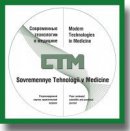
РНК-секвенирование и клеточные модели вирус-ассоциированного рака (обзор)
Обобщены результаты исследований, опирающихся на применение технологий транскриптомного анализа к современным клеточным модельным системам рака, ассоциированного с вирусом папилломы человека — ВПЧ (рака шейки матки, опухолей головы и шеи). Рассматривается многообразие трехмерных моделей рака, таких как сфероиды, органоиды (органотипические культуры), экспланты, мышиные ксенографты. Особое внимание уделено использованию биоматериала пациентов для получения временных культур первичных опухолевых клеток, а также созданию многокомпонентных (гетероклеточных) систем, включающих наравне с опухолевым компонентом элементы его микроокружения. Обсуждается ряд уникальных биологических свойств ВПЧ-индуцированных опухолей, которые делают получение клеточных моделей весьма незаурядной задачей.
Для каждого варианта модельных систем приведены новейшие обнаружения в области молекулярных механизмов возникновения и прогрессии ВПЧ-зависимого рака, сделанные с помощью РНК-секвенирования. Эти обнаружения рассматриваются в прикладном аспекте — с точки зрения открывающихся возможностей для доклинического тестирования новых препаратов, персонализированной диагностики и подбора индивидуальных, наиболее эффективных схем лечения. Рассмотрены вопросы формирования лекарственной устойчивости, молекулярно-клеточной гетерогенности, эпигенетического перепрограммирования, роли стромального микроокружения. Акцентируются проблемы, связанные с ограничениями применимости той или иной модельной системы. Отмечаются направления, по которым наблюдается существенное отставание омиксных исследований по вирус-ассоциированному раку в сравнении с другими типами онкопатологий, и возможные причины этого отставания. Обозначены также перспективы будущего развития модельных систем ВПЧ-ассоциированных опухолей в области высокотехнологичной тканевой инженерии, в частности применение биопечати и микрофлюидных биочипов. Сочетание данных технологий с методами полногеномного профилирования позволит существенно увеличить трансляционный потенциал описываемых модельных клеточных систем.
- Lõhmussaar K., Boretto M., Clevers H. Human-derived model systems in gynecological cancer research. Trends Cancer 2020; 6(12): 1031–1043, https://doi.org/10.1016/j.trecan.2020.07.007.
- Demers I., Donkers J., Kremer B., Speel E.J. Ex vivo culture models to indicate therapy response in head and neck squamous cell carcinoma. Cells 2020; 9(11): 2527, https://doi.org/10.3390/cells9112527.
- Méry B., Rancoule C., Guy J.B., Espenel S., Wozny A.S., Battiston-Montagne P., Ardail D., Beuve M., Alphonse G., Rodriguez-Lafrasse C., Magné N. Preclinical models in HNSCC: a comprehensive review. Oral Oncol 2017; 65: 51–56, https://doi.org/10.1016/j.oraloncology.2016.12.010.
- Shan F., Close D.A., Camarco D.P., Johnston P.A. High-content screening comparison of cancer drug accumulation and distribution in two-dimensional and three-dimensional culture models of head and neck cancer. Assay Drug Dev Technol 2018; 16(1): 27–50, https://doi.org/10.1089/adt.2017.812.
- Melissaridou S., Wiechec E., Magan M., Jain M.V., Chung M.K., Farnebo L., Roberg K. The effect of 2D and 3D cell cultures on treatment response, EMT profile and stem cell features in head and neck cancer. Cancer Cell Int 2019; 19: 16, https://doi.org/10.1186/s12935-019-0733-1.
- Ayuso J.M., Vitek R., Swick A.D., Skala M.C., Wisinski K.B., Kimple R.J., Lambert P.F., Beebe D.J. Effects of culture method on response to EGFR therapy in head and neck squamous cell carcinoma cells. Sci Rep 2019; 9(1): 12480, https://doi.org/10.1038/s41598-019-48764-3.
- Semertzidou A., Brosens J.J., McNeish I., Kyrgiou M. Organoid models in gynaecological oncology research. Cancer Treat Rev 2020; 90: 102103, https://doi.org/10.1016/j.ctrv.2020.102103.
- Doorbar J. Model systems of human papillomavirus-associated disease. J Pathol 2016; 238(2): 166–179, https://doi.org/10.1002/path.4656.
- Facompre N.D., Rajagopalan P., Sahu V., Pearson A.T., Montone K.T., James C.D., Gleber-Netto F.O., Weinstein G.S., Jalaly J., Lin A., Rustgi A.K., Nakagawa H., Califano J.A., Pickering C.R., White E.A., Windle B.E., Morgan I.M., Cohen R.B., Gimotty P.A., Basu D. Identifying predictors of HPV-related head and neck squamous cell carcinoma progression and survival through patient-derived models. Int J Cancer 2020; 147(11): 3236–3249, https://doi.org/10.1002/ijc.33125.
- Hayes T.F., Benaich N., Goldie S.J., Sipilä K., Ames-Draycott A., Cai W., Yin G., Watt F.M. Integrative genomic and functional analysis of human oral squamous cell carcinoma cell lines reveals synergistic effects of FAT1 and CASP8 inactivation. Cancer Lett 2016; 383(1): 106–114, https://doi.org/10.1016/j.canlet.2016.09.014.
- Pirotte E.F., Holzhauser S., Owens D., Quine S., Al-Hussaini A., Christian A.D., Giles P.J., Man S.T., Evans M., Powell N.G. Sensitivity to inhibition of DNA repair by Olaparib in novel oropharyngeal cancer cell lines infected with human papillomavirus. PLoS One 2018; 13(12): e0207934, https://doi.org/10.1371/journal.pone.0207934.
