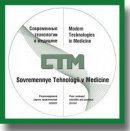
Особенности неспецифической резистентности при ожоговой травме: патофизиологическая оценка (обзор)
Проведен анализ современных сведений о состоянии неспецифической резистентности у ожоговых пациентов. Рассматриваются проблемы оценки функций субпопуляций нейтрофилов и моноцитов/макрофагов при тяжелой ожоговой травме. Установлена роль клеток крови в последующем развитии иммунной дисфункции, восприимчивости к сепсису и синдрома полиорганной недостаточности. Показано значение вторичных осложнений в развитии заболеваемости и смертности среди госпитализированных пациентов с ожоговой травмой. Рассмотрены новые подходы к выявлению лиц с риском неблагоприятного исхода.
- Ruiz-Castilla M., Roca O., Masclans J.R., Barret J.P. Recent advances in biomarkers in severe burns. Shock 2016; 45(2): 117–125, https://doi.org/10.1097/SHK.0000000000000497.
- Карякин Н.Н., Клеменова И.А. Технологии лечения ожогов в условиях влажной среды. Международный журнал прикладных и фундаментальных исследований 2015; 9(3): 495–499.
- Abdullahi A., Chen P., Stanojcic M., Sadri A.R., Coburn N., Jeschke M.G. IL-6 signal from the bone marrow is required for the browning of white adipose tissue post burn injury. Shock 2017; 47(1): 33–39, https://doi.org/10.1097/shk.0000000000000749.
- Auger C., Samadi O., Jeschke M.G. The biochemical alterations underlying post-burn hypermetabolism. Biochim Biophys Acta Mol Basis Dis 2017; 1863(10 Pt B): 2633–2644, https://doi.org/10.1016/j.bbadis.2017.02.019.
- Wang T., Nie C., Zhang H., Zeng X.Q., Yu H.T., Shi S.P., Wei Z.R., Shi X.Q. Epidemiological characteristics and disease burden of burns in children in Northern Guizhou, China. Chin Med J (Engl) 2018; 131(17): 2125–2127, https://doi.org/10.4103/0366-6999.239312.
- Моррисон В.В., Божедомов А.Ю., Симонян М.А., Моррисон А.В. Системный воспалительный ответ и цитокиновый профиль в динамике развития ожоговой болезни. Саратовский научно-медицинский журнал 2017; 13(2): 229–232.
- Muñoz B., Suárez-Sánchez R., Hernández-Hernández O., Franco-Cendejas R., Cortés H., Magaña J.J. From traditional biochemical signals to molecular markers for detection of sepsis after burn injuries. Burns 2019; 45(1): 16–31, https://doi.org/10.1016/j.burns.2018.04.016.
- Жилинский Е.В. Прогнозирование сепсиса при ожоговой болезни. Политравма 2017; 2: 50–56.
- Greenhalgh D.G. Sepsis in the burn patient: a different problem than sepsis in the general population. Burns Trauma 2017; 5: 23, https://doi.org/10.1186/s41038-017-0089-5.
- Nunez Lopez O., Cambiaso-Daniel J., Branski L.K., Norbury W.B., Herndon D.N. Predicting and managing sepsis in burn patients: current perspectives. Ther Clin Risk Manag 2017; 13: 1107–1117, https://doi.org/10.2147/TCRM.S119938.
- Fuss J., Voloboyeva A., Poliovyj V. Prognostic value of using neutrophil-lymphocyte ratio in patients with burn injury for the diagnosis of sepsis and bacteraemia. Pol Przegl Chir 2018; 90(5): 13–16, https://doi.org/10.5604/01.3001.0012.0971.
- Faix J.D. Biomarkers of sepsis. Crit Rev Clin Lab Sci 2013; 50(1): 23–36, https://doi.org/10.3109/10408363.2013.764490.
- Freystätter C., Radtke C., Ihra G., Thalhammer F., Fochtmann-Frana A. Sepsis caused by multidrug-resistant klebsiella pneumoniae infection in a 23-year-old burn patient: case report and literature review. Ann Burns Fire Disasters 2018; 31(2): 113–117.
- Mayadas T.N., Cullere X., Lowell C.A. The multifaceted functions of neutrophils. Annu Rev Pathol 2014; 9: 181–218, https://doi.org/10.1146/annurev-pathol-020712-164023.
