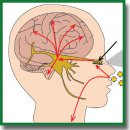
Молекулярные механизмы белков-мишеней для SARS-CoV-2 (обзор)
Быстро меняющаяся информация о новой коронавирусной инфекции и неоднозначные результаты, получаемые из различных источников, обусловливают необходимость проведения дополнительных исследований для разработки специфической профилактики и терапии этого заболевания. На данный момент имеется достаточно сведений о том, что возбудитель поражает не только дыхательную, но и центральную нервную систему.
Цель исследования — проанализировать информацию о молекулярных механизмах развития поражения центральной нервной системы при новой коронавирусной инфекции COVID-19, вызываемой вирусом SARS-CoV-2.
Результаты. Показано, что головной мозг является органом-мишенью для коронавирусной инфекции. SARS-CoV-2 попадает в организм через белки-мишени: ангиотензинпревращающий фермент 2 (ACE2), ассоциированную сериновую протеазу TMPRSS2 эпителия носовой полости. Поражение головного мозга развивается до появления легочной симптоматики. Вирус распространяется по ткани мозга в пириформную кору, базальные ганглии, средний мозг, гипоталамус. Позже поражаются черная субстанция среднего мозга, миндалевидное тело, гиппокамп и мозжечок. Наблюдается интенсивная гибель нейронов, развитие астроглиоза и активация микроглии. К 4-м суткам регистрируются выраженная гиперпродукция провоспалительных цитокинов в ткани головного мозга и развитие выраженного локального нейровоспаления, повышение проницаемости гематоэнцефалического барьера и повреждение механизмов нейропластичности, подразумевающих обязательное наличие васкулярного компонента в патогенезе заболевания. Последнее может быть обусловлено избыточной активностью матриксных металлопротеиназ, опосредованной через активность CD147. Основными участниками патогенеза в ткани головного мозга при COVID-19 являются продукты каталитической активности ангиотензина II (АТ II), в частности ангиотензин 1-7 (АТ 1-7) и ангиотензин IV (АТ IV). Имеются противоречивые данные в отношении их роли в развитии повреждения центральной нервной системы при различных заболеваниях, в том числе и при коронавирусной инфекции.
Вторым участником патогенеза поражения головного мозга при COVID-19 является индуктор внеклеточных матриксных металлопротеиназ (CD147). Эта молекула экспрессируется на клетках эндотелия церебральных микрососудов, а также клетках лейкоцитарной природы, присутствующих в ткани головного мозга при нейровоспалении. Молекула CD147 имеет важное значение в регуляции структурно-функциональной целостности гематоэнцефалического барьера — опосредованно, через контроль проницаемости базальной мембраны и реализацию астроцит-эндотелиальных взаимодействий. Таким образом, воздействие SARS-CoV-2 приводит к непосредственному повреждению нейроваскулярной единицы головного мозга.
- Alenina N., Bader M. ACE2 in brain physiology and pathophysiology: evidence from transgenic animal models. Neurochem Res 2019; 44(6): 1323–1329, https://doi.org/10.1007/s11064-018-2679-4.
- Li H., Xue Q., Xu X. Involvement of the nervous system in SARS-CoV-2 infection. Neurotox Res 2020; 38(1): 1–7, https://doi.org/10.1007/s12640-020-00219-8.
- Heurich A., Hofmann-Winkler H., Gierer S., Liepold T., Jahn O., Pöhlmann S. TMPRSS2 and ADAM17 cleave ACE2 differentially and only proteolysis by TMPRSS2 augments entry driven by the severe acute respiratory syndrome coronavirus spike protein. J Virol 2014; 88(2): 1293–1307, https://doi.org/10.1128/jvi.02202-13.
- Ulrich H., Pillat M.M. CD147 as a target for COVID-19 treatment: suggested effects of azithromycin and stem cell engagement. Stem Cell Rev Rep 2020; 16(3): 434–440, https://doi.org/10.1007/s12015-020-09976-7.
- Hoffmann M., Kleine-Weber H., Schroeder S., Krüger N., Herrler T., Erichsen S., Schiergens T.S., Herrler G., Wu N.H., Nitsche A., Müller M.A., Drosten C., Pöhlmann S. SARS-CoV-2 cell entry depends on ACE2 and TMPRSS2 and is blocked by a clinically proven protease inhibitor. Cell 2020; 181(2): 271–280.e8, https://doi.org/10.1016/j.cell.2020.02.052.
- Chen Z., Mi L., Xu J., Yu J., Wang X., Jiang J., Xing J., Shang P., Qian A., Li Y., Shaw P.X., Wang J., Duan S., Ding J., Fan C., Zhang Y., Yang Y., Yu X., Feng Q., Li B., Yao X., Zhang Z., Li L., Xue X., Zhu P. Function of HAb18G/CD147 in invasion of host cells by severe acute respiratory syndrome coronavirus. J Infect Dis 2005; 191(5): 755–760, https://doi.org/10.1086/427811.