- Deng H., Mondal S., Sur S., Woodworth C.D. Establishment and optimization of epithelial cell cultures from human ectocervix, transformation zone, and endocervix optimization of epithelial cell cultures. J Cell Physiol 2019; 234(6): 7683–7694, https://doi.org/10.1002/jcp.28049.
- Zhou Y., Zhang Q., Gao G., Zhang X., Liu Y., Yuan S., Wang X., Chen J.J. Role of WDHD1 in human papillomavirus-mediated oncogenesis identified by transcriptional profiling of E7-expressing cells. J Virol 2016; 90(13): 6071–6084, https://doi.org/10.1128/jvi.00513-16.
- Klymenko T., Gu Q., Herbert I., Stevenson A., Iliev V., Watkins G., Pollock C., Bhatia R., Cuschieri K., Herzyk P., Gatherer D., Graham S.V. RNA-Seq analysis of differentiated keratinocytes reveals a massive response to late events during human papillomavirus 16 infection, including loss of epithelial barrier function. J Virol 2017; 91(24): e01001-17, https://doi.org/10.1128/jvi.01001-17.
- Hua C., Zhu J., Zhang B., Sun S., Song Y., van der Veen S., Cheng H. Digital RNA sequencing of human epidermal keratinocytes carrying human papillomavirus type 16 E7. Front Genet 2020; 11: 819, https://doi.org/10.3389/fgene.2020.00819.
- Xu J., Fang Y., Qin J., Chen X., Liang X., Xie X., Lu W. A transcriptomic landscape of human papillomavirus 16 E6-regulated gene expression and splicing events. FEBS Lett 2016; 590(24): 4594–4605, https://doi.org/10.1002/1873-3468.12486.
- Evans M.R., James C.D., Bristol M.L., Nulton T.J., Wang X., Kaur N., White E.A., Windle B., Morgan I.M. Human papillomavirus 16 E2 regulates keratinocyte gene expression relevant to cancer and the viral life cycle. J Virol 2019; 93(4): e01941-18, https://doi.org/10.1128/jvi.01941-18.
- Barr J.A., Hayes K.E., Brownmiller T., Harold A.D., Jagannathan R., Lockman P.R., Khan S., Martinez I. Long non-coding RNA FAM83H-AS1 is regulated by human papillomavirus 16 E6 independently of p53 in cervical cancer cells. Sci Rep 2019; 9(1): 3662, https://doi.org/10.1038/s41598-019-40094-8.
- Habiger C., Jäger G., Walter M., Iftner T., Stubenrauch F. Interferon kappa inhibits human papillomavirus 31 transcription by inducing SP100 proteins. J Virol 2015; 90(2): 694–704, https://doi.org/10.1128/jvi.02137-15.
- Tamura R., Yoshihara K., Saito T., Ishimura R., Martínez-Ledesma J.E., Xin H., Ishiguro T., Mori Y., Yamawaki K., Suda K., Sato S., Itamochi H., Motoyama T., Aoki Y., Okuda S., Casingal C.R., Nakaoka H., Inoue I., Verhaak R.G.W., Komatsu M., Enomoto T. Novel therapeutic strategy for cervical cancer harboring FGFR3-TACC3 fusions. Oncogenesis 2018; 7(1): 4, https://doi.org/10.1038/s41389-017-0018-2.
- Samuels T.L., Zimmermann M.T., Zeighami A., Demos W., Southwood J.E., Blumin J.H., Bock J.M., Johnston N. RNA sequencing reveals cancer-associated changes in laryngeal cells exposed to non-acid pepsin. Laryngoscope 2021; 131(1): 121–129, https://doi.org/10.1002/lary.28636.
- Rosa M.N., Evangelista A.F., Leal L.F., De Oliveira C.M., Silva V.A.O., Munari C.C., Munari F.F., Matsushita G.M., Dos Reis R., Andrade C.E., Souza C.P., Reis R.M. Establishment, molecular and biological characterization of HCB-514: a novel human cervical cancer cell line. Sci Rep 2019; 9(1): 1913, https://doi.org/10.1038/s41598-018-38315-7.
- Fadlullah M.Z., Chiang I.K., Dionne K.R., Yee P.S., Gan C.P., Sam K.K., Tiong K.H., Ng A.K., Martin D., Lim K.P., Kallarakkal T.G., Mustafa W.M., Lau S.H., Abraham M.T., Zain R.B., Rahman Z.A., Molinolo A., Patel V., Gutkind J.S., Tan A.C., Cheong S.C. Genetically-defined novel oral squamous cell carcinoma cell lines for the development of molecular therapies. Oncotarget 2016; 7(19): 27802–27818, https://doi.org/10.18632/oncotarget.8533.
- Zammataro L., Lopez S., Bellone S., Pettinella F., Bonazzoli E., Perrone E., Zhao S., Menderes G., Altwerger G., Han C., Zeybek B., Bianchi A., Manzano A., Manara P., Cocco E., Buza N., Hui P., Wong S., Ravaggi A., Bignotti E., Romani C., Todeschini P., Zanotti L., Odicino F., Pecorelli S., Donzelli C., Ardighieri L., Angioli R., Raspagliesi F., Scambia G., Choi J., Dong W., Bilguvar K., Alexandrov L.B., Silasi D.A., Huang G.S., Ratner E., Azodi M., Schwartz P.E., Pirazzoli V., Stiegler A.L., Boggon T.J., Lifton R.P., Schlessinger J., Santin A.D. Whole-exome sequencing of cervical carcinomas identifies activating ERBB2 and PIK3CA mutations as targets for combination therapy. Proc Natl Acad Sci U S A 2019; 116(45): 22730–22736, https://doi.org/10.1073/pnas.1911385116.