- Teng T.S., Ji A.L., Ji X.Y., Li Y.Z. Neutrophils and immunity: from bactericidal action to being conquered. J Immunol Res 2017: 9671604, https://doi.org/10.1155/2017/9671604.
- Voisin M.B., Nourshargh S. Neutrophil transmigration: emergence of an adhesive cascade within venular walls. J Innate Immun 2013; 5(4): 336–347, https://doi.org/10.1159/000346659.
- Fiedler K., Brunner C. The role of transcription factors in the guidance of granulopoiesis. Am J Blood Res 2012; 2(1): 57–65.
- Tecchio C., Cassatella M.A. Neutrophil-derived chemokines on the road to immunity. Semin Immunol 2016; 28(2): 119–128, https://doi.org/10.1016/j.smim.2016.04.003.
- Greenlee-Wacker M.C. Clearance of apoptotic neutrophils and resolution of inflammation. Immunol Rev 2016; 273(1): 357–370, https://doi.org/10.1111/imr.12453.
- Chen F., Wu W., Millman A., Craft J.F., Chen E., Patel N., Boucher J.L., Urban J.F. Jr., Kim C.C., Gause W.C. Neutrophils prime a long-lived effector macrophage phenotype that mediates accelerated helminth expulsion. Nat Immunol 2014; 15(10): 938–946, https://doi.org/10.1038/ni.2984.
- Ericson J.A., Duffau P., Yasuda K., Ortiz-Lopez A., Rothamel K., Rifkin I.R., Monach P.A.; ImmGen Consortium. Gene expression during the generation and activation of mouse neutrophils: implication of novel functional and regulatory pathways. PLoS One 2014; 9(10): e108553, https://doi.org/10.1371/journal.pone.0108553.
- Manz M.G., Boettcher S. Emergency granulopoiesis. Nat Rev Immunol 2014; 14(5): 302–314, https://doi.org/10.1038/nri3660.
- Белобородова Н.В., Мороз В.В., Бедова А.Ю., Осипов А.А., Саршор Ю.Н., Черневская Е.А. Участие ароматических микробных метаболитов в развитии тяжелой инфекции и сепсиса. Анестезиология и реаниматология 2016; 61(3): 202–208.
- Silvestre-Roig C., Hidalgo A., Soehnlein O. Neutrophil heterogeneity: implications for homeostasis and pathogenesis. Blood 2016; 127(18): 2173–2181, https://doi.org/10.1182/blood-2016-01-688887.
- Kim M.H., Yang D., Kim M., Kim S.Y., Kim D., Kang S.J. A late-lineage murine neutrophil precursor population exhibits dynamic changes during demand-adapted granulopoiesis. Sci Rep 2017; 7: 39804, https://doi.org/10.1038/srep39804.
- Dinsdale R.J. Production and impaired regulation of neutrophil extracellular traps following severe thermal injury, implications for sepsis and multiple organ failure. University of Birmingham Research Archive; 2017. URL: http://etheses.bham.ac.uk/7958/1/Dinsdale17PhD.pdf.
- Strydom N., Rankin S.M. Regulation of circulating neutrophil numbers under homeostasis and in disease. J Innate Immun 2013; 5(4): 304–314, https://doi.org/10.1159/000350282.
- Rosales C. Neutrophil: a cell with many roles in inflammation or several cell types? Front Physiol 2018; 9: 113, https://doi.org/10.3389/fphys.2018.00113.
- McDermott D.H., Liu Q., Ulrick J., Kwatemaa N., Anaya-O’Brien S., Penzak S.R., Filho J.O., Priel D.A., Kelly C., Garofalo M., Littel P., Marquesen M.M., Hilligoss D., Decastro R., Fleisher T.A., Kuhns D.B., Malech H.L., Murphy P.M. The CXCR4 antagonist plerixafor corrects panleukopenia in patients with WHIM syndrome. Blood 2011; 118(18): 4957–4962, https://doi.org/10.1182/blood-2011-07-368084.
- Lerman Y.V., Kim M. Neutrophil migration under normal and sepsis conditions. Cardiovasc Hematol Disord Drug Targets 2015; 15(1): 19–28, https://doi.org/10.2174/1871529x15666150108113236.
