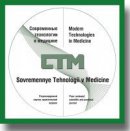
Децеллюляризованный внеклеточный матрикс для тканевой инженерии (обзор)
За последние годы децеллюляризованные ткани превратились в новую, полноценную платформу для создания тканеинженерных конструкций. Внеклеточный матрикс (ВКМ) каждой ткани создает уникальное тканеспецифическое микроокружение для резидентных клеток, обеспечивая их структурой и биохимическими сигналами, необходимыми для их функционирования. Установлено, что децеллюляризованный внеклеточный матрикс (дВКМ) оказывает влияние на дифференцировку клеток.
В обзоре приводятся данные о составе и функциях ВКМ, методах получения децеллюляризованных тканей и применении их в тканевой инженерии в зависимости от физической формы (каркас, порошок или гидрогель). Рассматривается влияние источника матрикса, способа децеллюляризации и стерилизации на состав дВКМ. Обсуждаются механизмы регуляции дифференцировки клеток внеклеточным матриксом. Приводятся различия в белковом составе нативных и децеллюляризованных материалов. Рассматривается применение дВКМ в составе биочернил для регенерации различных тканей с использованием технологий биопечати.
Делается вывод, что для успешного применения дВКМ в тканевой инженерии и регенеративной медицине необходимы постоянный и биологически приемлемый источник дВКМ, оптимизированные протоколы децеллюляризации тканей, улучшение механических свойств биочернил на основе дВКМ и предотвращение иммунологической реакции организма.
- Бекетов Е.Е., Исаева Е.В., Шегай П.В., Иванов С.А., Каприн А.Д. Современное состояние тканевой инженерии для восстановления хрящевой ткани. Гены и клетки 2019; 14(2): 12–20, https://doi.org/10.23868/201906013.
- Agmon G., Christman K.L. Controlling stem cell behavior with decellularized extracellular matrix scaffolds. Curr Opin Solid State Mater Sci 2016; 20(4): 193–201, https://doi.org/10.1016/j.cossms.2016.02.001.
- Hoshiba T., Lu H., Kawazoe N., Chen G. Decellularized matrices for tissue engineering. Expert Opin Biol Ther 2010; 10(12): 1717–1728, https://doi.org/10.1517/14712598.2010.534079.
- Frantz C., Stewart K.M., Weaver V.M. The extracellular matrix at a glance. J Cell Sci 2010; 123(Pt 24): 4195–4200, https://doi.org/10.1242/jcs.023820.
- Clause K.C., Barker T.H. Extracellular matrix signaling in morphogenesis and repair. Curr Opin Biotechnol 2013; 24(5): 830–833, https://doi.org/10.1016/j.copbio.2013.04.011.
- Theocharis A.D., Gialeli C., Hascall V., Karamanos N.K. Extracellular matrix: a functional scaffold. In: Extracellular matrix: pathobiology and signaling. Karamanos N.K. (editor). Berlin: Walter de Gruyter; 2012; p. 3–20, https://doi.org/10.1515/9783110258776.3.
- Cramer M.C., Badylak S.F. Extracellular matrix-based biomaterials and their influence upon cell behavior. Ann Biomed Eng 2020; 48(7): 2132–2153, https://doi.org/10.1007/s10439-019-02408-9.
- Yao Q., Zheng Y.W., Lan Q.H., Kou L., Xu H.L., Zhao Y.Z. Recent development and biomedical applications of decellularized extracellular matrix biomaterials. Mater Sci Eng C Mater Biol Appl 2019; 104: 109942, https://doi.org/10.1016/j.msec.2019.109942.
- Hynes R.O., Naba A. Overview of the matrisome — an inventory of extracellular matrix constituents and functions. Cold Spring Harb Perspect Biol 2012; 4(1): a004903, https://doi.org/10.1101/cshperspect.a004903.
- García-Gareta E., Abduldaiem Y., Sawadkar P., Kyriakidis Ch., Lali F., Greco K.V. Decellularised scaffolds: just a framework? Current knowledge and future directions. J Tissue Eng 2020; 11: 2041731420942903, https://doi.org/10.1177/2041731420942903.