- Wang Y., Liu L., Chen Z. Transcriptome profiling of cervical cancer cells acquired resistance to cisplatin by deep sequencing. Artif Cells Nanomed Biotechnol 2019; 47(1): 2820–2829, https://doi.org/10.1080/21691401.2019.1637882.
- Lu X., Wang Z., Huang H., Wang H. Hedgehog signaling promotes multidrug resistance by regulation of ABC transporters in oral squamous cell carcinoma. J Oral Pathol Med 2020; 49(9): 897–906, https://doi.org/10.1111/jop.13050.
- Stein-O’Brien G., Kagohara L.T., Li S., Thakar M., Ranaweera R., Ozawa H., Cheng H., Considine M., Schmitz S., Favorov A.V., Danilova L.V., Califano J.A., Izumchenko E., Gaykalova D.A., Chung C.H., Fertig E.J. Integrated time course omics analysis distinguishes immediate therapeutic response from acquired resistance. Genome Med 2018; 10(1): 37, https://doi.org/10.1186/s13073-018-0545-2.
- Kagohara L.T., Zamuner F., Davis-Marcisak E.F., Sharma G., Considine M., Allen J., Yegnasubramanian S., Gaykalova D.A., Fertig E.J. Integrated single-cell and bulk gene expression and ATAC-seq reveals heterogeneity and early changes in pathways associated with resistance to cetuximab in HNSCC-sensitive cell lines. Br J Cancer 2020; 123(1): 101–113, https://doi.org/10.1038/s41416-020-0851-5.
- Lupu-Plesu M., Claren A., Martial S., N’Diaye P.D., Lebrigand K., Pons N., Ambrosetti D., Peyrottes I., Feuillade J., Hérault J., Dufies M., Doyen J., Pagès G. Effects of proton versus photon irradiation on (lymph)angiogenic, inflammatory, proliferative and anti-tumor immune responses in head and neck squamous cell carcinoma. Oncogenesis 2017; 6(7): e354, https://doi.org/10.1038/oncsis.2017.56.
- Guo C., Xu L.F., Li H.M., Wang W., Guo J.H., Jia M.Q., Jia R., Jia J. Transcriptomic study of the mechanism of anoikis resistance in head and neck squamous carcinoma. Peer J 2019; 7: e6978, https://doi.org/10.7717/peerj.6978.
- Low J.L., Lau D.P., Zhang X., Kwang X.L., Rohatgi N., Chan J.V., Chong F.T., Wong S.Q.R., Leong H.S., Thangavelu M.T., Rikka S., Skanderup A.M.J., Tan D.S.W., Periyasamy G., Koh J.L.Y., Iyer N.G., DasGupta R. A chemical genetic screen identifies Aurora kinases as a therapeutic target in EGFR T790M negative, gefitinib-resistant head and neck squamous cell carcinoma (HNSCC). EBioMedicine 2021; 64: 103220, https://doi.org/10.1016/j.ebiom.2021.103220.
- Sharma A., Cao E.Y., Kumar V., Zhang X., Leong H.S., Wong A.M.L., Ramakrishnan N., Hakimullah M., Teo H.M.V., Chong F.T., Chia S., Thangavelu M.T., Kwang X.L., Gupta R., Clark J.R., Periyasamy G., Iyer N.G., DasGupta R. Longitudinal single-cell RNA sequencing of patient-derived primary cells reveals drug-induced infidelity in stem cell hierarchy. Nat Commun 2018; 9(1): 4931, https://doi.org/10.1038/s41467-018-07261-3.
- Voutsadakis I.A. PI3KCA mutations in uterine cervix carcinoma. J Clin Med 2021; 10(2): 220, https://doi.org/10.3390/jcm10020220.
- Xu C., Nikolova O., Basom R.S., Mitchell R.M., Shaw R., Moser R.D., Park H., Gurley K.E., Kao M.C., Green C.L., Schaub F.X., Diaz R.L., Swan H.A., Jang I.S., Guinney J., Gadi V.K., Margolin A.A., Grandori C., Kemp C.J., Méndez E. Functional precision medicine identifies novel druggable targets and therapeutic options in head and neck cancer. Clin Cancer Res 2018; 24(12): 2828–2843, https://doi.org/10.1158/1078-0432.ccr-17-1339.
- Sa J.K., Hwang J.R., Cho Y.J., Ryu J.Y., Choi J.J., Jeong S.Y., Kim J., Kim M.S., Paik E.S., Lee Y.Y., Choi C.H., Kim T.J., Kim B.G., Bae D.S., Lee Y., Her N.G., Shin Y.J., Cho H.J., Kim J.Y., Seo Y.J., Koo H., Oh J.W., Lee T., Kim H.S., Song S.Y., Bae J.S., Park W.Y., Han H.D., Ahn H.J., Sood A.K., Rabadan R., Lee J.K., Nam D.H., Lee J.W. Pharmacogenomic analysis of patient-derived tumor cells in gynecologic cancers. Genome Biol 2019; 20(1): 253, https://doi.org/10.1186/s13059-019-1848-3.