- Mao L., Jin H., Wang M., Hu Y., Chen S., He Q., Chang J., Hong C., Zhou Y., Wang D., Miao X., Li Y., Hu B. Neurologic manifestations of hospitalized patients with Coronavirus Disease 2019 in Wuhan, China. JAMA Neurol 2020; 77(6): 683–690, https://doi.org/10.1001/jamaneurol.2020.1127.
- Li Y.C., Bai W.Z., Hashikawa T. Response to commentary on “The neuroinvasive potential of SARS-CoV-2 may play a role in the respiratory failure of COVID-19 patients”. J Medic Virol 2020; 92(7): 707–709, https://doi.org/10.1002/jmv.25824.
- Pranata R., Huang I., Lim M.A., Wahjoepramono P.E.J., July J. Impact of cerebrovascular and cardiovascular diseases on mortality and severity of COVID-19 — systematic review, meta-analysis, and meta-regression. J Stroke Cerebrovasc Dis 2020; 29(8): 104949, https://doi.org/10.1016/j.jstrokecerebrovasdis.2020.104949.
- Afshar H., Yassin Z., Kalantari S., Aloosh O., Lotfi T., Moghaddasi M., Sadeghipour A., Emamikhah M. Evolution and resolution of brain involvement associated with SARS-CoV2 infection: a close clinical — paraclinical follow up study of a case. Mult Scler Relat Disord 2020; 43: 102216, https://doi.org/10.1016/j.msard.2020.102216.
- Lai C.C., Ko W.C., Lee P.I., Jean S.S., Hsueh P.R. Extra-respiratory manifestations of COVID-19. Int J Antimicrob Agents 2020; 56(2): 106024, https://doi.org/10.1016/j.ijantimicag.2020.106024.
- Matías-Guiu J., Gomez-Pinedo U., Montero-Escribano P., Gomez-Iglesias P., Porta-Etessam J., Matias-Guiu J.A. Should we expect neurological symptoms in the SARS-CoV-2 epidemic? Neurologia 2020; 35(3): 170–175, https://doi.org/10.1016/j.nrl.2020.03.001.
- Netland J., Meyerholz D.K., Moore S., Cassell M., Perlman S. Severe acute respiratory syndrome coronavirus infection causes neuronal death in the absence of encephalitis in mice transgenic for human ACE2. J Virol 2008; 82(15): 7264–7275, https://doi.org/10.1128/jvi.00737-08.
- Yamashita M., Yamate M., Li G.M., Ikuta K. Susceptibility of human and rat neural cell lines to infection by SARS-coronavirus. Biochem Biophys Res Commun 2005; 334(1): 79–85, https://doi.org/10.1016/j.bbrc.2005.06.061.
- Brann D.H., Tsukahara T., Weinreb C., Lipovsek M., Van den Berge K., Gong B., Chance R., Macaulay I.C., Chou H.J., Fletcher R., Das D., Street K., Bezieux H., Choi Y.G., Risso D., Dudoit S., Purdom E., Mill J., Hachem R.A., Matsunami H., Logan D.W., Goldstein B.J., Grubb M.S., Ngai J., Datta S.R. Non-neuronal expression of SARS-CoV-2 entry genes in the olfactory system suggests mechanisms underlying COVID-19-associated anosmia: preprint. Sci Adv 2020; 6(31): eabc5801, https://doi.org/10.1101/2020.03.25.009084.
- Li Y.C., Bai W.Z., Hashikawa T. The neuroinvasive potential of SARS-CoV2 may play a role in the respiratory failure of COVID-19 patients. J Medic Virol 2020; 92(6): 552–555, https://doi.org/10.1002/jmv.25728.
- Baig A.M., Khaleeq A., Ali U., Syeda H. Evidence of the COVID-19 virus targeting the CNS: tissue distribution, host-virus interaction, and proposed neurotropic mechanisms. ACS Chem Neurosci 2020; 11(7): 995–998, https://doi.org/10.1021/acschemneuro.0c00122.
- Giacomelli A., Pezzati L., Conti F., Bernacchia D., Siano M., Oreni L., Rusconi S., Gervasoni C., Ridolfo A.L., Rizzardini G., Antinori S., Galli M. Self-reported olfactory and taste disorders in SARS-CoV-2 patients: a cross-sectional study. Clin Infect Dis 2020; 71(15): 889–890, https://doi.org/10.1093/cid/ciaa330.
- Butowt R., Bilinska K. SARS-CoV-2: olfaction, brain infection, and the urgent need for clinical samples allowing earlier virus detection. ACS Chem Neurosci 2020; 11(9): 1200–1203, https://doi.org/10.1021/acschemneuro.0c00172.
