z.Q., Zhou J., Duan C.M., Cui F.Z., Wang C.Y. Posterolateral spinal fusion with nano-hydroxyapatite-collagen/PLA composite and autologous adipose-derived mesenchymal stem cells in a rabbit model. J Tissue Eng Regen Med 2012; 6(4): 325–336, https://doi.org/10.1002/term.445.
- Lee T.H., Huang Y.H., Chang N.K., Lin W.C., Chien P.W., Su T.M., Hsieh D.J., Lee T.C. Characterization and spinal fusion effect of rabbit mesenchymal stem cells. BMC Res Notes 2013; 6(1): 528, https://doi.org/10.1186/1756-0500-6-528.
- Liu G., Zhao L., Zhang W., Cui L., Liu W., Cao Y. Repair of goat tibial defects with bone marrow stromal cells and beta-tricalcium phosphate. J Mater Sci Mater Med 2008; 19(6): 2367–2376, https://doi.org/10.1007/s10856-007-3348-3.
- Giannoni P., Mastrogiacomo M., Alini M., Pearce S.G., Corsi A., Santolini F., Muraglia A., Bianco P., Cancedda R. Regeneration of large bone defects in sheep using bone marrow stromal cells. J Tissue Eng Regen Med 2008; 2(5): 253–262, https://doi.org/10.1002/term.90.
- Dumas A., Moreau M.F., Ghérardi R.K., Baslé M.F., Chappard D. Bone grafts cultured with bone marrow stromal cells for the repair of critical bone defects: an experimental study in mice. J Biomed Mater Res A 2009; 90(4): 1218–1229, https://doi.org/10.1002/jbm.a.32176.
- Xu J.Z., Qin H., Wang X.Q., Zhou Q., Luo F., Hou T.Y., He Q.Y. Repair of large segmental bone defects using bone marrow stromal cells with demineralized bone matrix. Orthop Surg 2009; 1(1): 34–41, https://doi.org/10.1111/j.2757-7861.2008.00007.x.
- Nair M.B., Varma H.K., Menon K.V., Shenoy S.J., John A. Reconstruction of goat femur segmental defects using triphasic ceramic-coated hydroxyapatite in combination with autologous cells and platelet-rich plasma. Acta Biomater 2009; 5(5): 1742–1755, https://doi.org/10.1016/j.actbio.2009.01.009.
- Chang S.C., Lin T.M., Chung H.Y., Chen P.K., Lin F.H., Lou J., Jeng L.B. Large-scale bicortical skull bone regeneration using ex vivo replication-defective adenoviral-mediated bone morphogenetic protein-2 gene-transferred bone marrow stromal cells and composite biomaterials. Neurosurgery 2009; 65(6 Suppl): 75–81, https://doi.org/10.1227/01.neu.0000345947.33730.91.
- Chang S.C., Chung H.Y., Tai C.L., Chen P.K., Lin T.M., Jeng L.B. Repair of large cranial defects by hBMP-2 expressing bone marrow stromal cells: comparison between alginate and collagen type I systems. J Biomed Mater Res A 2010; 94(2): 433–441, https://doi.org/10.1002/jbm.a.32685.
- Xiao C., Zhou H., Ge S., Tang T., Hou H., Luo M., Fan X. Repair of orbital wall defects using biocoral scaffolds combined with bone marrow stem cells enhanced by human bone morphogenetic protein-2 in a canine model. Int J Mol Med 2010; 26(4): 517–525, https://doi.org/10.3892/ijmm_00000494.
- Zhou H., Deng Y., Bi X., Xiao C., Wang Y., Sun J., Gu P., Fan X. Orbital wall repair in canines with beta-tricalcium phosphate and induced bone marrow stromal cells. J Biomed Mater Res B Appl Biomater 2013; 101(8): 1340–1349, https://doi.org/10.1002/jbm.b.32951.
- Gardel L., Afonso M., Frias C., Gomes M., Reis R. Assessing the repair of critical size bone defects performed in a goat tibia model using tissue-engineered constructs cultured in a bidirectional flow perfusion bioreactor. J Biomater Appl 2014; 29(2): 172–185, https://doi.org/10.1177/0885328213519351.
