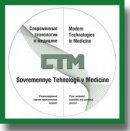
Перспективы использования бактериоцинов нормальной микробиоты в антибактериальной терапии (обзор)
Прогрессирующая резистентность микроорганизмов к антибиотикам стимулирует поиск альтернативной антимикробной терапии. В обзоре изучается возможность использования бактериоцинов в качестве антибактериальных препаратов. Дается определение бактериоцинов и объясняется их отличие от «классических» антибиотиков. Представлена современная классификация бактериоцинов, их свойства и механизмы действия. Приводятся примеры бактерий — представителей нормальной микробиоты человека — основных продуцентов бактериоцинов. Исследуется роль бактериоцинов микробиоты в обеспечении резистентности слизистых оболочек и стабилизации микробиома человека, а также возможность их применения при создании пробиотических препаратов. Описываются преимущества и недостатки бактериоцинов в качестве альтернативных антибактериальных препаратов. Рассматриваются варианты применения бактериоцинов в антимикробной терапии, а также способы их промышленного получения.
- World Health Organization. Antimicrobial resistance: global report on surveillance. Geneva; 2014. URL: http://www.who.int/drugresistance/documents/surveillancereport/en/.
- Holmes A.H., Moore L.S., Sundsfjord A., Steinbakk M., Regmi S., Karkey A., Guerin P.J., Piddock L.J. Understanding the mechanisms and drivers of antimicrobial resistance. Lancet 2016; 387(10014): 176–187, https://doi.org/10.1016/s0140-6736(15)00473-0.
- Blaser M. Antibiotic overuse: stop the killing of beneficial bacteria. Nature 2011; 476(7361): 393–394, https://doi.org/10.1038/476393a.
- Willing B.P., Russell S.L., Finlay B.B. Shifting the balance: antibiotic effects on host-microbiota mutualism. Nat Rev Microbiol 2011; 9(4): 233–243, https://doi.org/10.1038/nrmicro2536.
- Michael C.A., Dominey-Howes D., Labbate M. The antimicrobial resistance crisis: causes, consequences, and management. Front Public Health 2014; 2: 145, https://doi.org/10.3389/fpubh.2014.00145.
- Валышев А.В., Валышева Н.А. Комбинация антибиотиков и бактериоцинов — эффективный способ борьбы с резистентными микроорганизмами. Бюллетень Оренбургского научного центра УроРАН (электронный журнал) 2016; 4: 1–6.
- Cotter P.D., Ross R.P., Hill C. Bacteriocins — a viable alternative to antibiotics? Nat Rev Microbiol 2013; 11(2): 95–105, https://doi.org/10.1038/nrmicro2937.
- Ермоленко Е.И. Бактериоцины энтерококков: проблемы и перспективы использования (обзор литературы). Вестник Санкт-Петербургского университета. Медицина 2009; 3: 78–93.
- Ермоленко Е.И., Черныш А.Ю., Берлов М.Н., Тотолян А.А., Суворов А.Н. Антагонистическая активность энтерококков в отношении Streptococcus pyogenes. Вестник Санкт-Петербургского университета. Медицина 2008; 3: 137–144.
- Stoyanova L.G., Ustyugova E.A., Netrusov A.I. Antibacterial metabolites of lactic acid bacteria: their diversity and properties. Appl Biochem Microbiol 2012; 48(3): 229–243, https://doi.org/10.1134/s0003683812030143.
- Сультимова Т.Д., Захаров Е.В. Бактериоцины молочнокислых бактерий. Вестник ВСГУТУ 2016; 2(59): 41–47.
- Breukink E., de Kruijff B. Lipid II as a target for antibiotics. Nat Rev Drug Discov 2006; 5(4): 321–332, https://doi.org/10.1038/nrd2004.
- Enterococci: from commensals to leading causes of drug resistant infection. Gilmore M.S., Clewell D.B., Ike Y., Shankar N. (editors). Boston: Massachusetts Eye and Ear Infirmary; 2014.
- Блинкова Л.П., Альтшулер М.Л., Дорофеева Е.С., Горобец О.Б. Молекулярные основы продукции и действия бактериоцинов. Журнал микробиологии, эпидемиологии и иммунологии 2007; 2: 97–104.