- Lu R., Zhao X., Li J., Niu P., Yang B., Wu H., Wang W., Song H., Huang B., Zhu N., Bi Y., Ma X., Zhan F., Wang L., Hu T., Zhou H., Hu Z., Zhou W., Zhao L., Chen J., Meng Y., Wang J., Lin Y., Yuan J., Xie Z., Ma J., Liu W.J., Wang D., Xu W., Holmes E.C., Gao G.F., Wu G., Chen W., Shi W., Tan W. Genomic characterisation and epidemiology of 2019 novel coronavirus: implications for virus origins and receptor binding. Lancet 2020; 395(10224): 565–574, https://doi.org/10.1016/s0140-6736(20)30251-8.
- Lan J., Ge J., Yu J., Shan S., Zhou H., Fan S., Zhang Q., Shi X., Wang Q., Zhang L., Wang X. Structure of the SARS-CoV-2 spike receptor-binding domain bound to the ACE2 receptor. Nature 2020; 581(7807): 215–220, https://doi.org/10.1038/s41586-020-2180-5.
- Chen I.Y., Moriyama M., Chang M.F., Ichinohe T. Severe acute respiratory syndrome coronavirus viroporin 3a activates the NLRP3 inflammasome. Front Microbiol 2019; 10: 50, https://doi.org/10.3389/fmicb.2019.00050.
- Shi C.S., Nabar N.R., Huang N.N., Kehrl J.H. SARS-coronavirus open reading frame-8b triggers intracellular stress pathways and activates NLRP3 inflammasomes. Cell Death Discov 2019; 5: 101, https://doi.org/10.1038/s41420-019-0181-7.
- Luo C., Luo H., Zheng S., Gui C., Yue L., Yu C., Sun T., He P., Chen J., Shen J., Luo X., Li Y., Liu H., Bai D., Shen J., Yang Y., Li F., Zuo J., Hilgenfeld R., Pei G., Chen K., Shen X., Jiang H. Nucleocapsid protein of SARS coronavirus tightly binds to human cyclophilin A. Biochem Biophys Res Commun 2004; 321(3): 557–565, https://doi.org/10.1016/j.bbrc.2004.07.003.
- Kuba K., Imai Y., Rao S., Gao H., Guo F., Guan B., Huan Y., Yang P., Zhang Y., Deng W., Bao L., Zhang B., Liu G., Wang Z., Chappell M., Liu Y., Zheng D., Leibbrandt A., Wada T., Slutsky A.S., Liu D., Qin C., Jiang C., Penninger J.M. A crucial role of angiotensin converting enzyme 2 (ACE2) in SARS coronavirus-induced lung injury. Nat Med 2005; 11(8): 875–879, https://doi.org/10.1038/nm1267.
- de Wilde A.H., Pham U., Posthuma C.C., Snijder E.J. Cyclophilins and cyclophilin inhibitors in nidovirus replication. Virology 2018; 522: 46–55, https://doi.org/10.1016/j.virol.2018.06.011.
- von Brunn A., Ciesek S., von Brunn B., Carbajo-Lozoya J. Genetic deficiency and polymorphisms of cyclophilin A reveal its essential role for Human Coronavirus 229E replication. Curr Opin Virol 2015; 14: 56–61, https://doi.org/10.1016/j.coviro.2015.08.004.
- Tian L., Liu W., Sun L. Role of cyclophilin A during coronavirus replication and the antiviral activities of its inhibitors. Sheng Wu Gong Cheng Xue Bao 2020; 36(4): 605–611, https://doi.org/10.13345/j.cjb.200049.
- Zehendner C.M., White R., Hedrich J., Luhmann H.J. A neurovascular blood-brain barrier in vitro model. Methods Mol Biol 2014; 1135: 403–413, https://doi.org/10.1007/978-1-4939-0320-7_33.
- Мельникова Ю.С., Макарова Т.П. Эндотелиальная дисфункция как центральное звено патогенеза хронических болезней. Казанский медицинский журнал 2015; 96(4): 659–665.
- Jullienne A., Badaut J. Molecular contributions to neurovascular unit dysfunctions after brain injuries: lessons for target-specific drug development. Future Neurol 2013; 8(6): 677–689, https://doi.org/10.2217/fnl.13.55.
- Моргун А.В., Кувачева Н.В., Хилажева Е.Д., Пожиленкова Е.А., Салмина А.Б. Изучение метаболического сопряжения и межклеточных взаимодействий на модели нейроваскулярной единицы in vitro. Сибирское медицинское обозрение 2015; 1: 28–31.