- Bohannon J.K., Luan L., Hernandez A., Afzal A., Guo Y., Patil N.K., Fensterheim B., Sherwood E.R. Role of G-CSF in monophosphoryl lipid A-mediated augmentation of neutrophil functions after burn injury. J Leukoc Biol 2015; 99(4): 629–640, https://doi.org/10.1189/jlb.4A0815-362R.
- Shen X.F., Cao K., Jiang J.P., Guan W.X., Du J.F. Neutrophil dysregulation during sepsis: an overview and update. J Cell Mol Med 2017; 21(9): 1687–1697, https://doi.org/10.1111/jcmm.13112.
- Delano M.J., Ward P.A. Sepsis-induced immune dysfunction: can immune therapies reduce mortality? J Clin Invest 2016; 126(1): 23–31, https://doi.org/10.1172/JCI82224.
- Hotchkiss R.S., Monneret G., Payen D. Sepsis-induced immunosuppression: from cellular dysfunctions to immunotherapy. Nat Rev Immunol 2013; 13(12): 862–874, https://doi.org/10.1038/nri3552.
- Guerin E., Orabona M., Raquil M.A., Giraudeau B., Bellier R., Gibot S., Béné M.C., Lacombe F., Droin N., Solary E., Vignon P., Feuillard J., François B. Circulating immature granulocytes with T-cell killing functions predict sepsis deterioration. Crit Care Med 2014; 42(9): 2007–2018, https://doi.org/10.1097/CCM.0000000000000344.
- Nierhaus A., Klatte S., Linssen J., Eismann N.M., Wichmann D., Hedke J., Braune S.A., Kluge S. Revisiting the white blood cell count: immature granulocytes count as a diagnostic marker to discriminate between SIRS and sepsis — a prospective, observational study. BMC Immunol 2013; 14: 8, https://doi.org/10.1186/1471-2172-14-8.
- Hampson P., Dinsdale R.J., Wearn C.M., Bamford A.L., Bishop J.R.B., Hazeldine J., Moiemen N.S., Harrison P., Lord J.M. Neutrophil dysfunction, immature granulocytes, and cell-free DNA are early biomarkers of sepsis in burn-injured patients: a prospective observational cohort study. Ann Surg 2017; 265(6): 1241–1249, https://doi.org/10.1097/SLA.0000000000001807.
- Drifte G., Dunn-Siegrist I., Tissieres P., Pugin J. Innate immune functions of immature neutrophils in patients with sepsis and severe systemic inflammatory response syndrome. Crit Care Med 2013; 41(3): 820–832, https://doi.org/10.1097/CCM.0b013e318274647d.
- Галкин А.А., Демидова В.С. Повреждение защитных функций нейтрофилов на ранней стадии ожоговой болезни. Успехи современной биологии 2012; 132(3): 297–311.
- Kasperkiewicz P., Poreba M., Snipas S.J., Lin S.J., Kirchhofer D., Salvesen G.S., Drag M. Design of a selective substrate and activity based probe for human neutrophil serine protease 4. PLоS One 2015; 10(7): e0132818, https://doi.org/10.1371/journal.pone.0132818.
- Stapels D.A., Geisbrecht B.V., Rooijakkers S.H. Neutrophil serine proteases in antibacterial defense. Curr Opin Microbiol 2015; 23: 42–48, https://doi.org/10.1016/j.mib.2014.11.002.
- Martin L., van Meegern A., Doemming S., Schuerholz T. Antimicrobial peptides in human sepsis. Front Immunol 2015; 6: 404, https://doi.org/10.3389/fimmu.2015.00404.
- Clark H.L., Jhingran A., Sun Y., Vareechon C., de Jesus Carrion S., Skaar E.P., Chazin W.J., Calera J.A., Hohl T.M., Pearlman E. Zinc and manganese chelation by neutrophil S100A8/A9 (Calprotectin) limits extracellular aspergillus fumigatus hyphal growth and corneal infection. J Immunol 2016; 196(1): 336–344, https://doi.org/10.4049/jimmunol.1502037.
- Embleton N.D., Berrington J.E., McGuire W., Stewart C.J., Cummings S.P. Lactoferrin: antimicrobial activity and therapeutic potential. Semin Fetal Neonatal Med 2013; 18(3): 143–149, https://doi.org/10.1016/j.siny.2013.02.001.
- Mayeur S., Spahis S., Pouliot Y., Levy E. Lactoferrin, a pleiotropic protein in health and disease. Antioxid Redox Signal 2016; 24(14): 813–836, https://doi.org/10.1089/ars.2015.6458.