- Mokoena M.P. Lactic acid bacteria and their bacteriocins: classification, biosynthesis and applications against uropathogens: a mini-review. Molecules 2017; 22(8): 1255, https://doi.org/10.3390/molecules22081255.
- Engevik M.A. Versalovic J. Biochemical features of beneficial microbes: foundations for therapeutic microbiology. Microbiol Spectr 2017; 5(5): 3–47, https://doi.org/10.1128/microbiolspec.BAD-0012-2016.
- Nishie M., Nagao J., Sonomoto K. Antibacterial peptides “bacteriocins”: an overview of their diverse characteristics and applications. Biocontrol Sci 2012; 17(1): 1–16, https://doi.org/10.4265/bio.17.1.
- Guder A., Wiedemann I., Sahl H.G. Posttranslationally modified bacteriocins — the lantibiotics. Biopolymers 2000; 55(1): 62–73, https://doi.org/10.1002/1097-0282(2000)55:162::aid-bip603.3.co;2-p.
- Field D., Begley M., O’Connor P.M., Daly K.M., Hugenholtz F., Cotter P.D., Hill C., Ross R.P. Bioengineered nisin A derivatives with enhanced activity against both gram positive and gram negative pathogens. PLoS One 2012; 7(10): e46884, https://doi.org/10.1371/journal.pone.0046884.
- Field D., Daly K., O’Connor P.M., Cotter P.D., Hill C., Ross R.P. Efficacies of nisin A and nisin V semipurified preparations alone and in combination with plant essential oils for controlling Listeria monocytogenes. Appl Environ Microbiol 2015; 81(8): 2762–2769, https://doi.org/10.1128/aem.00070-15.
- Field D., O’ Connor R., Cotter P.D., Ross R.P., Hill C. In vitro activities of nisin and nisin derivatives alone and in combination with antibiotics against staphylococcus biofilms. Front Microbiol 2016; 7: 508, https://doi.org/10.3389/fmicb.2016.00508.
- Field D., Seisling N., Cotter P.D., Ross R.P., Hill C. Synergistic nisin-polymyxin combinations for the control of Pseudomonas biofilm formation. Front Microbiol 2016; 7: 1713, https://doi.org/10.3389/fmicb.2016.01713.
- Jabés D., Brunati C., Candiani G., Riva S., Romanó G., Donadio S. Efficacy of the new lantibiotic NAI-107 in experimental infections induced by MDR gram positive pathogens. Antimicrob Agents Chemother 2011; 55(4): 1671–1676, https://doi.org/10.1128/aac.01288-10.
- McAuliffe O., Ross R.P., Hill C. Lantibiotics: structure, biosynthesis and mode of action. FEMS Microbiol Rev 2001; 25(3): 285–308, https://doi.org/10.1016/s0168-6445(00)00065-6.
- Balla E., Dicks L.M., Du Toit M., Van Der Merwe M.J., Holzapfel W.H. Characterization and cloning of the genes encoding enterocin 1071A and enterocin 1071B, two antimicrobial peptides produced by Enterococcus faecalis BFE 1071. Appl Environ Microbiol 2000; 66(4): 1298–1304, https://doi.org/10.1128/aem.66.4.1298-1304.2000.
- González C., Langdon G.M., Bruix M., Gálvez A., Valdivia E., Maqueda M., Rico M. Bacteriocin AS-48, a microbial cyclic polypeptide structurally and functionally related to mammalian NK-lysin. Proc Natl Acad Sci U S A 2000; 97(21): 11221–11226, https://doi.org/10.1073/pnas.210301097.
- Bastos M. do C. de F., Coutinho B.G., Coelho M.L.V. Lysostaphin: a Staphylococcal bacteriolysin with potential clinical applications. Pharmaceuticals 2010; 3(4): 1139–1161, https://doi.org/10.3390/ph3041139.
- Pickard J.M., Zeng M.Y., Caruso R., Núñez G. Gut microbiota: role in pathogen colonization, immune responses, and inflammatory disease. Immunol Rev 2017; 279(1): 70–89, https://doi.org/10.1111/imr.12567.
- Chiu L., Bazin T., Truchetet M., Schaeverbeke T., Delhaes L., Pradeu T. Protective microbiota: from localized to long-reaching co-immunity. Front Immunol 2017; 8: 1678, https://doi.org/10.3389/fimmu.2017.01678.