- Kumar M.M., Davuluri S., Poojar S., Mukherjee G., Bajpai A.K., Bafna U.D., Devi U.K., Kallur P.P., Kshitish A.K., Jayshree R.S. Role of estrogen receptor alpha in human cervical cancer-associated fibroblasts: a transcriptomic study. Tumour Biol 2016; 37(4): 4409–4420, https://doi.org/10.1007/s13277-015-4257-6.
- Patel A.K., Vipparthi K., Thatikonda V., Arun I., Bhattacharjee S., Sharan R., Arun P., Singh S. A subtype of cancer-associated fibroblasts with lower expression of alpha-smooth muscle actin suppresses stemness through BMP4 in oral carcinoma. Oncogenesis 2018; 7(10): 78, https://doi.org/10.1038/s41389-018-0087-x.
- Takahashi H., Rokudai S., Kawabata-Iwakawa R., Sakakura K., Oyama T., Nishiyama M., Chikamatsu K. AKT3 is a novel regulator of cancer-associated fibroblasts in head and neck squamous cell carcinoma. Cancers (Basel) 2021; 13(6): 1233, https://doi.org/10.3390/cancers13061233.
- Ding L., Ren J., Zhang D., Li Y., Huang X., Hu Q., Wang H., Song Y., Ni Y., Hou Y. A novel stromal lncRNA signature reprograms fibroblasts to promote the growth of oral squamous cell carcinoma via LncRNA-CAF/interleukin-33. Carcinogenesis 2018; 39(3): 397–406, https://doi.org/10.1093/carcin/bgy006.
- Lopatina T., Favaro E., Danilova L., Fertig E.J., Favorov A.V., Kagohara L.T., Martone T., Bussolati B., Romagnoli R., Albera R., Pecorari G., Brizzi M.F., Camussi G., Gaykalova D.A. Extracellular vesicles released by tumor endothelial cells spread immunosuppressive and transforming signals through various recipient cells. Front Cell Dev Biol 2020; 8: 698, https://doi.org/10.3389/fcell.2020.00698.
- Mattoscio D., Isopi E., Lamolinara A., Patruno S., Medda A., De Cecco F., Chiocca S., Iezzi M., Romano M., Recchiuti A. Resolvin D1 reduces cancer growth stimulating a protective neutrophil-dependent recruitment of anti-tumor monocytes. J Exp Clin Cancer Res 2021; 40(1): 129, https://doi.org/10.1186/s13046-021-01937-3.
- Arayataweegool A., Srisuttee R., Bin-Alee F., Mahattanasakul P., Tangjaturonrasme N., Kerekhanjanarong V., Mutirangura A., Kitkumthorn N. Induction of ZCCHC6 expression in peripheral blood mononuclear cells by HNSCC secretions. Gene 2020; 754: 144880, https://doi.org/10.1016/j.gene.2020.144880.
- Liu C., Billet S., Choudhury D., Cheng R., Haldar S., Fernandez A., Biondi S., Liu Z., Zhou H., Bhowmick N.A. Bone marrow mesenchymal stem cells interact with head and neck squamous cell carcinoma cells to promote cancer progression and drug resistance. Neoplasia 2021; 23(1): 118–128, https://doi.org/10.1016/j.neo.2020.11.012.
- Castro-Oropeza R., Vazquez-Santillan K., Díaz-Gastelum C., Melendez-Zajgla J., Zampedri C., Ferat-Osorio E., Rodríguez-González A., Arriaga-Pizano L., Maldonado V. Adipose-derived mesenchymal stem cells promote the malignant phenotype of cervical cancer. Sci Rep 2020; 10(1): 14205, https://doi.org/10.1038/s41598-020-69907-x.
- Jackson R., Maarsingh J.D., Herbst-Kralovetz M.M., Van Doorslaer K. 3D oral and cervical tissue models for studying papillomavirus host-pathogen interactions. Curr Protoc Microbiol 2020; 59(1): e129, https://doi.org/10.1002/cpmc.129.
- De Gregorio V., La Rocca A., Urciuolo F., Annunziata C., Tornesello M.L., Buonaguro F.M., Netti P.A., Imparato G. Modeling the epithelial-mesenchymal transition process in a 3D organotypic cervical neoplasia. Acta Biomater 2020; 116: 209–222, https://doi.org/10.1016/j.actbio.2020.09.006.
- Santi M., Mapanao A.K., Cappello V., Voliani V. Production of 3D tumor models of head and neck squamous cell carcinomas for nanotheranostics assessment. ACS Biomater Sci Eng 2020; 6(9): 4862–4869, https://doi.org/10.1021/acsbiomaterials.0c00617.
- Yang S., Chen T., Huang L., Xu S., Cao Z., Zhang S., Xu J., Li Y., Yue Y., Lu W., Cheng X., Xie X. High-risk human papillomavirus E7 maintains stemness via APH1B in cervical cancer stem-cell like cells. Cancer Manag Res 2019; 11: 9541–9552, https://doi.org/10.2147/cmar.s194239.
- Thippabhotla S., Zhong C., He M. 3D cell culture stimulates the secretion of in vivo like extracellular vesicles. Sci Rep 2019; 9(1): 13012, https://doi.org/10.1038/s41598-019-49671-3.
- Tyagi A., Vishnoi K., Mahata S., Verma G., Srivastava Y., Masaldan S., Roy B.G., Bharti A.C., Das B.C. Cervical cancer stem cells selectively overexpress HPV oncoprotein E6 that controls stemness and self-renewal through upregulation of HES1. Clin Cancer Res 2016; 22(16): 4170–4184, https://doi.org/10.1158/1078-0432.ccr-15-2574.