- Mahlapuu M., Håkansson J., Ringstad L., Björn C. Antimicrobial peptides: an emerging category of therapeutic agents. Front Cell Infect Microbiol 2016; 6: 194, https://doi.org/10.3389/fcimb.2016.00194.
- Qiao J., Liu Z., Purro M., Xiong M.P. Antibacterial and potentiation properties of charge-optimized polyrotaxanes for combating opportunistic bacteria. J Mater Chem B 2018; 6(33): 5353–5361, https://doi.org/10.1039/C8TB01610K.
- Santajit S., Indrawattana N. Mechanisms of antimicrobial resistance in ESKAPE pathogens. Biomed Res Int 2016: 2475067, https://doi.org/10.1155/2016/2475067.
- Pfalzgraff A., Brandenburg K., Weindl G. Antimicrobial peptides and their therapeutic potential for bacterial skin infections and wounds. Front Pharmacol 2018; 9: 281, https://doi.org/10.3389/fphar.2018.00281.
- Azzopardi E.A., Azzopardi E., Camilleri L., Villapalos J., Boyce D.E., Dziewulski P., Dickson W.A., Whitaker I.S. Gram negative wound infection in hospitalised adult burn patients-systematic review and metanalysis. PLoS One 2014; 9(4): e95042, https://doi.org/10.1371/journal.pone.0095042.
- Selders G.S., Fetz A.E., Radic M.Z., Bowlin G.L. An overview of the role of neutrophils in innate immunity, inflammation and host-biomaterial integration. Regen Biomater 2017; 4(1): 55–68, https://doi.org/10.1093/rb/rbw041.
- Zawrotniak M., Rapala-Kozik M. Neutrophil extracellular traps (NETs) — formation and implications. Acta Biochim Po 2013; 60(3): 277–284.
- Yipp B.G., Kubes P. NETosis: how vital is it? Blood 2013; 122(16): 2784–2794, https://doi.org/10.1182/blood-2013-04-457671.
- Li Y., Liu B., Fukudome E.Y., Lu J., Chong W., Jin G., Liu Z., Velmahos G.C., Demoya M., King D.R., Alam H.B. Identification of citrullinated histone H3 as a potential serum protein biomarker in a lethal model of lipopolysaccharide-induced shock. Surgery 2011; 150(3): 442–451, https://doi.org/10.1016/j.surg.2011.07.003.
- Remijsen Q., Kuijpers T.W., Wirawan E., Lippens S., Vandenabeele P., Vanden Berghe T. Dying for a cause: NETosis, mechanisms behind an antimicrobial cell death modality. Cell Death Differ 2011; 18(4): 581–588, https://doi.org/10.1038/cdd.2011.1.
- Dwivedi D.J., Toltl L.J., Swystun L.L., Pogue J., Liaw K.L., Weitz J.I., Cook D.J., Fox-Robichaud A.E., Liaw P.C.; Canadian Critical Care Translational Biology Group. Prognostic utility and characterization of cell-free DNA in patients with severe sepsis. Crit Care 2012; 16(4): R151, https://doi.org/10.1186/cc11466.
- Hirose T., Hamaguchi S., Matsumoto N., Irisawa T., Seki M., Tasaki O., Hosotsubo H., Yamamoto N., Yamamoto K., Akeda Y., Oishi K., Tomono K., Shimazu T. Presence of neutrophil extracellular traps and citrullinated histone H3 in the bloodstream of critically ill patients. PLoS One 2014; 9(11): e111755, https://doi.org/10.1371/journal.pone.0111755.
- De Backer D., Orbegozo Cortes D., Donadello K., Vincent J.L. Pathophysiology of microcirculatory dysfunction and the pathogenesis of septic shock. Virulence 2014; 5(1): 73–79, https://doi.org/10.4161/viru.26482.
- Kovach M.A., Standiford T.J. The function of neutrophils in sepsis. Curr Opin Infect Dis 2012; 25(3): 321–327, https://doi.org/10.1097/QCO.0b013e3283528c9b.
- Wang X., Qin W., Sun B. New strategy for sepsis: targeting a key role of platelet-neutrophil interaction. Burns Trauma 2014; 2(3): 114–120, https://doi.org/10.4103/2321-3868.135487.