- Gillor O., Giladi I., Riley M.A. Persistence of colicinogenic Escherichia coli in the mouse gastrointestinal tract. BMC Microbiol 2009; 9(1): 165, https://doi.org/10.1186/1471-2180-9-165.
- Zheng J., Gänzle M.G., Lin X.B., Ruan L., Sun M. Diversity and dynamics of bacteriocins from human microbiome. Environ Microbiol 2015; 17(6): 2133–2143, https://doi.org/10.1111/1462-2920.12662.
- Mignolet J., Fontaine L., Sass A., Nannan C., Mahillon J., Coenye T., Hols P. Circuitry rewiring directly couples competence to predation in the gut dweller Streptococcus salivarius. Cell Rep 2018; 22(7): 1627–1638, https://doi.org/10.1016/j.celrep.2018.01.055.
- van der Ploeg J.R. Regulation of bacteriocin production in Streptococcus mutans by the quorum-sensing system required for development of genetic competence. J Bacteriol 2005; 187(12): 3980–3989, https://doi.org/10.1128/jb.187.12.3980-3989.2005.
- Gillor O., Ghazaryan L. Recent advances in bacteriocin application as antimicrobials. Recent Pat Antiinfect Drug Discov 2007; 2(2): 115–122, https://doi.org/10.2174/157489107780832613.
- Majeed H., Lampert A., Ghazaryan L., Gillor O. The weak shall inherit: bacteriocin-mediated interactions in bacterial populations. PLoS One 2013; 21: 8(5): e63837, https://doi.org/10.1371/journal.pone.0063837.
- Kommineni S., Bretl D.J., Lam V., Chakraborty R., Hayward M., Simpson P., Cao Y., Bousounis P., Kristich C.J., Salzman N.H. Bacteriocin production augments niche competition by enterococci in the mammalian gastrointestinal tract. Nature 2015; 29: 526(7575): 719–722, https://doi.org/10.1038/nature15524.
- Hecht A.L., Casterline B.W., Earley Z.M., Goo Y.A., Goodlett D.R., Bubeck Wardenburg J. Strain competition restricts colonization of an enteric pathogen and prevents colitis. EMBO Rep 2016; 17(9): 1281–1291, https://doi.org/10.15252/embr.201642282.
- Janek D., Zipperer A., Kulik A., Krismer B., Peschel A. High frequency and diversity of antimicrobial activities produced by nasal Staphylococcus strains against bacterial competitors. PLoS Pathog 2016; 4: 12(8): e1005812, https://doi.org/10.1371/journal.ppat.1005812.
- Бондаренко В.М., Суворов А.Н. Симбиотические энтерококки и проблема энтерококковой оппортунистической инфекции. М; 2007.
- Saleh F.A., El-Sayed E.M. Isolation and characterization of bacteriocins produced by Bifidobacterium lactis BB-12 and Bifidobacterium longum BB-46. In: 9th Egyptian conference for dairy science and technology. Cairo: Research Papers; 2004; p. 323–337.
- Lee J.H., Li X., O’Sullivan D.J. Transcription analysis of a lantibiotic gene cluster from Bifidobacterium longum DJO10A. Appl Environ Microbiol 2011; 77(17): 5879–5887, https://doi.org/10.1128/aem.00571-11.
- Djadouni F., Kihal M. Antimicrobial activity of lactic acid bacteria and the spectrum of their biopeptides against spoiling germs in foods. Braz Arch Biol Technol 2012; 55(3): 435–443, https://doi.org/10.1590/s1516-89132012000300015.
- Martinez F.C., Balciunas E.M., Converti A., Cotter P.D., de Souza Oliveira R.P. Bacteriocin production by Bifidobacterium spp. A review. Biotechnol Adv 2013; 31(4): 482–488, https://doi.org/10.1016/j.biotechadv.2013.01.010.
- Kim Y.C., Tarr A.W., Penfold C.N. Colicin import into E. coli cells: a model system for insights into the import mechanisms of bacteriocins. Biochim Biophys Acta 2014; 1843(8): 1717–31, https://doi.org/10.1016/j.bbamcr.2014.04.010.