- Fernandes M.B., Guimarães J.A., Casado P.L., Cavalcanti Ados S., Gonçalves N.N., Ambrósio C.E., Rodrigues F., Pinto A.C., Miglino M.A., Duarte M.E. The effect of bone allografts combined with bone marrow stromal cells on the healing of segmental bone defects in a sheep model. BMC Vet Res 2014; 10(1): 36, https://doi.org/10.1186/1746-6148-10-36.
- Ronca A., Guarino V., Raucci M.G., Salamanna F., Martini L., Zeppetelli S., Fini M., Kon E., Filardo G., Marcacci M., Ambrosio L. Large defect-tailored composite scaffolds for in vivo bone regeneration. J Biomater Appl 2014; 29(5): 715–727, https://doi.org/10.1177/0885328214539823.
- Quarto R., Mastrogiacomo M., Cancedda R., Kutepov S.M., Mukhachev V., Lavroukov A., Kon E., Marcacci M. Repair of large bone defects with the use of autologous bone marrow stromal cells. N Engl J Med 2001; 344(5): 385–386, https://doi.org/10.1056/nejm200102013440516.
- Marcacci M., Kon E., Moukhachev V., Lavroukov A., Kutepov S., Quarto R., Mastrogiacomo M., Cancedda R. Stem cells associated with macroporous bioceramics for long bone repair: 6- to 7-year outcome of a pilot clinical study. Tissue Eng 2007; 13(5): 947–955, https://doi.org/10.1089/ten.2006.0271.
- Tolar J., Nauta A.J., Osborn M.J., Panoskaltsis Mortari A., McElmurry R.T., Bell S., Xia L., Zhou N., Riddle M., Schroeder T.M., Westendorf J.J., McIvor R.S., Hogendoorn P.C., Szuhai K., Oseth L., Hirsch B., Yant S.R., Kay M.A., Peister A., Prockop D.J., Fibbe W.E., Blazar B.R. Sarcoma derived from cultured mesenchymal stem cells. Stem Cells 2007; 25(2): 371–379, https://doi.org/10.1634/stemcells.2005-0620.
- Tasso R., Augello A., Carida’ M., Postiglione F., Tibiletti M.G., Bernasconi B., Astigiano S., Fais F., Truini M., Cancedda R., Pennesi G. Development of sarcomas in mice implanted with mesenchymal stem cells seeded onto bioscaffolds. Carcinogenesis 2009; 30(1): 150–157, https://doi.org/10.1093/carcin/bgn234.
- Hustedt J.W., Jegede K.A., Badrinath R., Bohl D.D., Blizzard D.J., Grauer J.N. Optimal aspiration volume of vertebral bone marrow for use in spinal fusion. Spine J 2013; 13(10): 1217–1222, https://doi.org/10.1016/j.spinee.2013.07.435.
- Liu H.C., E L.L., Wang D.S., Su F., Wu X., Shi Z.P., Lv Y., Wang J.Z. Reconstruction of alveolar bone defects using bone morphogenetic protein 2 mediated rabbit dental pulp stem cells seeded on nano-hydroxyapatite/collagen/poly(L-lactide). Tissue Eng Part A 2011; 17(19–20): 2417–2433, https://doi.org/10.1089/ten.tea.2010.0620.
- Lavery K., Swain P., Falb D., Alaoui-Ismaili M.H. BMP-2/4 and BMP-6/7 differentially utilize cell surface receptors to induce osteoblastic differentiation of human bone marrow-derived mesenchymal stem cells. J Biol Chem 2008; 283(30): 20948–20958, https://doi.org/10.1074/jbc.m800850200.
- Egermann M., Lill C.A., Griesbeck K., Evans C.H., Robbins P.D., Schneider E., Baltzer A.W. Effect of BMP-2 gene transfer on bone healing in sheep. Gene Ther 2006; 13(17): 1290–1299, https://doi.org/10.1038/sj.gt.3302785.
- Lee S.S., Huang B.J., Kaltz S.R., Sur S., Newcomb C.J., Stock S.R., Shah R.N., Stupp S.I. Bone regeneration with low dose BMP-2 amplified by biomimetic supramolecular nanofibers within collagen scaffolds. Biomaterials 2013, 34(2): 452–459, https://doi.org/10.1016/j.biomaterials.2012.10.005.
- Yang F., Wang J., Hou J., Guo H., Liu C. Bone regeneration using cell-mediated responsive degradable PEG-based scaffolds incorporating with rhBMP-2. Biomaterials 2013; 34(5): 1514–1528, https://doi.org/10.1016/j.biomaterials.2012.10.058.