- Kaseb H.O., Fohrer-Ting H., Lewis D.W., Lagasse E., Gollin S.M. Identification, expansion and characterization of cancer cells with stem cell properties from head and neck squamous cell carcinomas. Exp Cell Res 2016; 348(1): 75–86, https://doi.org/10.1016/j.yexcr.2016.09.003.
- Azharuddin M., Roberg K., Dhara A.K., Jain M.V., Darcy P., Hinkula J., Slater N.K.H., Patra H.K. Dissecting multi drug resistance in head and neck cancer cells using multicellular tumor spheroids. Sci Rep 2019; 9(1): 20066, https://doi.org/10.1038/s41598-019-56273-6.
- Hagemann J., Jacobi C., Gstoettner S., Welz C., Schwenk-Zieger S., Stauber R., Strieth S., Kuenzel J., Baumeister P., Becker S. Therapy testing in a spheroid-based 3D cell culture model for head and neck squamous cell carcinoma. J Vis Exp 2018; 134: 57012, https://doi.org/10.3791/57012.
- Chumduri C., Gurumurthy R.K., Berger H., Dietrich O., Kumar N., Koster S., Brinkmann V., Hoffmann K., Drabkina M., Arampatzi P., Son D., Klemm U., Mollenkopf H.J., Herbst H., Mangler M., Vogel J., Saliba A.E., Meyer T.F. Opposing Wnt signals regulate cervical squamocolumnar homeostasis and emergence of metaplasia. Nat Cell Biol 2021; 23(2): 184–197, https://doi.org/10.1038/s41556-020-00619-0.
- Lõhmussaar K., Oka R., Espejo Valle-Inclan J., Smits M.H.H., Wardak H., Korving J., Begthel H., Proost N., van de Ven M., Kranenburg O.W., Jonges T.G.N., Zweemer R.P., Veersema S., van Boxtel R., Clevers H. Patient-derived organoids model cervical tissue dynamics and viral oncogenesis in cervical cancer. Cell Stem Cell 2021; 28(8): 1380–1396.e6, https://doi.org/10.1016/j.stem.2021.03.012.
- Maru Y., Kawata A., Taguchi A., Ishii Y., Baba S., Mori M., Nagamatsu T., Oda K., Kukimoto I., Osuga Y., Fujii T., Hippo Y. Establishment and molecular phenotyping of organoids from the squamocolumnar junction region of the uterine cervix. Cancers (Basel) 2020; 12(3): 694, https://doi.org/10.3390/cancers12030694.
- Maru Y., Tanaka N., Ebisawa K., Odaka A., Sugiyama T., Itami M., Hippo Y. Establishment and characterization of patient-derived organoids from a young patient with cervical clear cell carcinoma. Cancer Sci 2019; 110(9): 2992–3005, https://doi.org/10.1111/cas.14119.
- Hill S.J., D’Andrea A.D. Predictive potential of head and neck squamous cell carcinoma organoids. Cancer Discov 2019; 9(7): 828–830, https://doi.org/10.1158/2159-8290.cd-19-0527.
- Chitturi Suryaprakash R.T., Kujan O., Shearston K., Farah C.S. Three-dimensional cell culture models to investigate oral carcinogenesis: a scoping review. Int J Mol Sci 2020; 21(24): 9520, https://doi.org/10.3390/ijms21249520.
- Karakasheva T.A., Kijima T., Shimonosono M., Maekawa H., Sahu V., Gabre J.T., Cruz-Acuña R., Giroux V., Sangwan V., Whelan K.A., Natsugoe S., Yoon A.J., Philipone E., Klein-Szanto A.J., Ginsberg G.G., Falk G.W., Abrams J.A., Que J., Basu D., Ferri L., Diehl J.A., Bass A.J., Wang T.C., Rustgi A.K., Nakagawa H. Generation and characterization of patient-derived head and neck, oral, and esophageal cancer organoids. Curr Protoc Stem Cell Biol 2020; 53(1): e109, https://doi.org/10.1002/cpsc.109.
- Driehuis E., Spelier S., Beltrán Hernández I., de Bree R., Willems S.M., Clevers H., Oliveira S. Patient-derived head and neck cancer organoids recapitulate EGFR expression levels of respective tissues and are responsive to EGFR-targeted photodynamic therapy. J Clin Med 2019; 8(11): 1880, https://doi.org/10.3390/jcm8111880.
- Driehuis E., Kretzschmar K., Clevers H. Establishment of patient-derived cancer organoids for drug-screening applications. Nat Protoc 2020; 15(10): 3380–3409, https://doi.org/10.1038/s41596-020-0379-4.
- Kijima T., Nakagawa H., Shimonosono M., Chandramouleeswaran P.M., Hara T., Sahu V., Kasagi Y., Kikuchi O., Tanaka K., Giroux V., Muir A.B., Whelan K.A., Ohashi S., Naganuma S., Klein-Szanto A.J., Shinden Y., Sasaki K., Omoto I., Kita Y., Muto M., Bass A.J., Diehl J.A., Ginsberg G.G., Doki Y., Mori M., Uchikado Y., Arigami T., Avadhani N.G., Basu D., Rustgi A.K., Natsugoe S. Three-dimensional organoids reveal therapy resistance of esophageal and oropharyngeal squamous cell carcinoma cells. Cell Mol Gastroenterol Hepatol 2018; 7(1): 73–91, https://doi.org/10.1016/j.jcmgh.2018.09.003.