- Соловьева И.В., Белова И.В., Точилина А.Г., Григорьева Г.И., Леонов А.В., Воробьев Г.Е., Иванова Т.П., Жирнов В.А. Профилактика формирования устойчивых дисбиотических состояний микрофлоры желудочно-кишечного тракта студентов с помощью нового симбиотика. Медицинский альманах 2011; 2(15): 112–114.
- Дисбиоз кишечника. Руководство по диагностике и лечению. Под ред. Суворова А.Н., Ткаченко Е.И., Успенского Ю.П. СПб.: ИнформМед; 2013; 270 с.
- Suvorov A., Simanenkov V., Gromova L., Kolodjieva V., Tsapieva A., Chernish A., Solovieva O., Ermolenko E. Enterococci as probiotics or autoprobiotics. In: Ivanova I. (editor). Prebiotics and probiotics potential for human health. Paisi Hilendarski, Sofia; 2011; p. 104–112.
- Гончар Н.В., Алехина Л.А., Суворов А.Н. Пробиотические штаммы энтерококков как средства терапии и профилактики заболеваний кишечника у детей (обзор литературы). Экспериментальная и клиническая гастроэнтерология 2013; 1: 74–78.
- Щепитова Н.Е., Сычева М. В., Карташова О.Л. Скрининг штаммов энтерококков с целью разработки на их основе препаратов-пробиотиков. Вестник Оренбургского государственного университета 2015; 13(188): 226–233.
- Соловьева И.В., Точилина А.Г., Белова И.В., Новикова Н.А., Иванова Т.П. Биологические свойства лактобацилл. Перспективы использования в лабораториях роспотребнадзора экспресс-методов амплификации нуклеиновых кислот (МАНК) при контроле качества пищевых продуктов, БАД к пище, лекарственных форм, содержащих лактобациллы. Медиаль 2014; 2(12): 29–44.
- Шендеров Б.А., Синица А.В., Захарченко М.М. Метабиотики: вчера, сегодня, завтра. СПб: ИнформМед; 2017.
- Dobson A., Cotter P.D., Ross R.P., Hill C. Bacteriocin production: a probiotic trait? Appl Environ Microbiol 2012; 78(1): 1–6, https://doi.org/10.1128/aem.05576-11.
- Umu Ö.C.O., Rudi K., Diep D.B. Modulation of the gut microbiota by prebiotic fibres and bacteriocins. Microb Ecol Health Dis 2017; 28(1): 1348886, https://doi.org/10.1080/16512235.2017.1348886.
- Aranha C.C., Gupta S.M., Reddy K.V. Assessment of cervicovaginal cytokine levels following exposure to microbicide nisin gel in rabbits. Cytokine 2008; 43(1): 63–70, https://doi.org/10.1016/j.cyto.2008.04.005.
- Mathur H., O’Connor P.M., Hill C., Cotter P.D., Ross R.P. Analysis of anti-Clostridium difficile activity of thuricin CD, vancomycin, metronidazole, ramoplanin, and actagardine, both singly and in paired combinations. Antimicrob Agents Chemother 2013; 57(6): 2882–2886, https://doi.org/10.1128/aac.00261-13.
- Ming L., Zhang Q., Yang L., Huang J.A. Comparison of antibacterial effects between antimicrobial peptide and bacteriocins isolated from Lactobacillus plantarum on three common pathogenic bacteria. Int J Clin Exp Med 2015; 8(4): 5806–5811.
- Mathur H., Des F., Rea M.C., Cotter P.D., Hill C., Ross R.P. Bacteriocin-antimicrobial synergy: a medical and food perspective. Front Microbiol 2017; 8: 1205, https://doi.org/10.3389/fmicb.2017.01205.
- Yuan J., Zhang Z.Z., Chen X.Z., Yang W., Huan L.D. Site-directed mutagenesis of the hinge region of nisin Z and properties of nisin Z mutants. Appl Microbiol Biotechnol 2004; 64(6): 806–815, https://doi.org/10.1007/s00253-004-1599-1.
- Draper L.A., Cotter P.D., Hill C., Ross R.P. Lantibiotic resistance. Microbiol Mol Biol Rev 2015; 79(2): 171–191, https://doi.org/10.1128/mmbr.00051-14.