- Бойцова Е.Б., Моргун А.В., Мартынова Г.П., Тохидпур A., Писарева Н.В., Рузаева В.А., Салмина А.Б. GPR81 рецепторы лактата в регуляции функциональной активности клеток. Сибирское медицинское обозрение 2016; 5: 15–23.
- Успенская Ю.А., Комлева Ю.К., Горина Я.В., Пожиленкова Е.А., Белова О.А., Салмина А.Б. Полифункциональность CD147 и новые возможности для диагностики и терапии. Сибирское медицинское обозрение 2018; 4: 22–30.
- Моргун А.В., Осипова Е.Д., Бойцова Е.Б., Лопатина О.Л., Горина Я.В., Пожиленкова Е.А., Салмина А.Б. Васкулярный компонент нейровоспаления при экспериментальной болезни Альцгеймера у мышей. Цитология 2020; 62(1): 16–23.
- Bostancıklıoğlu M. SARS-CoV2 entry and spread in the lymphatic drainage system of the brain. Brain Behav Immun 2020; 87: 122–123, https://doi.org/10.1016/j.bbi.2020.04.080.
- Bostancıklıoğlu M. Temporal correlation between neurological and gastrointestinal symptoms of SARS-CoV-2. Inflamm Bowel Dis 2020; 26(8): e89-e91, https://doi.org/10.1093/ibd/izaa131.
- Cabirac G.F., Murray R.S., McLaughlin L.B., Skolnick D.M., Hogue B., Dorovini-Zis K., Didier P.J. In vitro interaction of coronaviruses with primate and human brain microvascular endothelial cells. Adv Exp Med Biol 1995; 380: 79–88, https://doi.org/10.1007/978-1-4615-1899-0_11.
- Bleau C., Filliol A., Samson M., Lamontagne L. Brain invasion by mouse hepatitis virus depends on impairment of tight junctions and beta interferon production in brain microvascular endothelial cells. J Virol 2015; 89(19): 9896–9908, https://doi.org/10.1128/jvi.01501-15.
- South A.M., Diz D.I., Chappell M.C. COVID-19, ACE2, and the cardiovascular consequences. Am J Physiol Heart Circ Physiol 2020; 318(5): H1084–H1090, https://doi.org/10.1152/ajpheart.00217.2020.
- Magrone T., Magrone M., Jirillo E. Focus on receptors for coronaviruses with special reference to angiotensin-converting enzyme 2 as a potential drug target — a perspective. Endocr Metab Immune Disord Drug Targets 2020; 20(6): 807–811, https://doi.org/10.2174/1871530320666200427112902.
- Zangrillo A., Landoni G., Beretta L., Morselli F., Serpa Neto A., Bellomo R; COVID-BioB Study Group. Angiotensin II infusion in COVID-19-associated vasodilatory shock: a case series. Crit Care 2020; 24(1): 227, https://doi.org/10.1186/s13054-020-02928-0.
- Xia H., Lazartigues E. Angiotensin-converting enzyme 2 in the brain: properties and future directions. J Neurochem 2008; 107(6): 1482–1494, https://doi.org/10.1111/j.1471-4159.2008.05723.x.
- Clarke N.E., Turner A.J. Angiotensin-converting enzyme 2: the first decade. Int J Hypertens 2012; 2012: 307315, https://doi.org/10.1155/2012/307315.
- Moccia F., Gerbino A., Lionetti V., Miragoli M., Munaron L.M., Pagliaro P., Pasqua T., Penna C., Rocca C., Samaja M., Angelone T. COVID-19-associated cardiovascular morbidity in older adults: a position paper from the Italian Society of Cardiovascular Researches. Geroscience 2020; 42(4): 1021–1049, https://doi.org/10.1007/s11357-020-00198-w.
- Perez S.E., Nadeem M., Malek-Ahmadi M.H., He B., Mufson E.J. Frontal cortex and hippocampal γ-secretase activating protein levels in prodromal Alzheimer disease. Neurodegener Dis 2017; 17(6): 235–241, https://doi.org/10.1159/000477937.
- Vickers C., Hales P., Kaushik V., Dick L., Gavin J., Tang J., Godbout K., Parsons T., Baronas E., Hsieh F., Acton S., Patane M., Nichols A., Tummino P. Hydrolysis of biological peptides by human angiotensin-converting enzyme-related carboxypeptidase. J Biol Chem 2002; 277(17): 14838–14843, https://doi.org/10.1074/jbc.m200581200.
- Guignabert C., de Man F., Lombès M. ACE2 as therapy for pulmonary arterial hypertension: the good outweighs the bad. Eur Respir J 2018; 51(6): 1800848, https://doi.org/10.1183/13993003.00848-2018.
- Bader M., Alenina N., Young D., Santos R.A.S., Touyz R.M. The meaning of Mas. Hypertension 2018; 72(5): 1072–1075, https://doi.org/10.1161/hypertensionaha.118.10918.