- Tanaka N., Osman A.A., Takahashi Y., Lindemann A., Patel A.A., Zhao M., Takahashi H., Myers J.N. Head and neck cancer organoids established by modification of the CTOS method can be used to predict in vivo drug sensitivity. Oral Oncol 2018; 87: 49–57, https://doi.org/10.1016/j.oraloncology.2018.10.018.
- Driehuis E., Kolders S., Spelier S., Lõhmussaar K., Willems S.M., Devriese L.A., de Bree R., de Ruiter E.J., Korving J., Begthel H., van Es J.H., Geurts V., He G.W., van Jaarsveld R.H., Oka R., Muraro M.J., Vivié J., Zandvliet M.M.J.M., Hendrickx A.P.A., Iakobachvili N., Sridevi P., Kranenburg O., van Boxtel R., Kops G.J.P.L., Tuveson D.A., Peters P.J., van Oudenaarden A., Clevers H. Oral mucosal organoids as a potential platform for personalized cancer therapy. Cancer Discov 2019; 9(7): 852–871, https://doi.org/10.1158/2159-8290.cd-18-1522.
- De Gregorio V., Urciuolo F., Netti P.A., Imparato G. In vitro organotypic systems to model tumor microenvironment in human papillomavirus (HPV)-related cancers. Cancers (Basel) 2020; 12(5): 1150, https://doi.org/10.3390/cancers12051150.
- Evans M.R., James C.D., Loughran O., Nulton T.J., Wang X., Bristol M.L., Windle B., Morgan I.M. An oral keratinocyte life cycle model identifies novel host genome regulation by human papillomavirus 16 relevant to HPV positive head and neck cancer. Oncotarget 2017; 8(47): 81892–81909, https://doi.org/10.18632/oncotarget.18328.
- Bienkowska-Haba M., Luszczek W., Zwolinska K., Scott R.S., Sapp M. Genome-wide transcriptome analysis of human papillomavirus 16-infected primary keratinocytes reveals subtle perturbations mostly due to E7 protein expression. J Virol 2020; 94(3): e01360-19, https://doi.org/10.1128/jvi.01360-19.
- Wang X., Wang H.K., Li Y., Hafner M., Banerjee N.S., Tang S., Briskin D., Meyers C., Chow L.T., Xie X., Tuschl T., Zheng Z.M. MicroRNAs are biomarkers of oncogenic human papillomavirus infections. Proc Natl Acad Sci U S A 2014; 111(11): 4262–4267, https://doi.org/10.1073/pnas.1401430111.
- Jackson R., Rosa B.A., Lameiras S., Cuninghame S., Bernard J., Floriano W.B., Lambert P.F., Nicolas A., Zehbe I. Functional variants of human papillomavirus type 16 demonstrate host genome integration and transcriptional alterations corresponding to their unique cancer epidemiology. BMC Genomics 2016; 17(1): 851, https://doi.org/10.1186/s12864-016-3203-3.
- Israr M., Rosenthal D., Frejo-Navarro L., DeVoti J., Meyers C., Bonagura V.R. Microarray analysis of human keratinocytes from different anatomic sites reveals site-specific immune signaling and responses to human papillomavirus type 16 transfection. Mol Med 2018; 24(1): 23, https://doi.org/10.1186/s10020-018-0022-9.
- Chatterjee S., Do Kang S., Alam S., Salzberg A.C., Milici J., van der Burg S.H., Freeman W., Meyers C. Tissue-specific gene expression during productive human papillomavirus 16 infection of cervical, foreskin, and tonsil epithelium. J Virol 2019; 93(17): e00915-19, https://doi.org/10.1128/jvi.00915-19.
- Villa P.L., Jackson R., Eade S., Escott N., Zehbe I. Isolation of biopsy-derived, human cervical keratinocytes propagated as monolayer and organoid cultures. Sci Rep 2018; 8(1): 17869, https://doi.org/10.1038/s41598-018-36150-4.
- Deng H., Hillpot E., Mondal S., Khurana K.K., Woodworth C.D. HPV16-immortalized cells from human transformation zone and endocervix are more dysplastic than ectocervical cells in organotypic culture. Sci Rep 2018; 8(1): 15402, https://doi.org/10.1038/s41598-018-33865-2.
- Zuk A.K., Wen X., Dilworth S., Li D., Ghali L. Modeling and validating three dimensional human normal cervix and cervical cancer tissues in vitro. J Biomed Res 2017; 31(3): 240–247.
- Hougardy B.M., Reesink-Peters N., van den Heuvel F.A., ten Hoor K.A., Hollema H., de Vries E.G., de Jong S., van der Zee A.G. A robust ex vivo model for evaluation of induction of apoptosis by rhTRAIL in combination with proteasome inhibitor MG132 in human premalignant cervical explants. Int J Cancer 2008; 123(6): 1457–1465, https://doi.org/10.1002/ijc.23684.
- Baird J.R., Bell R.B., Troesch V., Friedman D., Bambina S., Kramer G., Blair T.C., Medler T., Wu Y., Sun Z., de Gruijl T.D., van de Ven R., Leidner R.S., Crittenden M.R., Gough M.J. Evaluation of explant responses to STING ligands: personalized immunosurgical therapy for head and neck squamous cell carcinoma. Cancer Res 2018; 78(21): 6308–6319, https://doi.org/10.1158/0008-5472.can-18-1652.
- Hiroshima Y., Maawy A., Zhang Y., Zhang N., Murakami T., Chishima T., Tanaka K., Ichikawa Y., Bouvet M., Endo I., Hoffman R.M. Patient-derived mouse models of cancer need to be orthotopic in order to evaluate targeted anti-metastatic therapy. Oncotarget 2016; 7(44): 71696–71702, https://doi.org/10.18632/oncotarget.12322.