- Modi K.D., Chikindas M.L., Montville T.J. Sensitivity of nisin-resistant Listeria monocytogenes to heat and the synergistic action of heat and nisin. Lett Appl Microbiol 2000; 30(3): 249–253, https://doi.org/10.1046/j.1472-765x.2000.00708.x.
- McBride S.M., Sonenshein A.L. Identification of a genetic locus responsible for antimicrobial peptide resistance in Clostridium difficile. Infect Immun 2011; 79(1): 167–176, https://doi.org/10.1128/iai.00731-10.
- Suarez J.M., Edwards A.N., McBride S.M. The Clostridium difficile cpr locus is regulated by a noncontiguous two-component system in response to type A and B lantibiotics. J Bacteriol 2013; 195(11): 2621–2631, https://doi.org/10.1128/jb.00166-13
- Khosa S., Alkhatib Z., Smits S.H. NSR from Streptococcus agalactiae confers resistance against nisin and is encoded by a conserved nsr operon. Biol Chem 2013; 394(11): 1543–1549, https://doi.org/10.1515/hsz-2013-0167.
- Abi Khattar Z., Rejasse A., Destoumieux-Garzón D., Escoubas J.M., Sanchis V., Lereclus D., Givaudan A., Kallassy M., Nielsen-Leroux C., Gaudriault S. The dlt operon of Bacillus cereus is required for resistance to cationic antimicrobial peptides and for virulence in insects. J Bacteriol 2009; 191(22): 7063–7073, https://doi.org/10.1128/jb.00892-09.
- Kovács M., Halfmann A., Fedtke I., Heintz M., Peschel A., Vollmer W., Hakenbeck R., Brückner R. A functional dlt operon, encoding proteins required for incorporation of D-alanine in teichoic acids in gram-positive bacteria, confers resistance to cationic antimicrobial peptides in Streptococcus pneumoniae. J Bacteriol 2006; 188(16): 5797–5805, https://doi.org/10.1128/jb.00336-06.
- Abachin E., Poyart C., Pellegrini E., Milohanic E., Fiedler F., Berche P., Trieu-Cuot P. Formation of D-alanyl-lipoteichoic acid is required for adhesion and virulence of Listeria monocytogenes. Mol Microbiol 2002; 43: 1–14, https://doi.org/10.1046/j.1365-2958.2002.02723.x.
- Kjos M., Nes I.F., Diep D.B. Mechanisms of resistance to bacteriocins targeting the mannose phosphotransferase system. Appl Environ Microbiol 2011; 77(10): 3335–3342, https://doi.org/10.1128/aem.02602-10.
- Kommineni S., Kristich C.J., Salzman N.H. Harnessing bacteriocin biology as targeted therapy in the GI tract. Gut Microbes 2016; 7(6): 512–517, https://doi.org/10.1080/19490976.2016.1233089.
- Yang S.C., Lin C.H., Sung C.T., Fang J.Y. Antibacterial activities of bacteriocins: application in foods and pharmaceuticals. Front Microbiol 2014; 5: 241, https://doi.org/10.3389/fmicb.2014.00241.
- Mendes C.A., Cordeiro J.A., Burdmann E.A. Prevalence and risk factors for acute kidney injury associated with parenteral polymyxin B use. Ann Pharmacother 2009; 43(12): 1948–1955, https://doi.org/10.1345/aph.1m277.
- Abdelraouf K., Braggs K.H., Yin T., Truong L.D., Hu M., Tam V.H. Characterization of polymyxin B-induced nephrotoxicity: implications for dosing regimen design. Antimicrob Agents Chemother 2012; 56(9): 4625–4629, https://doi.org/10.1128/aac.00280-12.
- Ghobrial O., Derendorf H., Hillman J.D. Human serum binding and its effect on the pharmacodynamics of the lantibiotic MU1140. Eur J Pharm Sci 2010; 41(5): 658–664, https://doi.org/10.1016/j.ejps.2010.09.005.
- Ghobrial O.G., Derendorf H., Hillman J.D. Development and validation of a LC–MS quantification method for the lantibiotic MU1140 in rat plasma. J Pharm Biomed Anal 2009; 49(4): 970–975, https://doi.org/10.1016/j.jpba.2008.12.022.
- Ghobrial O., Derendorf H., Hillman J.D. Pharmacokinetic and pharmacodynamic evaluation of the lantibiotic MU1140. J Pharm Sci 2010; 99(5): 2521–2528, https://doi.org/10.1002/jps.22015.