- Ohishi M., Yamamoto K., Rakugi H. Angiotensin (1–7) and other angiotensin peptides. Curr Pharm Des 2013; 19(17): 3060–3064, https://doi.org/10.2174/1381612811319170013.
- Ziegler C.G.K., Allon S.J., Nyquist S.K., Mbano I.M., Miao V.N., Tzouanas C.N., Cao Y., Yousif A.S., Bals J., Hauser B.M., Feldman J., Muus C., Wadsworth M.H. II, Kazer S.W., Hughes T.K., Doran B., Gatter G.J., Vukovic M., Taliaferro F., Mead B.E., Guo Z., Wang J.P., Gras D., Plaisant M., Ansari M., Angelidis I., Adler H., Sucre J.M.S., Taylor C.J., Lin B., Waghray A., Mitsialis V., Dwyer D.F., Buchheit K.M., Boyce J.A., Barrett N.A., Laidlaw T.M., Carroll S.L., Colonna L., Tkachev V., Peterson C.W., Yu A., Zheng H.B., Gideon H.P., Winchell C.G., Lin P.L., Bingle C.D., Snapper S.B., Kropski J.A., Theis F.J., Schiller H.B., Zaragosi L.E., Barbry P., Leslie A., Kiem H.P., Flynn J.L., Fortune S.M., Berger B., Finberg R.W., Kean L.S., Garber M., Schmidt A.G., Lingwood D., Shalek A.K., Ordovas-Montanes J.; HCA Lung Biological Network. SARS-CoV-2 receptor ACE2 is an interferon-stimulated gene in human airway epithelial cells and is detected in specific cell subsets across tissues. Cell 2020; 181(5): 1016–1035.e19, https://doi.org/10.1016/j.cell.2020.04.035.
- Peña Silva R.A., Chu Y., Miller J.D., Mitchell I.J., Penninger J.M., Faraci F.M., Heistad D.D. Impact of ACE2 deficiency and oxidative stress on cerebrovascular function with aging. Stroke 2012; 43(12): 3358–3363, https://doi.org/10.1161/strokeaha.112.667063.
- Nautiyal M., Arnold A.C., Chappell M.C., Diz D.I. The brain renin-angiotensin system and mitochondrial function: influence on blood pressure and baroreflex in transgenic rat strains. Int J Hypertens 2013; 2013: 136028, https://doi.org/10.1155/2013/136028.
- Wu J., Zhao D., Wu S., Wang D. Ang-(1–7) exerts protective role in blood-brain barrier damage by the balance of TIMP-1/MMP-9. Eur J Pharmacol 2015; 748: 30–36, https://doi.org/10.1016/j.ejphar.2014.12.007.
- Li X., Wang X., Xie J., Liang B., Wu J. Suppression of angiotensin-(1–7) on the disruption of blood-brain barrier in rat of brain glioma. Pathol Oncol Res 2019; 25(1): 429–435, https://doi.org/10.1007/s12253-018-0471-z.
- Wang J., Chen S., Bihl J. Exosome-mediated transfer of ACE2 (angiotensin-converting enzyme 2) from endothelial progenitor cells promotes survival and function of endothelial cell. Oxid Med Cell Longev 2020; 2020: 4213541, https://doi.org/10.1155/2020/4213541.
- Labandeira-Garcia J.L., Costa-Besada M.A., Labandeira C.M., Villar-Cheda B., Rodríguez-Perez A.I. Insulin-like growth factor-1 and neuroinflammation. Front Aging Neurosci 2017; 9: 365, https://doi.org/10.3389/fnagi.2017.00365.
- Wright J.W., Harding J.W. Contributions by the brain renin-angiotensin system to memory, cognition, and Alzheimer’s disease. J Alzheimers Dis 2019; 67(2): 469–480, https://doi.org/10.3233/jad-181035.
- Stragier B., De Bundel D., Sarre S., Smolders I., Vauquelin G., Dupont A., Michotte Y., Vanderheyden P. Involvement of insulin-regulated aminopeptidase in the effects of the renin-angiotensin fragment angiotensin IV: a review. Heart Fail Rev 2008; 13(3): 321–337, https://doi.org/10.1007/s10741-007-9062-x.
- Gard P.R. Cognitive-enhancing effects of angiotensin IV. BMC Neurosci 2008; 9(Suppl 2): S15, https://doi.org/10.1186/1471-2202-9-s2-s15.
- Zhang M.Y., Beyer C.E. Measurement of neurotransmitters from extracellular fluid in brain by in vivo microdialysis and chromatography-mass spectrometry. J Pharm Biomed Anal 2006; 40(3): 492–499, https://doi.org/10.1016/j.jpba.2005.07.025.