- Jansen K.A., Atherton P., Ballestrem C. Mechanotransduction at the cell-matrix interface. Semin Cell Dev Biol 2017; 71: 75–83, https://doi.org/10.1016/j.semcdb.2017.07.027.
- Aiyelabegan H.T., Sadroddiny E. Fundamentals of protein and cell interactions in biomaterials. Biomed Pharmacother 2017; 88: 956–970, https://doi.org/10.1016/j.biopha.2017.01.136.
- Bonnans C., Chou J., Werb Z. Remodelling the extracellular matrix in development and disease. Nat Rev Mol Cell Biol 2014; 15(12): 786–801, https://doi.org/10.1038/nrm3904.
- Järveläinen H., Sainio A., Koulu M., Wight T.N., Penttinen R. Extracellular matrix molecules: potential targets in pharmacotherapy. Pharmacol Rev 2009; 61(2): 198–223, https://doi.org/10.1124/pr.109.001289.
- Bateman J.F., Boot-Handford R.P., Lamandé S.R. Genetic diseases of connective tissues: cellular and extracellular effects of ECM mutations. Nature Rev Genet 2009; 10(3): 173–183, https://doi.org/10.1038/nrg2520.
- Rozario T., DeSimone D.W. The extracellular matrix in development and morphogenesis: a dynamic view. Dev Biol 2010; 341(1): 126–140, https://doi.org/10.1016/j.ydbio.2009.10.026.
- Theocharis A.D., Skandalis S.S., Gialeli C., Karamanos N.K. Extracellular matrix structure. Adv Drug Deliv Rev 2016; 97: 4–27, https://doi.org/10.1016/j.addr.2015.11.001.
- LeBleu V.S., Macdonald B., Kalluri R. Structure and function of basement membranes. Exp Biol Med (Maywood) 2007; 232(9): 1121–1129, https://doi.org/10.3181/0703-mr-72.
- Kirkpatrick C.A., Selleck S.B. Heparan sulfate proteoglycans at a glance. J Cell Sci 2007; 120(11): 1829–1832, https://doi.org/10.1242/jcs.03432.
- Lu P., Takai K., Weaver V.M., Werb Z. Extracellular matrix degradation and remodeling in development and disease. Cold Spring Harb Perspect Biol 2011; 3(12): a005058, https://doi.org/10.1101/cshperspect.a005058.
- Zhen G., Cao X. Targeting TGFβ signaling in subchondral bone and articular cartilage homeostasis. Trends Pharmacol Sci 2014; 35(5): 227–236, https://doi.org/10.1016/j.tips.2014.03.005.
- Oever M.V., Twaroski K., Osborn M.J., Wagner J.E., Tolar J. Inside out: regenerative medicine for recessive dystrophic epidermolysis bullosa. Pediatr Res 2018; 83(1–2): 318–324, https://doi.org/10.1038/pr.2017.244.
- Gattazzo F., Urciuolo A., Bonaldo P. Extracellular matrix: a dynamic microenvironment for stem cell niche. Biochim Biophys Acta 2014; 1840(8): 2506–2519, https://doi.org/10.1016/j.bbagen.2014.01.010.
- Novoseletskaya E.S., Grigorieva O.A., Efimenko A.Y., Kalinina N.I. Extracellular matrix in the regulation of stem cell differentiation. Biochemistry (Mosc) 2019; 84(3): 232–240, https://doi.org/10.1134/s0006297919030052.
- Novoseletskaya E., Grigorieva O., Nimiritsky P., Basalova N., Eremichev R., Milovskaya I., Kulebyakin K., Kulebyakina M., Rodionov S., Omelyanenko N., Efimenko A. Mesenchymal stromal cell-produced components of extracellular matrix potentiate multipotent stem cell response to differentiation stimuli. Front Cell Dev Biol 2020; 8: 555378, https://doi.org/10.3389/fcell.2020.555378.