- van Heel A.J., Montalban-Lopez M., Kuipers O.P. Evaluating the feasibility of lantibiotics as an alternative therapy against bacterial infections in humans. Expert Opin Drug Metab Toxicol 2011; 7(6): 675–680, https://doi.org/10.1517/17425255.2011.573478.
- Caballero Gómez N., Abriouel H., Grande M.J., Pérez Pulido R., Gálvez A. Combined treatments of enterocin AS-48 with biocides to improve the inactivation of methicillin-sensitive and methicillin-resistant Staphylococcus aureus planktonic and sessile cells. Int J Food Microbiol 2013; 163(2–3): 96–100, https://doi.org/10.1016/j.ijfoodmicro.2013.02.018.
- Sharma A., Srivastava S. Anti-Candida activity of two-peptide bacteriocins, plantaricins (Pln E/F and J/K) and their mode of action. Fungal Biol 2014; 118(2): 264–275, https://doi.org/10.1016/j.funbio.2013.12.006.
- Cavera V.L., Volski A., Chikindas M.L. The natural antimicrobial subtilosin A synergizes with lauramide arginine ethyl ester (LAE), ε-poly-l-lysine (polylysine), clindamycin phosphate and metronidazole, against the vaginal pathogen Gardnerella vaginalis. Probiotics Antimicrob Proteins 2015; 7(2): 164–171, https://doi.org/10.1007/s12602-014-9183-1.
- Brumfitt W., Salton M.R., Hamilton-Miller J.M. Nisin, alone and combined with peptidoglycan-modulating antibiotics: activity against methicillin-resistant Staphylococcus aureus and vancomycin-resistant enterococci. J Antimicrob Chemother 2002; 50(5): 731–734, https://doi.org/10.1093/jac/dkf190.
- Draper L.A., Cotter P.D., Hill C., Ross R.P. The two peptide lantibioticlacticin 3147 acts synergistically with polymyxin to inhibit gram negative bacteria. BMC Microbiol 2013; 13(1): 212, https://doi.org/10.1186/1471-2180-13-212.
- LeBel G., Piché F., Frenette M., Gottschalk M., Grenier D. Antimicrobial activity of nisin against the swine pathogen Streptococcus suis and its synergistic interaction with antibiotics. Peptides 2013; 50: 19–23, https://doi.org/10.1016/j.peptides.2013.09.014.
- Tong Z., Zhang L., Ling J., Jian Y., Huang L., Deng D. An in vitro study on the effect of free amino acids alone or in combination with nisin on biofilms as well as on planktonic bacteria of Streptococcus mutans. PLoS One 2014; 9(6): e99513, https://doi.org/10.1371/journal.pone.0099513.
- Tong Z., Zhang Y., Ling J., Ma J., Huang L., Zhang L. In vitro study on the effects of nisin on the antibacterial activities of 18 antibiotics against Enterococcus faecalis. PLoS One 2014; 9(2): e89209, https://doi.org/10.1371/journal.pone.0089209.
- Vaillancourt K., LeBel G., Frenette M., Gottschalk M., Grenier D. Suicin 3908, a new lantibiotic produced by a strain of Streptococcus suis serotype 2 isolated from a healthy carrier pig. PLoS One 2015; 10(2): e0117245, https://doi.org/10.1371/journal.pone.0117245.
- Flemming H.C., Wingender J. The biofilm matrix. Nat Rev Microbiol 2010; 8(9): 623–633, https://doi.org/10.1038/nrmicro2415.
- Mah T.F., O’Toole G.A. Mechanisms of biofilm resistance to antimicrobial agents. Trends Microbiol 2001; 9(1): 34–39, https://doi.org/10.1016/s0966-842x(00)01913-2.
- Davies D. Understanding biofilm resistance to antibacterial agents. Nat Rev Drug Discov 2003; 2(2): 114–122, https://doi.org/10.1038/nrd1008.
- Mataraci E., Dosler S. In vitro activities of antibiotics and antimicrobial cationic peptides alone and in combination against methicillin-resistant Staphylococcus aureus biofilms. Antimicrob Agents Chemother 2012; 56(12): 6366–6371, https://doi.org/10.1128/aac.01180-12.