- Cha J.K., Lee J.S., Kim M.S., Choi S.H., Cho K.S., Jung U.W. Sinus augmentation using BMP-2 in a bovine hydroxyapatite/collagen carrier in dogs. J Clin Periodontol 2014; 41(1): 86–93, https://doi.org/10.1111/jcpe.12174.
- Jang J.W., Yun J.H., Lee K.I., Jang J.W., Jung U.W., Kim C.S., Choi S.H., Cho K.S. Osteoinductive activity of biphasic calcium phosphate with different rhBMP-2 doses in rats. Oral Surg Oral Med Oral Pathol Oral Radiol 2012; 113(4): 480–487, https://doi.org/10.1016/j.tripleo.2011.04.013.
- Schwender J.D., Holly L., Rouben D.P., Foley K.T. Minimally invasive transforaminal lumbar interbody fusion (TLIF): technical feasibility and initial results. J Spinal Disord Tech 2005; 18(S1): S1–S6, https://doi.org/10.1097/01.bsd.0000132291.50455.d0.
- Lewandrowski K.U., Nanson C., Calderon R. Vertebral osteolysis after posterior interbody lumbar fusion with recombinant human bone morphogenetic protein 2: a report of five cases. Spine J 2007; 7(5): 609–614, https://doi.org/10.1016/j.spinee.2007.01.011.
- Shields L.B., Raque G.H., Glassman S.D., Campbell M., Vitaz T., Harpring J., Shields C.B. Adverse effects associated with high-dose recombinant human bone morphogenetic protein-2 use in anterior cervical spine fusion. Spine 2006; 31(5): 542–547, https://doi.org/10.1097/01.brs.0000201424.27509.72.
- Vaidya R., Weir R., Sethi A., Meisterling S., Hakeos W., Wybo C.D. Interbody fusion with allograft and rhBMP-2 leads to consistent fusion but early subsidence. J Bone Joint Surg Br 2007; 89(3): 342–345, https://doi.org/10.1302/0301-620x.89b3.18270.
- Mesfin A., Buchowski J.M., Zebala L.P., Bakhsh W.R., Aronson A.B., Fogelson J.L., Hershman S., Kim H.J., Ahmad A., Bridwell K.H. High-dose rhBMP-2 for adults: major and minor complications: a study of 502 spine cases. J Bone Joint Surg Am 2013; 95(17): 1546–1553, https://doi.org/10.2106/jbjs.l.01730.
- Moshel Y.A., Hernandez E.I., Kong L., Liu C., Samadani U. Acute renal insufficiency, supraventricular tachycardia, and confusion after recombinant human bone morphogenetic protein-2 implantation for lumbosacral spine fusion. J Neurosurg Spine 2008; 8(6): 589–593, https://doi.org/10.3171/spi/2008/8/6/589.
- Fu R., Selph S., McDonagh M., Peterson K., Tiwari A., Chou R., Helfand M. Effectiveness and harms of recombinant human bone morphogenetic protein-2 in spine fusion: a systematic review and meta-analysis. Ann Intern Med 2013; 158(12): 890–902, https://doi.org/10.7326/0003-4819-158-12-201306180-00006.
- Guo X., Zheng Q., Kulbatski I., Yuan Q., Yang S., Shao Z., Wang H., Xiao B., Pan Z., Tang S. Bone regeneration with active angiogenesis by basic fibroblast growth factor gene transfected mesenchymal stem cells seeded on porous beta-TCP ceramic scaffolds. Biomed Mater 2006; 1(3): 93–99, https://doi.org/10.1088/1748-6041/1/3/001.
- Maehara H., Sotome S., Yoshii T., Torigoe I., Kawasaki Y., Sugata Y., Yuasa M., Hirano M., Mochizuki N., Kikuchi M., Shinomiya K., Okawa A. Repair of large osteochondral defects in rabbits using porous hydroxyapatite/collagen (HAp/Col) and fibroblast growth factor-2 (FGF-2). J Orthop Res 2010; 28(5): 677–686, https://doi.org/10.1002/jor.21032.
- Hollinger J.O., Hart C.E., Hirsch S.N., Lynch S., Friedlaender G.E. Recombinant human platelet-derived growth factor: biology and clinical applications. J Bone Joint Surg Am 2008; 90(Suppl 1): 48–54, https://doi.org/10.2106/jbjs.g.01231.