- Chaudary N., Jaluba K., Pintilie M., Hill R.P. Establishment of orthotopic primary cervix cancer xenografts. Methods Mol Biol 2015; 1249: 381–391, https://doi.org/10.1007/978-1-4939-2013-6_28.
- Larmour L.I., Cousins F.L., Teague J.A., Deane J.A., Jobling T.W., Gargett C.E. A patient derived xenograft model of cervical cancer and cervical dysplasia. PLoS One 2018; 13(10): e0206539, https://doi.org/10.1371/journal.pone.0206539.
- Rofstad E.K., Simonsen T.G., Huang R., Andersen L.M., Galappathi K., Ellingsen C., Wegner C.S., Hauge A., Gaustad J.V. Patient-derived xenograft models of squamous cell carcinoma of the uterine cervix. Cancer Lett 2016; 373(2): 147–155, https://doi.org/10.1016/j.canlet.2016.01.047.
- Wegner C.S., Hauge A., Andersen L.M.K., Huang R., Simonsen T.G., Gaustad J.V., Rofstad E.K. Increasing aggressiveness of patient-derived xenograft models of cervix carcinoma during serial transplantation. Oncotarget 2018; 9(30): 21036–21051, https://doi.org/10.18632/oncotarget.24783.
- Zhang X., Yang P., Luo X., Su C., Chen Y., Zhao L., Wei L., Zeng H., Varghese Z., Moorhead J.F., Ruan X.Z., Chen Y. High olive oil diets enhance cervical tumour growth in mice: transcriptome analysis for potential candidate genes and pathways. Lipids Health Dis 2019; 18(1): 76, https://doi.org/10.1186/s12944-019-1023-6.
- Chu J., Gao J., Wang J., Li L., Chen G., Dang J., Wang Z., Jin Z., Liu X. Mechanism of hydrogen on cervical cancer suppression revealed by high‑throughput RNA sequencing. Oncol Rep 2021; 46(1): 141, https://doi.org/10.3892/or.2021.8092.
- Kang H.N., Kim J.H., Park A.Y., Choi J.W., Lim S.M., Kim J., Shin E.J., Hong M.H., Pyo K.H., Yun M.R., Kim D.H., Lee H., Yoon S.O., Kim D.H., Park Y.M., Byeon H.K., Jung I., Paik S., Koh Y.W., Cho B.C., Kim H.R. Establishment and characterization of patient-derived xenografts as paraclinical models for head and neck cancer. BMC Cancer 2020; 20(1): 316, https://doi.org/10.1186/s12885-020-06786-5.
- Tinhofer I., Braunholz D., Klinghammer K. Preclinical models of head and neck squamous cell carcinoma for a basic understanding of cancer biology and its translation into efficient therapies. Cancers Head Neck 2020; 5: 9, https://doi.org/10.1186/s41199-020-00056-4.
- Facompre N.D., Sahu V., Montone K.T., Harmeyer K.M., Nakagawa H., Rustgi A.K., Weinstein G.S., Gimotty P.A., Basu D. Barriers to generating PDX models of HPV-related head and neck cancer. Laryngoscope 2017; 127(12): 2777–2783, https://doi.org/10.1002/lary.26679.
- Lee T.W., Lai A., Harms J.K., Singleton D.C., Dickson B.D., Macann A.M.J., Hay M.P., Jamieson S.M.F. Patient-derived xenograft and organoid models for precision medicine targeting of the tumour microenvironment in head and neck cancer. Cancers (Basel) 2020; 12(12): 3743, https://doi.org/10.3390/cancers12123743.
- Keysar S.B., Le P.N., Miller B., Jackson B.C., Eagles J.R., Nieto C., Kim J., Tang B., Glogowska M.J., Morton J.J., Padilla-Just N., Gomez K., Warnock E., Reisinger J., Arcaroli J.J., Messersmith W.A., Wakefield L.M., Gao D., Tan A.C., Serracino H., Vasiliou V., Roop D.R., Wang X.J., Jimeno A. Regulation of head and neck squamous cancer stem cells by PI3K and SOX2. J Natl Cancer Inst 2016; 109(1): djw189, https://doi.org/10.1093/jnci/djw189.
- Lilja-Fischer J.K., Ulhøi B.P., Alsner J., Stougaard M., Thomsen M.S., Busk M., Lassen P., Steiniche T., Nielsen V.E., Overgaard J. Characterization and radiosensitivity of HPV-related oropharyngeal squamous cell carcinoma patient-derived xenografts. Acta Oncol 2019; 58(10): 1489–1494, https://doi.org/10.1080/0284186x.2019.1660802.
- Klinghammer K., Otto R., Raguse J.D., Albers A.E., Tinhofer I., Fichtner I., Leser U., Keilholz U., Hoffmann J. Basal subtype is predictive for response to cetuximab treatment in patient-derived xenografts of squamous cell head and neck cancer. Int J Cancer 2017; 141(6): 1215–1221, https://doi.org/10.1002/ijc.30808.
- Yegodayev K.M., Novoplansky O., Golden A., Prasad M., Levin L., Jagadeeshan S., Zorea J., Dimitstein O., Joshua B.Z., Cohen L., Khrameeva E., Elkabets M. TGF-beta-activated cancer-associated fibroblasts limit cetuximab efficacy in preclinical models of head and neck cancer. Cancers (Basel) 2020; 12(2): 339, https://doi.org/10.3390/cancers12020339.