- Hietbrink F., Koenderman L., Althuizen M., Pillay J., Kamp V., Leenen L.P. Kinetics of the innate immune response after trauma: implications for the development of late onset sepsis. Shock 2013; 40(1): 21–27, https://doi.org/10.1097/shk.0b013e318295a40a.
- Osterbur K., Mann F.A., Kuroki K., DeClue A. Multiple organ dysfunction syndrome in humans and animals. J Vet Intern Med 2014; 28(4): 1141–1151, https://doi.org/10.1111/jvim.12364.
- Yago T., Liu Z., Ahamed J., McEver R.P. Cooperative PSGL-1 and CXCR2 signaling in neutrophils promotes deep vein thrombosis in mice. Blood 2018; 132(13): 1426–1437, https://doi.org/10.1182/blood-2018-05-850859.
- Rahman M., Zhang S., Chew M., Syk I., Jeppsson B., Thorlacius H. Platelet shedding of CD40L is regulated by matrix metalloproteinase-9 in abdominal sepsis. J Thromb Haemost 2013; 11(7): 1385–98, https://doi.org/10.1111/jth.12273.
- Kaplan M.J., Radic M. Neutrophil extracellular traps: double-edged swords of innate immunity. J Immunol 2012; 189(6): 2689–2695, https://doi.org/10.4049/jimmunol.1201719.
- McDonald B., Urrutia R., Yipp B.G., Jenne C.N., Kubes P. Intravascular neutrophil extracellular traps capture bacteria from the bloodstream during sepsis. Cell Host Microbe 2012; 12(3): 324–333, https://doi.org/10.1016/j.chom.2012.06.011.
- Derive M., Bouazza Y., Sennoun N., Marchionni S., Quigley L., Washington V., Massin F., Max J.P., Ford J., Alauzet C., Levy B., McVicar D.W., Gibot S. Soluble TREM-like transcript-1 regulates leukocyte activation and controls microbial sepsis. J Immunol 2012; 188(11): 5585–5592, https://doi.org/10.4049/jimmunol.1102674.
- Butler K.L., Ambravaneswaran V., Agrawal N., Bilodeau M., Toner M., Tompkins R.G., Fagan S., Irimia D. Burn injury reduces neutrophil directional migration speed in microfluidic devices. PLoS One 2010; 5(7): e11921, https://doi.org/10.1371/journal.pone.0011921.
- Jones C.N., Moore M., Dimisko L., Alexander A., Ibrahim A., Hassell B.A., Warren H.S., Tompkins R.G., Fagan S.P., Irimia D. Spontaneous neutrophil migration patterns during sepsis after major burns. PLoS One 2014; 9(12): e114509, https://doi.org/10.1371/journal.pone.0114509.
- Ellett F., Jorgensen J., Marand A.L., Liu Y.M., Martinez M.M., Sein V., Butler K.L., Lee J., Irimia D. Diagnosis of sepsis from a drop of blood by measurement of spontaneous neutrophil motility in a microfluidic assay. Nat Biomed Eng 2018; 2(4): 207–214, https://doi.org/10.1038/s41551-018-0208-z.
- Zhang F., Liu A.L., Gao S., Ma S., Guo S.B. Neutrophil dysfunction in sepsis. Chin Med J 2016; 129(22): 2741–2744, https://doi.org/10.4103/0366-6999.193447.
- Spiller F., Costa C., Souto F.O., Vinchi F., Mestriner F.L., Laure H.J., Alves-Filho J.C., Freitas A., Rosa J.C., Ferreira S.H., Altruda F., Hirsch E., Greene L.J., Tolosano E., Cunha F. Inhibition of neutrophil migration by hemopexin leads to increased mortality due to sepsis in mice. Am J Respir Crit Care Med 2011; 183(7): 922–931, https://doi.org/10.1164/rccm.201002-0223OC.
- Bzowska M., Hamczyk M., Skalniak A., Guzik K. Rapid decrease of CD16 (FcγRIII) expression on heat-shocked neutrophils and their recognition by macrophages. J Biomed Biotechnol 2011; 2011: 284759, https://doi.org/10.1155/2011/284759.
- Adams M.N., Ramachandran R., Yau M.K., Suen J.Y., Fairlie D.P., Hollenberg M.D., Hooper J.D. Structure, function and pathophysiology of protease activated receptors. Pharmacol Ther 2011; 130(3): 248–282, https://doi.org/10.1016/j.pharmthera.2011.01.003.