- Kim B.S., Das S., Jang J., Cho D.W. Decellularized extracellular matrix-based bioinks for engineering tissue- and organ-specific microenvironments. Chem Rev 2020; 120(19): 10608–10661, https://doi.org/10.1021/acs.chemrev.9b00808.
- Ragelle H., Naba A., Larson B.L., Zhou F., Prijić M., Whittaker C.A., Rosarioa A.D., Langer R., Hynes R.O., Anderson D.G. Comprehensive proteomic characterization of stem cell-derived extracellular matrices. Biomaterials 2017; 128: 147–159, https://doi.org/10.1016/j.biomaterials.2017.03.008.
- Naba A., Clauser K.R., Ding H., Whittaker C.A., Carr S.A., Hynes R.O. The extracellular matrix: tools and insights for the “omics” era. Matrix Biol 2016; 49: 10–24, https://doi.org/10.1016/j.matbio.2015.06.003.
- Yamashita Y.M., Fuller M.T., Jones D.L. Signaling in stem cell niches: lessons from the Drosophila germline. J Cell Sci 2005; 118(4): 665–672, https://doi.org/10.1242/jcs.01680.
- Jung C.S., Kim B.K., Lee J., Min B.H., Park S.H. Development of printable natural cartilage matrix bioink for 3D printing of irregular tissue shape. Tissue Eng Regen Med 2017; 15(2): 155–162, https://doi.org/10.1007/s13770-017-0104-8.
- Ott H.C., Matthiesen T.S., Goh S.K., Black L.D., Kren S.M., Netoff T.I., Taylor D.A. Perfusion-decellularized matrix: using nature’s platform to engineer a bioartificial heart. Nat Med 2008; 14(2): 213–221, https://doi.org/10.1038/nm1684.
- Crapo P.M., Gilbert T.W., Badylak S.F. An overview of tissue and whole organ decellularization processes. Biomaterials 2011; 32(12): 3233–3243, https://doi.org/10.1016/j.biomaterials.2011.01.057.
- Poornejad N., Momtahan N., Salehi A.S., Scott D.R., Fronk C.A., Roeder B.L., Reynolds P.R., Bundy B.C., Cook A.D. Efficient decellularization of whole porcine kidneys improves reseeded cell behavior. Biomed Mater 2016; 11(2): 025003, https://doi.org/10.1088/1748-6041/11/2/025003.
- Choudhury D., Yee M., Sheng Z.L.J., Amirul A., Naing M.W. Decellularization systems and devices: state-of-the-art. Acta Biomater 2020; 115: 51–59, https://doi.org/10.1016/j.actbio.2020.07.060.
- Kabirian F., Mozafari M. Decellularized ECM-derived bioinks: prospects for the future. Methods 2020; 171: 108–118, https://doi.org/10.1016/j.ymeth.2019.04.019.
- Choudhury D., Tun H.W., Wang T., Naing M.W. Organ-derived decellularized extracellular matrix: a game changer for bioink manufacturing? Trends Biotechnol 2018; 36(8): 787–805, https://doi.org/10.1016/j.tibtech.2018.03.003.
- Dzobo K., Motaung K.S.C.M., Adesida A. Recent trends in decellularized extracellular matrix bioinks for 3D printing: an updated review. Int J Mol Sci 2019; 20(18): 4628, https://doi.org/10.3390/ijms20184628.
- Saldin L.T., Cramer M.C., Velankar S.S., White L.J., Badylak S.F. Extracellular matrix hydrogels from decellularized tissues: structure and function. Acta Biomater 2017; 49: 1–15, https://doi.org/10.1016/j.actbio.2016.11.068.
- Johnson T.D., DeQuach J.A., Gaetani R., Ungerleider J., Elhag D., Nigam V., Behfar A., Christman K.L. Human versus porcine tissue sourcing for an injectable myocardial matrix hydrogel. Biomater Sci 2014; 2014: 60283D, https://doi.org/10.1039/c3bm60283d.