- Hamming I., Timens W., Bulthuis M.L., Lely A.T., Navis G., van Goor H. Tissue distribution of ACE2 protein, the functional receptor for SARS coronavirus. A first step in understanding SARS pathogenesis. J Pathol 2004; 203(2): 631–637, https://doi.org/10.1002/path.1570.
- Elased K.M., Cunha T.S., Marcondes F.K., Morris M. Brain angiotensin-converting enzymes: role of angiotensin-converting enzyme 2 in processing angiotensin II in mice. Exp Physiol 2008; 93(5): 665–675, https://doi.org/10.1113/expphysiol.2007.040311.
- Tikellis C., Thomas M.C. Angiotensin-converting enzyme 2 (ACE2) is a key modulator of the renin angiotensin system in health and disease. Int J Pept 2012; 2012: 256294, https://doi.org/10.1155/2012/256294.
- Evans C.E., Miners J.S., Piva G., Willis C.L., Heard D.M., Kidd E.J., Good M.A., Kehoe P.G. ACE2 activation protects against cognitive decline and reduces amyloid pathology in the Tg2576 mouse model of Alzheimer’s disease. Acta Neuropathol 2020; 139(3): 485–502, https://doi.org/10.1007/s00401-019-02098-6.
- Wright J.W., Kawas L.H., Harding J.W. A role for the brain RAS in Alzheimer’s and Parkinson’s diseases. Front Endocrinol (Lausanne) 2013; 4: 158, https://doi.org/10.3389/fendo.2013.00158.
- Gironacci M.M. Angiotensin-(1–7): beyond its central effects on blood pressure. Ther Adv Cardiovasc Dis 2015; 9(4): 209–216, https://doi.org/10.1177/1753944715599875.
- Kehoe P.G., Wong S., Al Mulhim N., Palmer L.E., Miners J.S. Angiotensin-converting enzyme 2 is reduced in Alzheimer’s disease in association with increasing amyloid-β and tau pathology. Alzheimers Res Ther 2016; 8(1): 50, https://doi.org/10.1186/s13195-016-0217-7.
- Miners J.S., Ashby E., Van Helmond Z., Chalmers K.A., Palmer L.E., Love S., Kehoe P.G. Angiotensin-converting enzyme (ACE) levels and activity in Alzheimer’s disease, and relationship of perivascular ACE-1 to cerebral amyloid angiopathy. Neuropathol Appl Neurobiol 2008; 34(2): 181–193, https://doi.org/10.1111/j.1365-2990.2007.00885.x.
- Liu S., Liu J., Miura Y., Tanabe C., Maeda T., Terayama Y., Turner A.J., Zou K., Komano H. Conversion of Aβ43 to Aβ40 by the successive action of angiotensin-converting enzyme 2 and angiotensin-converting enzyme. J Neurosci Res 2014; 92(9): 1178–1186, https://doi.org/10.1002/jnr.23404.
- Liu S., Ando F., Fujita Y., Liu J., Maeda T., Shen X., Kikuchi K., Matsumoto A., Yokomori M., Tanabe-Fujimura C., Shimokata H., Michikawa M., Komano H., Zou K. A clinical dose of angiotensin-converting enzyme (ACE) inhibitor and heterozygous ACE deletion exacerbate Alzheimer’s disease pathology in mice. J Biol Chem 2019; 294(25): 9760–9770, https://doi.org/10.1074/jbc.ra118.006420.
- Chappell M.C., Marshall A.C., Alzayadneh E.M., Shaltout H.A., Diz D.I. Update on the angiotensin converting enzyme 2-angiotensin (1-7)-Mas receptor axis: fetal programing, sex differences, and intracellular pathways. Front Endocrinol (Lausanne) 2014; 4: 201, https://doi.org/10.3389/fendo.2013.00201.
- Wilson B.A., Nautiyal M., Gwathmey T.M., Rose J.C., Chappell M.C. Evidence for a mitochondrial angiotensin-(1–7) system in the kidney. Am J Physiol Renal Physiol 2016; 310(7): 637–645, https://doi.org/10.1152/ajprenal.00479.2015.
- Yu C., Tang W., Wang Y., Shen Q., Wang B., Cai C., Meng X., Zou F. Downregulation of ACE2/Ang-(1–7)/Mas axis promotes breast cancer metastasis by enhancing store-operated calcium entry. Cancer Lett 2016; 376(2): 268–277, https://doi.org/10.1016/j.canlet.2016.04.006.
- Li Z., Mo N., Li L., Cao Y., Wang W., Liang Y., Deng H., Xing R., Yang L., Ni C., Chui D., Guo X. Surgery-induced hippocampal angiotensin II elevation causes blood-brain barrier disruption via MMP/TIMP in aged rats. Front Cell Neurosci 2016; 10: 105, https://doi.org/10.3389/fncel.2016.00105.