- van den Berg C.W., Tambourgi D.V., Clark H.W., Hoong S.J., Spiller O.B., McGreal E.P. Mechanism of neutrophil dysfunction: neutrophil serine proteases cleave and inactivate the C5a receptor. J Immunol 2014; 192(4): 1787–1795, https://doi.org/10.4049/jimmunol.1301920.
- Goh C., Narayanan S., Hahn Y.S. Myeloid-derived suppressor cells: the dark knight or the joker in viral infections? Immunol Rev 2013; 255(1): 210–221, https://doi.org/10.1111/imr.12084.
- Leliefeld P.H., Wessels C.M., Leenen L.P., Koenderman L., Pillay J. The role of neutrophils in immune dysfunction during severe inflammation. Crit Care 2016; 20: 73, https://doi.org/10.1186/s13054-016-1250-4.
- Tak T., Wijten P., Heeres M., Pickkers P., Scholten A., Heck A.J.R., Vrisekoop N., Leenen L.P., Borghans J.A.M., Tesselaar K., Koenderman L. Human CD62Ldim neutrophils identified as a separate subset by proteome profiling and in vivo pulse-chase labeling. Blood 2017; 129(26): 3476–3485, https://doi.org/10.1182/blood-2016-07-727669.
- Visser T., Pillay J., Pickkers P., Leenen L.P., Koenderman L. Homology in systemic neutrophil response induced by human experimental endotoxemia and by trauma. Shock 2012; 37(2): 145–151, https://doi.org/10.1097/SHK.0b013e31823f14a4.
- Pillay J., Kamp V.M., van Hoffen E., Visser T., Tak T., Lammers J.W., Ulfman L.H., Leenen L.P., Pickkers P., Koenderman L. A subset of neutrophils in human systemic inflammation inhibits T cell responses through Mac-1. J Clin Invest 2012; 122(1): 327–336, https://doi.org/10.1172/JCI57990.
- Kamp V.M., Pillay J., Lammers J.W., Pickkers P., Ulfman L.H., Koenderman L. Human suppressive neutrophils CD16bright/CD62Ldim exhibit decreased adhesion. J Leukoc Biol 2012; 92(5): 1011–1020, https://doi.org/10.1189/jlb.0612273.
- de Kleijn S., Langereis J.D., Leentjens J., Kox M., Netea M.G., Koenderman L., Ferwerda G., Pickkers P., Hermans P.W. IFN-γ-stimulated neutrophils suppress lymphocyte proliferation through expression of PD-L1. PLoS One 2013; 8(8): e72249, https://doi.org/10.1371/journal.pone.0072249.
- Liu Q., Li C.S. Programmed cell death-1/programmed death-ligand 1 pathway: a new target for sepsis. Chin Med J 2017; 130(8): 986–992, https://doi.org/10.4103/0366-6999.204113.
- Hampton H.R., Bailey J., Tomura M., Brink R., Chtanova T. Microbe-dependent lymphatic migration of neutrophils modulates lymphocyte proliferation in lymph nodes. Nat Commun 2015; 6: 7139, https://doi.org/10.1038/ncomms8139.
- Kamenyeva O., Boularan C., Kabat J., Cheung G.Y., Cicala C., Yeh A.J., Chan J.L., Periasamy S., Otto M., Kehrl J.H. Neutrophil recruitment to lymph nodes limits local humoral response to Staphylococcus aureus. PLoS Pathog 2015; 11(4): e1004827, https://doi.org/10.1371/journal.ppat.1004827.
- Gabrilovich D.I., Ostrand-Rosenberg S., Bronte V. Coordinated regulation of myeloid cells by tumours. Nat Rev Immunol 2012; 12(4): 253–268, https://doi.org/10.1038/nri3175.
- Darcy C.J., Minigo G., Piera K.A., Davis J.S., McNeil Y.R., Chen Y., Volkheimer A.D., Weinberg J.B., Anstey N.M., Woodberry T. Neutrophils with myeloid derived suppressor function deplete arginine and constrain T cell function in septic shock patients. Crit Care 2014; 8(4): R163, https://doi.org/10.1186/cc14003.
- Xiu F., Jeschke M.G. Perturbed mononuclear phagocyte system in severely burned and septic patients. Shock 2013; 40(2): 81–88, https://doi.org/10.1097/SHK.0b013e318299f774.