- Hynes R.O. The evolution of metazoan extracellular matrix. J Cell Biol 2012; 196(6): 671–679, https://doi.org/10.1083/jcb.201109041.
- LoPresti S.T., Brown B.N. Effect of source animal age upon macrophage response to extracellular matrix biomaterials. J Immunol Regen Med 2018; 1: 57–66, https://doi.org/10.1016/j.regen.2018.03.004.
- Silva A.C., Rodrigues S.C., Caldeira J., Nunes A.M., Sampaio-Pinto V., Resende T.P., Oliveira M.J., Barbosa M.A., Thorsteinsdóttir S., Nascimento D.S., Pinto-do-Ó P. Three-dimensional scaffolds of fetal decellularized hearts exhibit enhanced potential to support cardiac cells in comparison to the adult. Biomaterials 2016; 104: 52–64, https://doi.org/10.1016/j.biomaterials.2016.06.062.
- Tottey S., Johnson S.A., Crapo P.M., Reing J.E., Zhang L., Jiang H., Medberry C.J., Reines B., Badylak S.F. The effect of source animal age upon extracellular matrix scaffold properties. Biomaterials 2011; 32(1): 128–136, https://doi.org/10.1016/j.biomaterials.2010.09.006.
- Williams C., Quinn K.P., Georgakoudi I., Black L.D. III. Young developmental age cardiac extracellular matrix promotes the expansion of neonatal cardiomyocytes in vitro. Acta Biomater 2014; 10(1): 194–204, https://doi.org/10.1016/j.actbio.2013.08.037.
- Wang Z., Long D.W., Huang Y., Chen W.C.W., Kim K., Wang Y. Decellularized neonatal cardiac extracellular matrix prevents widespread ventricular remodeling in adult mammals after myocardial infarction. Acta Biomater 2019; 87: 140–151, https://doi.org/10.1016/j.actbio.2019.01.062.
- Godin L.M., Sandri B.J., Wagner D.E., Meyer C.M., Price A.P., Akinnola I., Weiss D.J., Panoskaltsis-Mortari A.P.M. Decreased laminin expression by human lung epithelial cells and fibroblasts cultured in acellular lung scaffolds from aged mice. PLoS One 2016; 11(3): e0150966, https://doi.org/10.1371/journal.pone.0150966.
- Porzionato A., Stocco E., Barbon S., Grandi F., Macchi V., De Caro R. Tissue-engineered grafts from human decellularized extracellular matrices: a systematic review and future perspectives. Int J Mol Sci 2018; 19(12): 4117, https://doi.org/10.3390/ijms19124117.
- Kočí Z., Výborný K., Dubišová J., Vacková I., Jäger A., Lunov O., Jiráková K., Kubinová Š. Extracellular matrix hydrogel derived from human umbilical cord as a scaffold for neural tissue repair and its comparison with extracellular matrix from porcine tissues. Tissue Eng Part C Methods 2017; 23(6): 333–345, https://doi.org/10.1089/ten.tec.2017.0089.
- Keane T.J., Swinehart I.T., Badylak S.F. Methods of tissue decellularization used for preparation of biologic scaffolds and in vivo relevance. Methods 2015; 84: 25–34, https://doi.org/10.1016/j.ymeth.2015.03.005.
- Badylak S.F., Taylor D., Uygun K. Whole-organ tissue engineering: decellularization and recellularization of three-dimensional matrix scaffolds. Annu Rev Biomed Eng 2011; 13: 27–53, https://doi.org/10.1146/annurev-bioeng-071910-124743.
- Ebrahimi Sadrabadi A., Baei P., Hosseini S., Baghaban Eslaminejad M. Decellularized extracellular matrix as a potent natural biomaterial for regenerative medicine. Adv Exp Med Biol 2021; 1341: 27–43, https://doi.org/10.1007/5584_2020_504.
- Kim Y.S., Majid M., Melchiorri A.J., Mikos A.G. Applications of decellularized extracellular matrix in bone and cartilage tissue engineering. Bioeng Transl Med 2019; 4(1): 83–95, https://doi.org/10.1002/btm2.10110.