- Lieberman J.R., Daluiski A., Einhorn T.A. The role of growth factors in the repair of bone. Biology and clinical applications. J Bone Joint Surg Am 2002; 84(6): 1032–1044, https://doi.org/10.2106/00004623-200206000-00022.
- Graham S., Leonidou A., Lester M., Heliotis M., Mantalaris A., Tsiridis E. Investigating the role of PDGF as a potential drug therapy in bone formation and fracture healing. Expert Opin Investig Drugs 2009; 18(11): 1633–1654, https://doi.org/10.1517/13543780903241607.
- Luvizuto E.R., Tangl S., Dobsak T., Reich K., Gruber R., Sonoda C.K., Okamoto R. Effect of recombinant PDGF-BB on bone formation in the presence of β-tricalcium phosphate and bovine bone mineral matrix: a pilot study in rat calvarial defects. BMC Oral Health 2016; 16(1): 52, https://doi.org/10.1186/s12903-016-0210-3.
- Zhao L., Jiang S., Hantash B.M. Transforming growth factor β1 induces osteogenic differentiation of murine bone marrow stromal cells. Tissue Eng Part A 2010; 16(2): 725–733, https://doi.org/10.1089/ten.tea.2009.0495.
- Kwok S., Partridge N.C., Srinivasan N., Nair S.V., Selvamurugan N. Mitogen-activated protein kinase-dependent inhibition of osteocalcin gene expression by transforming growth factor-β1. J Cell Biochem 2009; 106(1): 161–169, https://doi.org/10.1002/jcb.21991.
- Zhang H., Ahmad M., Gronowicz G. Effects of transforming growth factor-β1 (TGF-β1) on in vitro mineralization of human osteoblasts on implant materials. Biomaterials 2003; 24(12): 2013–2020, https://doi.org/10.1016/s0142-9612(02)00616-6.
- Alliston T., Choy L., Ducy P., Karsenty G., Derynck R. TGF-β-induced repression of CBFA1 by Smad3 decreases cbfa1 and osteocalcin expression and inhibits osteoblast differentiation. EMBO J 2001; 20(9): 2254–2272, https://doi.org/10.1093/emboj/20.9.2254.
- Kaji H., Naito J., Sowa H., Sugimoto T., Chihara K. Smad3 differently affects osteoblast differentiation depending upon its differentiation stage. Horm Metab Res 2006: 38(11): 740–745, https://doi.org/10.1055/s-2006-955085.
- Centrella M., Ji C., Casinghino S., McCarthy T.L. Rapid flux in transforming growth factor-β receptors on bone cells. J Biol Chem 1996; 271(31): 18616–18622, https://doi.org/10.1074/jbc.271.31.18616.
- Ochiai H., Yamamoto Y., Yokoyama A., Yamashita H., Matsuzaka K., Abe S., Azuma T. Dual nature of TGF-β1 in osteoblastic differentiation of human periodontal ligament cells. J Hard Tissue Biol 2010; 19(3): 187–191, https://doi.org/10.2485/jhtb.19.187.
- Ochiai H., Okada S., Saito A., Hoshi K., Yamashita H., Takato T., Azuma T. Inhibition of insulin-like growth factor-1 (IGF-1) expression by prolonged transforming growth factor-β1 (TGF-β1) administration suppresses osteoblast differentiation. J Biol Chem 2012; 287(27): 22654–22661, https://doi.org/10.1074/jbc.m111.279091.
- Lau K.H., Kapur S., Kesavan C., Baylink D.J. Up-regulation of the Wnt, estrogen receptor, insulin-like growth factor-I, and bone morphogenetic protein pathways in C57BL/6J osteoblasts as opposed to C3H/HeJ osteoblasts in part contributes to the differential anabolic response to fluid shear. J Biol Chem 2006; 281(14): 9576–9588, https://doi.org/10.1074/jbc.M509205200.
- Lean J.M., Mackay A.G., Chow J.W., Chambers T.J. Osteocytic expression of mRNA for c-fos and IGF-I: an immediate early gene response to an osteogenic stimulus. Am J Physiol 1996; 270(6 Pt1): 937–945, https://doi.org/10.1152/ajpendo.1996.270.6.e937.
- Kawata A., Mikuni-Takagaki Y. Mechanotransduction in stretched osteocytes — temporal expression of immediate early and other genes. Biochem Biophys Res Commun 1998; 246(2): 404–408, https://doi.org/10.1006/bbrc.1998.8632.