- Ko Y.C., Lai T.Y., Hsu S.C., Wang F.H., Su S.Y., Chen Y.L., Tsai M.L., Wu C.C., Hsiao J.R., Chang J.Y., Wu Y.M., Robinson D.R., Lin C.Y., Lin S.F. Index of cancer-associated fibroblasts is superior to the epithelial-mesenchymal transition score in prognosis prediction. Cancers (Basel) 2020; 12(7): 1718, https://doi.org/10.3390/cancers12071718.
- Bhatia S., Sharma J., Bukkapatnam S., Oweida A., Lennon S., Phan A., Milner D., Uyanga N., Jimeno A., Raben D., Somerset H., Heasley L., Karam S.D. Inhibition of EphB4–Ephrin-B2 signaling enhances response to cetuximab-radiation therapy in head and neck cancers. Clin Cancer Res 2018; 24(18): 4539–4550, https://doi.org/10.1158/1078-0432.ccr-18-0327.
- Kim H.R., Kang H.N., Yun M.R., Ju K.Y., Choi J.W., Jung D.M., Pyo K.H., Hong M.H., Ahn M.J., Sun J.M., Kim H.S., Kim J., Yoo J., Kim K.R., Koh Y.W., Kim S.H., Choi E.C., Yoon S.O., Shim H.S., Paik S., Kim T.M., Cho B.C. Mouse–human co-clinical trials demonstrate superior anti-tumour effects of buparlisib (BKM120) and cetuximab combination in squamous cell carcinoma of head and neck. Br J Cancer 2020; 123(12): 1720–1729, https://doi.org/10.1038/s41416-020-01074-2.
- Campbell K.M., Lin T., Zolkind P., Barnell E.K., Skidmore Z.L., Winkler A.E., Law J.H., Mardis E.R., Wartman L.D., Adkins D.R., Chernock R.D., Griffith M., Uppaluri R., Griffith O.L. Oral cavity squamous cell carcinoma xenografts retain complex genotypes and intertumor molecular heterogeneity. Cell Rep 2018; 24(8): 2167–2178, https://doi.org/10.1016/j.celrep.2018.07.058.
- Cosper P.F., Abel L., Lee Y.S., Paz C., Kaushik S., Nickel K.P., Alexandridis R., Scott J.G., Bruce J.Y., Kimple R.J. Patient derived models to study head and neck cancer radiation response. Cancers (Basel) 2020; 12(2): 419, https://doi.org/10.3390/cancers12020419.
- Liu T., Delavaux C., Zhang Y.S. 3D bioprinting for oncology applications. J 3D Print Med 2019; 3(2): 55–58, https://doi.org/10.2217/3dp-2019-0004.
- Cao X., Ashfaq R., Cheng F., Maharjan S., Li J., Ying G., Hassan S., Xiao H., Yue K., Zhang Y.S. A tumor-on-a-chip system with bioprinted blood and lymphatic vessel pair. Adv Funct Mater 2019; 29(31): 1807173, https://doi.org/10.1002/adfm.201807173.
- Meng F., Meyer C.M., Joung D., Vallera D.A., McAlpine M.C., Panoskaltsis-Mortari A. 3D bioprinted in vitro metastatic models via reconstruction of tumor microenvironments. Adv Mater 2019; 31(10): e1806899, https://doi.org/10.1002/adma.201806899.
- Xie F., Sun L., Pang Y., Xu G., Jin B., Xu H., Lu X., Xu Y., Du S., Wang Y., Feng S., Sang X., Zhong S., Wang X., Sun W., Zhao H., Zhang H., Yang H., Huang P., Mao Y. Three-dimensional bio-printing of primary human hepatocellular carcinoma for personalized medicine. Biomaterials 2021; 265: 120416, https://doi.org/10.1016/j.biomaterials.2020.120416.
- Pang Y., Mao S.S., Yao R., He J.Y., Zhou Z.Z., Feng L., Zhang K.T., Cheng S.J., Sun W. TGF-β induced epithelial-mesenchymal transition in an advanced cervical tumor model by 3D printing. Biofabrication 2018; 10(4): 044102, https://doi.org/10.1088/1758-5090/aadbde.
- Gospodinova A., Nankov V., Tomov S., Redzheb M., Petrov P.D. Extrusion bioprinting of hydroxyethylcellulose-based bioink for cervical tumor model. Carbohydr Polym 2021; 260: 117793, https://doi.org/10.1016/j.carbpol.2021.117793.
- Almela T., Al-Sahaf S., Brook I.M., Khoshroo K., Rasoulianboroujeni M., Fahimipour F., Tahriri M., Dashtimoghadam E., Bolt R., Tayebi L., Moharamzadeh K. 3D printed tissue engineered model for bone invasion of oral cancer. Tissue Cell 2018; 52: 71–77, https://doi.org/10.1016/j.tice.2018.03.009.
- Li X., He J., Shao M., Cui B., Peng F., Li J., Ran Y., Jin D., Kong J., Chang J., Duan L., Yang X., Luo Y., Lu Y., Lin B., Liu T. Downregulation of miR-218-5p promotes invasion of oral squamous cell carcinoma cells via activation of CD44-ROCK signaling. Biomed Pharmacother 2018; 106: 646–654, https://doi.org/10.1016/j.biopha.2018.06.151.
- Wang N., Wang J., Meng X., Bao Y., Wang S., Li T. 3D microfluidic in vitro model and bioinformatics integration to study the effects of Spatholobi Caulis tannin in cervical cancer. Sci Rep 2018; 8(1): 12285, https://doi.org/10.1038/s41598-018-29848-y.