- Gilbert T.W., Sellaro T.L., Badylak S.F. Decellularization of tissues and organs. Biomaterials 2006; 27(19): 3675–3683, https://doi.org/10.1016/j.biomaterials.2006.02.014.
- Ghiringhelli M., Zenobi A., Brizzola S., Gandolfi F., Bontempo V., Rossi S., Brevini T.A.L., Acocella F. Simple and quick method to obtain a decellularized, functional liver bioscaffold. Methods Mol Biol 2018; 1577: 283–292, https://doi.org/10.1007/7651_2017_97.
- Kajbafzadeh A.M., Javan-Farazmand N., Monajemzadeh M., Baghayee A. Determining the optimal decellularization and sterilization protocol for preparing a tissue scaffold of a human-sized liver tissue. Tissue Eng Part C Methods 2013; 19(8): 642–651, https://doi.org/10.1089/ten.tec.2012.0334.
- Lu H., Hoshiba T., Kawazoe N., Chen G. Comparison of decellularization techniques for preparation of extracellular matrix scaffold derived from three-dimensional cell culture. J Biomed Mater Res Part A 2012; 100(9): 2507–2516, https://doi.org/10.1002/jbm.a.34150.
- Zhou P., Huang Y., Guo Y., Wang L., Ling C., Guo Q., Wang Y., Zhu S., Fan X., Zhu M., Huang H., Lu Y., Wang Z. Decellularization and recellularization of rat livers with hepatocytes and endothelial progenitor cells. Artif Organs 2016; 40(3): E25–E38, https://doi.org/10.1111/aor.12645.
- Guyette J.P., Gilpin S.E., Charest J.M., Tapias L.F., Ren X., Ott H.C. Perfusion decellularization of whole organs. Nat Protoc 2014; 9(6): 1451–1468, https://doi.org/10.1038/nprot.2014.097.
- Lee H., Han W., Kim H., Ha D.H., Jang J., Kim B.S., Cho D.W. Development of liver decellularized extracellular matrix bioink for three-dimensional cell printing-based liver tissue engineering. Biomacromolecules 2017; 18(4): 1229–1237, https://doi.org/10.1021/acs.biomac.6b01908.
- Skardal A., Devarasetty M., Kang H.W., Mead I., Bishop C., Shupe T., Lee S.J., Jackson J., Yoo J., Soker S., Atala A. A hydrogel bioink toolkit for mimicking native tissue biochemical and mechanical properties in bioprinted tissue constructs. Acta Biomater 2015; 25: 24–34, https://doi.org/10.1016/j.actbio.2015.07.030.
- Gungor-Ozkerim P.S., Inci I., Zhang Y.S., Khademhosseini A., Dokmeci M.R. Bioinks for 3D bioprinting: an overview. Biomater Sci 2018; 6(5): 915–946, https://doi.org/10.1039/c7bm00765e.
- Ahn G., Min K.H., Kim C., Lee J.S., Kang D., Won J.Y., Cho D.W., Kim J.Y., Jin S., Yun W.S., Shim J.H. Precise stacking of decellularized extracellular matrix based 3D cell-laden constructs by a 3D cell printing system equipped with heating modules. Sci Rep 2017; 7(1): 8624, https://doi.org/10.1038/s41598-017-09201-5.
- Hennessy R.S., Jana S., Tefft B.J., Helder M.R., Young M.D., Hennessy R.R., Stoyles N.J., Lerman A. Supercritical carbon dioxide–based sterilization of decellularized heart valves. JACC Basic Transl Sci 2017; 2(1): 71–84, https://doi.org/10.1016/j.jacbts.2016.08.009.
- Helder M.R.K., Hennessy R.S., Spoon D.B., Tefft B.J., Witt T.A., Marler R.J., Pislaru S.V., Simari R.D., Stulak J.M., Lerman A. Low-dose gamma irradiation of decellularized heart valves results in tissue injury in vitro and in vivo. Ann Thorac Surg 2016; 101(2): 667–674, https://doi.org/10.1016/j.athoracsur.2015.07.080.