- Murray P.J., Wynn T.A. Protective and pathogenic functions of macrophage subsets. Nat Rev Immunol 2011; 11(11): 723–37, https://doi.org/10.1038/nri3073.
- Ito I., Asai A., Suzuki S., Kobayashi M., Suzuki F. M2b macrophage polarization accompanied with reduction of long noncoding RNA GAS5. Biochem Biophys Res Commun 2017; 493(1): 170–175, https://doi.org/10.1016/j.bbrc.2017.09.053.
- Sica A., Mantovani A. Macrophage plasticity and polarization: in vivo veritas. J Clin Invest 2012; 122(3): 787–795, https://doi.org/10.1172/JCI59643.
- Krzyszczyk P., Schloss R., Palmer A., Berthiaume F. The role of macrophages in acute and chronic wound healing and interventions to promote pro-wound healing phenotypes. Front Physiol 2018; 9: 419, https://doi.org/10.3389/fphys.2018.00419.
- Porta C., Riboldi E., Ippolito A., Sica A. Molecular and epigenetic basis of macrophage polarized activation. Semin Immunol 2015; 27: 237–248, https://doi.org/10.1016/j.smim.2015.10.003.
- Lateef Z., Stuart G., Jones N., Mercer A., Fleming S., Wise L. The cutaneous inflammatory response to thermal burn injury in a murine model. Int J Mol Sci 2019; 20(3): E538, https://doi.org/10.3390/ijms20030538.
- Laskin D.L., Sunil V.R., Gardner C.R., Laskin J.D. Macrophages and tissue injury: agents of defense or destruction? Annu Rev Pharmacol Toxicol 2011; 51: 267–288, https://doi.org/10.1146/annurev.pharmtox.010909.105812.
- Kobayashi M., Toliver-Kinsky T., Suzuki F. Host defense antibacterial effector cells influenced by massive burns. In: Total burn care. Elsevier; 2018; p. 221–231, https://doi.org/10.1016/b978-0-323-47661-4.00020-4.
- Hume D.A. The many alternative faces of macrophage activation. Front Immunol 2015; 6: 370, https://doi.org/10.3389/fimmu.2015.00370.
- Wang L.X., Zhang S.X., Wu H.J., Rong X.L., Guo J. M2b macrophage polarization and its roles in diseases. J Leukoc Biol 2019; 106(2): 345–358, https://doi.org/10.1002/JLB.3RU1018-378RR.
- Лямина С.В., Малышев И.Ю. Поляризация макрофагов в современной концепции формирования иммунного ответа. Фундаментальные исследования 2014; 10–5: 930–935.
- Martinez F.O., Gordon S. The M1 and M2 paradigm of macrophage activation: time for reassessment. F1000Prime Rep 2014; 6: 13, https://doi.org/10.12703/P6-13.
- Ogle M.E., Segar C.E., Sridhar S., Botchwey E.A. Monocytes and macrophages in tissue repair: implications for immunoregenerative biomaterial design. Exp Biol Med (Maywood) 2016; 241(10): 1084–1097, https://doi.org/10.1177/1535370216650293.
- Kobayashi M., Jeschke M.G., Shigematsu K., Asai A., Yoshida S., Herndon D.N., Suzuki F. M2b monocytes predominated in peripheral blood of severely burned patients. J Immunol 2010; 185(12): 7174–7179, https://doi.org/10.4049/jimmunol.0903935.
- Сарбаева Н.Н., Пономарева Ю.В., Милякова М.Н. Макрофаги: разнообразие фенотипов и функций, взаимодействие с чужеродными материалами. Гены и клетки 2016; 11(1): 9–17.
- Ito I., Bhopale K.K., Nishiguchi T., Lee J.O., Herndon D.N., Suzuki S., Sowers L.C., Suzuki F., Kobayashi M. The polarization of M2b monocytes in cultures of burn patient peripheral CD14+ cells treated with a selected human CCL1 antisense oligodeoxynucleotide. Nucleic Acid Ther 2016; 26(5): 269–276, https://doi.org/10.1089/nat.2016.0617.
- Nishiguchi T., Ito I., Lee J.O., Suzuki S., Suzuki F., Kobayashi M. Macrophage polarization and MRSA infection in burned mice. Immunol Cell Biol 2017; 95(2): 198–206, https://doi.org/10.1038/icb.2016.84.