- Xu J., Sriramula S., Lazartigues E. Excessive glutamate stimulation impairs ACE2 activity through adam17-mediated shedding in cultured cortical neurons. Cell Mol Neurobiol 2018; 38(6): 1235–1243, https://doi.org/10.1007/s10571-018-0591-8.
- Mo J., Enkhjargal B., Travis Z.D., Zhou K., Wu P., Zhang G., Zhu Q., Zhang T., Peng J., Xu W., Ocak U., Chen Y., Tang J., Zhang J., Zhang J.H. AVE 0991 attenuates oxidative stress and neuronal apoptosis via Mas/PKA/CREB/UCP-2 pathway after subarachnoid hemorrhage in rats. Redox Biol 2019; 20: 75–86, https://doi.org/10.1016/j.redox.2018.09.022.
- Cao X., Lu X.M., Tuo X., Liu J.Y., Zhang Y.C., Song L.N., Cheng Z.Q., Yang J.K., Xin Z. Angiotensin-converting enzyme 2 regulates endoplasmic reticulum stress and mitochondrial function to preserve skeletal muscle lipid metabolism. Lipids Health Dis 2019; 18(1): 207, https://doi.org/10.1186/s12944-019-1145-x.
- Arroja M.M.C., Reid E., Roy L.A., Vallatos A.V., Holmes W.M., Nicklin S.A., Work L.M., McCabe C. Assessing the effects of Ang-(1–7) therapy following transient middle cerebral artery occlusion. Sci Rep 2019; 9(1): 3154, https://doi.org/10.1038/s41598-019-39102-8.
- Grass G.D., Toole B.P. How, with whom and when: an overview of CD147-mediated regulatory networks influencing matrix metalloproteinase activity. Biosci Rep 2015; 36(1): e00283, https://doi.org/10.1042/bsr20150256.
- Успенская Ю.А., Моргун А.В., Осипова Е.Д., Семячкина-Глушковская О.В., Малиновская Н.А. CD147 как новая молекула-мишень для фармакотерапии в онкологии. Экспериментальная и клиническая фармакология 2019; 82(3): 36–44, https://doi.org/10.30906/0869-2092-2019-82-3-36-44.
- Kaushik D.K., Hahn J.N., Yong V.W. EMMPRIN, an upstream regulator of MMPs, in CNS biology. Matrix Biol 2015; 44–46: 138–146, https://doi.org/10.1016/j.matbio.2015.01.018.
- Agrawal S.M., Silva C., Tourtellotte W.W., Yong V.W. EMMPRIN: a novel regulator of leukocyte transmigration into the CNS in multiple sclerosis and experimental autoimmune encephalomyelitis. J Neurosci 2011; 31(2): 669–677, https://doi.org/10.1523/jneurosci.3659-10.2011.
- Kanyenda L.J., Verdile G., Boulos S., Krishnaswamy S., Taddei K., Meloni B.P., Mastaglia F.L., Martins R.N. The dynamics of CD147 in Alzheimer’s disease development and pathology. J. Alzheimers Dis 2011; 26(4): 593–605, https://doi.org/10.3233/jad-2011-110584.
- Nahalkova J., Volkmann I., Aoki M., Winblad B., Bogdanovic N., Tjernberg L.O., Behbahani H. CD147, a γ-secretase associated protein is upregulated in Alzheimer’s disease brain and its cellular trafficking is affected by presenilin-2. Neurochem Int 2010; 56(1): 67–76, https://doi.org/10.1016/j.neuint.2009.09.003.
- Zhou S., Zhou H., Walian P.J., Jap B.K. Regulation of γ-secretase activity in Alzheimer’s disease. Biochemistry 2007; 46(10): 2553–2563, https://doi.org/10.1021/bi602509c.
- Zhou S., Zhou H., Walian P.J., Jap B.K. CD147 is a regulatory subunit of the gamma-secretase complex in Alzheimer’s disease amyloid β-peptide production. Proc Natl Acad Sci U S A 2005; 102(21): 7499–7504, https://doi.org/10.1073/pnas.0502768102.
- Satoh J., Tabunoki H., Ishida T., Saito Y., Arima K. Immunohistochemical characterization of γ-secretase activating protein expression in Alzheimer’s disease brains. Neuropathol Appl Neurobiol 2012; 38(2): 132–141, https://doi.org/10.1111/j.1365-2990.2011.01206.x.
- Xie J., Li X., Zhou Y., Wu J., Tan Y., Ma X., Zhao Y., Liu X., Zhao Y. Resveratrol abrogates hypoxia-induced up-regulation of exosomal amyloid-β partially by inhibiting CD147. Neurochem Res 2019; 44(5): 1113–1126, https://doi.org/10.1007/s11064-019-02742-3.