- Reijnders C.M., Bravenboer N., Tromp A.M., Blankenstein M.A., Lips P. Effect of mechanical loading on insulin-like growth factor-I gene expression in rat tibia. J Endocrinol 2007; 192(1): 131–140, https://doi.org/10.1677/joe.1.06880.
- Sakata T., Wang Y., Halloran B.P., Elalieh H.Z., Cao J., Bikle D.D. Skeletal unloading induces resistance to insulin-like growth factor-I (IGF-I) by inhibiting activation of the IGF-I signaling pathways. J Bone Miner Res 2004; 19(3): 436–446, https://doi.org/10.1359/jbmr.0301241.
- Kesavan C., Wergedal J.E., Lau K.H., Mohan S. Conditional disruption of IGF-I gene in type 1alpha collagen-expressing cells shows an essential role of IGF-I in skeletal anabolic response to loading. Am J Physiol Endocrinol Metab 2011; 301(6): E1191–E1197, https://doi.org/10.1152/ajpendo.00440.2011.
- Sheng M.H., Lau K.H., Baylink D.J. Role of osteocyte-derived insulin-like growth factor i in developmental growth, modeling, remodeling, and regeneration of the bone. J Bone Metab 2014; 21(1): 41–54, https://doi.org/10.11005/jbm.2014.21.1.41.
- Geiger F., Lorenz H., Xu W., Szalay K., Kasten P., Claes L., Augat P., Richter W. VEGF producing bone marrow stromal cells (BMSC) enhance vascularization and resorption of a natural coral bone substitute. Bone 2007; 41(4): 516–522, https://doi.org/10.1016/j.bone.2007.06.018.
- Wernike E., Montjovent M.O., Liu Y., Wismeijer D., Hunziker E.B., Siebenrock K.A., Hofstetter W., Klenke F. VEGF incorporated into calcium phosphate ceramics promotes vascularisation and bone formation in vivo. Eur Cell Mater 2010; 19: 30–40, https://doi.org/10.22203/ecm.v019a04.
- Maes C., Coenegrachts L., Stockmans I., Daci E., Luttun A., Petryk A., Gopalakrishnan R., Moermans K., Smets N., Verfaillie C., Carmeliet P., Bouillon R., Carmeliet G. Placental growth factor mediates mesenchymal cell development, cartilage turnover, and bone remodeling during fracture repair. J Clin Invest 2006; 116(5): 1230–1242, https://doi.org/10.1172/jci26772.
- McCoy R.J., Widaa A., Watters K.M., Wuerstle M., Stallings R.L., Duffy G.P., O’Brien F.J. Orchestrating osteogenic differentiation of mesenchymal stem cells — identification of placental growth factor as a mechanosensitive gene with a pro-osteogenic role. Stem Cells 2013; 31(11): 2420–2431, https://doi.org/10.1002/stem.1482.
- Liu Y., Deng L.Z., Sun H.P., Xu J.Y., Li Y.M., Xie X., Zhang L.M., Deng F.L. Sustained dual release of placental growth factor-2 and bone morphogenic protein-2 from heparin-based nanocomplexes for direct osteogenesis. Int J Nanomedicine 2016; 11: 1147–1158, https://doi.org/10.2147/ijn.s100156.
- Kadam A., Millhouse P.W., Kepler C.K., Radcliff K.E., Fehlings M.G., Janssen M.E., Sasso R.C., Benedict J.J., Vaccaro A.R. Bone substitutes and expanders in spine surgery: a review of their fusion efficacies. Int J Spine Surg 2016; 10: 33, https://doi.org/10.14444/3033.
- Ghassemi T., Shahroodi A., Ebrahimzadeh M.H., Mousavian A., Movaffagh J., Moradi A. Current concepts in scaffolding for bone tissue engineering. Arch Bone Jt Surg 2018; 6(2): 90–99.
- Chen W., Liu X., Chen Q., Bao C., Zhao L., Zhu Z., Xu H.H.K. Angiogenic and osteogenic regeneration in rats via calcium phosphate scaffold and endothelial cell co-culture with human bone marrow mesenchymal stem cells (MSCs), human umbilical cord MSCs, human induced pluripotent stem cell-derived MSCs and human embryonic stem cell-derived MSCs. J Tissue Eng Regen Med 2018; 12(1): 191–203, https://doi.org/10.1002/term.2395.