- Dosler S., Mataraci E. In vitro pharmacokinetics of antimicrobial cationic peptides alone and in combination with antibiotics against methicillin resistant Staphylococcus aureus biofilms. Peptides 2013; 49: 53–58, https://doi.org/10.1016/j.peptides.2013.08.008.
- Algburi A., Volski A., Chikindas M.L. Natural antimicrobials subtilosin and lauramide arginine ethyl ester synergize with conventional antibiotics clindamycin and metronidazole against biofilms of Gardnerella vaginalis but not against biofilms of healthy vaginal lactobacilli. Pathog Dis 2015; 73: ftv018, https://doi.org/10.1093/femspd/ftv018.
- Ceotto-Vigoder H., Marques S.L., Santos I.N., Alves M.D., Barrias E.S., Potter A., Alviano D.S., Bastos M.C. Nisin and lysostaphin activity against preformed biofilm of Staphylococcus aureus involved in bovine mastitis. J Appl Microbiol 2016; 121(1): 101–114, https://doi.org/10.1111/jam.13136.
- Rodríguez-Rubio L., García P., Rodríguez A., Billington C., Hudson J.A., Martínez B. Listeria phages and coagulin C23 act synergistically to kill Listeria monocytogenes in milk under refrigeration conditions. Int J Food Microbiol 2015; 205: 68–72, https://doi.org/10.1016/j.ijfoodmicro.2015.04.007.
- Martínez B., Obeso J.M., Rodríguez A., García P. Nisin-bacteriophage cross resistance in Staphylococcus aureus. Int J Food Microbiol 2008; 122(3): 253–258, https://doi.org/10.1016/j.ijfoodmicro.2008.01.011.
- Nascimento J.G., Guerreiro-Pereira M.D., Costa S F., São-José C., Santos M.A. Nisin-triggered activity of Lys44, the secreted endolysin from Oenococcus oeni phage fOg44. J Bacteriol 2008; 190(1): 457–461, https://doi.org/10.1128/jb.01195-07.
- Obeso J.M., Martínez B., Rodríguez A., García P. Lytic activity of the recombinant staphylococcal bacteriophage phiH5 endolysin active against Staphylococcus aureus in milk. Int J Food Microbiol 2008; 128(2): 212–218, https://doi.org/10.1016/j.ijfoodmicro.2008.08.010.
- García P., Martínez B., Rodríguez L., Rodríguez A. Synergy between the phage endolysin LysH5 and nisin to kill Staphylococcus aureus in pasteurized milk. Int J Food Microbiol 2010; 141: 151–155, https://doi.org/10.1016/j.ijfoodmicro.2010.04.029.
- Dajcs J.J., Thibodeaux B.A., Girgis D.O., Shaffer M.D., Delvisco S.M., O’Callaghan R.J. Immunity to lysostaphin and its therapeutic value for ocular MRSA infections in the rabbit. Invest Ophthalmol Vis Sci 2002; 43(12): 3712–3716.
- Becker S.C., Foster-Frey J., Donovan D.M. The phage K lytic enzyme LysK and lysostaphin act synergistically to kill MRSA. FEMS Microbiol Lett 2008; 287(2): 185–191, https://doi.org/10.1111/j.1574-6968.2008.01308.x.
- Mathur H., Rea M.C., Cotter P.D., Hill C., Ross R.P. The sactibiotic subclass of bacteriocins: an update. Curr Protein Pept Sci 2015; 16(6) 549–558, https://doi.org/10.2174/1389203716666150515124831.
- Блинкова Л.П., Машенцева Н.Г., Хорольский В.В., Горобец О.Б., Дорофеева Е.С. Биотехнологические условия синтеза бактериоцинов. Журнал микробиологии, эпидемиологии и иммунологии 2006; 2: 83–89.
- Pei J., Yuan Y., Yue T. Characterization of bacteriocin bificin C6165: a novel bacteriocin. J Appl Microbiol 2013; 114(5): 1273–1284, https://doi.org/10.1111/jam.12145.
- Bédard F., Biron E. Recent progress in the chemical synthesis of class II and S-glycosylated bacteriocins. Front Microbiol 2018; 9: 1048, https://doi.org/10.3389/fmicb.2018.01048.