- Muri L., Leppert D., Grandgirard D., Leib S.L. MMPs and ADAMs in neurological infectious diseases and multiple sclerosis. Cell Mol Life Sci 2019; 76(16): 3097–3116, https://doi.org/10.1007/s00018-019-03174-6.
- Rosenberg G.A., Estrada E.Y., Mobashery S. Effect of synthetic matrix metalloproteinase inhibitors on lipopolysaccharide-induced blood–brain barrier opening in rodents: differences in response based on strains and solvents. Brain Research 2007; 1133(1): 186–192, https://doi.org/10.1016/j.brainres.2006.11.041.
- Salmina A.B., Kuvacheva N.V., Morgun A.V., Komleva Yu.K., Pozhilenkova E.A., Lopatina O.L., Gorina Y.V., Taranushenko T.E., Petrova L.L. Glycolysis-mediated control of blood-brain barrier development and function. Int J Biochem Cell Biol 2015; 64: 174–184, https://doi.org/10.1016/j.biocel.2015.04.005.
- Curtin K.D., Meinertzhagen I.A., Wyman R.J. Basigin (EMMPRIN/CD147) interacts with integrin to affect cellular architecture. J Cell Sci 2005; 118(Pt 12): 2649–2660, https://doi.org/10.1242/jcs.02408.
- Higashida H., Salmina A.B., Olovyannikova R.Y., Hashii M., Yokoyama S., Koizumi K., Jin D., Liu H.X., Lopatina O., Amina S., Islam M.S., Huang J.J., Noda M. Cyclic ADP-ribose as a universal calcium signal molecule in the nervous system. Neurochem Int 2007; 51(2–4): 192–199, https://doi.org/10.1016/j.neuint.2007.06.023.
- Buckley C., Wilson C., McCarron J.G. FK506 regulates Ca2+ release evoked by inositol 1,4,5-trisphosphate independently of FK-binding protein in endothelial cells. Br J Pharmacol 2020; 177(5): 1131–1149, https://doi.org/10.1111/bph.14905.
- Liu S., Jin R., Xiao A.Y., Zhong W., Li G. Inhibition of CD147 improves oligodendrogenesis and promotes white matter integrity and functional recovery in mice after ischemic stroke. Brain Behav Immun 2019; 82: 13–24, https://doi.org/10.1016/j.bbi.2019.07.027.
- Kim M.S., Lee G.H., Kim Y.M., Lee B.W., Nam H.Y., Sim U.C., Choo S.J., Yu S.W., Kim J.J., Kim Kwon Y., Who Kim S. Angiotensin II causes apoptosis of adult hippocampal neural stem cells and memory impairment through the action on AMPK-PGC1α signaling in heart failure. Stem Cells Transl Med 2017; 6(6): 1491–1503, https://doi.org/10.1002/sctm.16-0382.
- Jin Z.G., Melaragno M.G., Liao D.F., Yan C., Haendeler J., Suh Y.A., Lambeth J.D., Berk B.C. Cyclophilin A is a secreted growth factor induced by oxidative stress. Circ Res 2000; 87(9): 789–796, https://doi.org/10.1161/01.res.87.9.789.
- Bell R.D., Winkler E.A., Singh I., Sagare A.P., Deane R., Wu Z., Holtzman D.M., Betsholtz C., Armulik A., Sallstrom J., Berk B.C., Zlokovic B.V. Apolipoprotein E controls cerebrovascular integrity via cyclophilin A. Nature 2012; 485(7399): 512–516, https://doi.org/10.1038/nature11087.
- Halliday M.R., Rege S.V., Ma Q., Zhao Z., Miller C.A., Winkler E.A., Zlokovic B.V. Accelerated pericyte degeneration and blood-brain barrier breakdown in apolipoprotein E4 carriers with Alzheimer’s disease. J Cereb Blood Flow Metab 2016; 36(1): 216–227, https://doi.org/10.1038/jcbfm.2015.44.
- Halliday M.R., Pomara N., Sagare A.P., Mack W.J., Frangione B., Zlokovic B.V. Relationship between cyclophilin a levels and matrix metalloproteinase 9 activity in cerebrospinal fluid of cognitively normal apolipoprotein e4 carriers and blood-brain barrier breakdown. JAMA Neurol 2013; 70(9): 1198–1200, https://doi.org/10.1001/jamaneurol.2013.3841.
- Tohidpour A., Morgun A.V., Boitsova E.B., Malinovskaya N.A., Martynova G.P., Khilazheva E.D., Kopylevich N.V., Gertsog G.E., Salmina A.B. Neuroinflammation and infection: molecular mechanisms associated with dysfunction of neurovascular unit. Front Cell Infect Microbiol 2017; 7: 276, https://doi.org/10.3389/fcimb.2017.00276.