- Bernhardt A., Wehrl M., Paul B., Hochmuth T., Schumacher M., Schütz K., Gelinsky M. Improved sterilization of sensitive biomaterials with supercritical carbon dioxide at low temperature. PLoS One 2015; 10(6): e0129205, https://doi.org/10.1371/journal.pone.0129205.
- Dearth C.L., Keane T.J., Carruthers C.A., Reing J.E., Huleihel L., Ranallo C.A., Kollar E.W., Badylak S.F. The effect of terminal sterilization on the material properties and in vivo remodeling of a porcine dermal biologic scaffold. Acta Biomater 2016; 33: 78–87, https://doi.org/10.1016/j.actbio.2016.01.038.
- Gilpin A., Yang Y. Decellularization strategies for regenerative medicine: from processing techniques to applications. Biomed Res Int 2017; 2017: 9831534, https://doi.org/10.1155/2017/9831534.
- Fu R.H., Wang Y.C., Liu S.P., Shih T.R., Lin H.L., Chen Y.M., Sung J.H., Lu C.H., Wei J.R., Wang Z.W., Huang S.J., Tsai C.H., Shyu W.C., Lin S.Z. Decellularization and recellularization technologies in tissue engineering. Cell Transplant 2014; 23(4–5): 621–630, https://doi.org/10.3727/096368914x678382.
- Sabetkish S., Kajbafzadeh A.M., Sabetkish N., Khorramirouz R., Akbarzadeh A., Seyedian S.L., Pasalar P., Orangian S., Beigi R.S., Aryan Z., Akbari H., Tavangar S.M. Whole-organ tissue engineering: decellularization and recellularization of three-dimensional matrix liver scaffolds. J Biomed Mater Res A 2015; 103(4): 1498–1508, https://doi.org/10.1002/jbm.a.35291.
- De Santis M.M., Alsafadi H.N., Tas S., Bölükbas D.A., Prithiviraj S., Da Silva I.A.N., Mittendorfer M., Ota C., Stegmayr J.N., Daoud F., Königshoff M., Swärd K., Wood J.A., Tassieri M., Bourgine P.E., Lindstedt S., Mohlin S., Wagner D.E. Extracellular-matrix-reinforced bioinks for 3D bioprinting human tissue. Adv Mater 2021; 33(3): e2005476, https://doi.org/10.1002/adma.202005476.
- Hussey G.S., Dziki J.L., Badylak S.F. Extracellular matrix-based materials for regenerative medicine. Nat Rev Mater 2018; 3(7): 159–173, https://doi.org/10.1038/s41578-018-0023-x.
- Assmann А., Delfs C., Munakata H., Schiffer F., Horstkötter K., Huynh K., Barth M., Stoldt V.R., Kamiya H., Boeken U., Lichtenberg A., Akhyari P. Acceleration of autologous in vivo recellularization of decellularized aortic conduits by fibronectin surface coating. Biomaterials 2013; 34(25): 6015–6026, https://doi.org/10.1016/j.biomaterials.2013.04.037.
- Quinn R.W., Hilbert S.L., Bert A.A., Drake B.W., Bustamante J.A., Fenton J.E., Moriarty S.J., Neighbors S.L., Lofland G.K., Hopkins R.A. Performance and morphology of decellularized pulmonary valves implanted in juvenile sheep. Ann Thorac Surg 2011; 92(1): 131–137, https://doi.org/10.1016/j.athoracsur.2011.03.039.
- Tudorache I., Theodoridis K., Baraki H., Sarikouch S., Bara C., Meyer T., Höffler K., Hartung D., Hilfiker A., Haverich A., Cebotari S. Decellularized aortic allografts versus pulmonary autografts for aortic valve replacement in the growing sheep model: haemodynamic and morphological results at 20 months after implantation. Eur J Cardiothoracic Surg 2015; 49(4): 1228–1238, https://doi.org/10.1093/ejcts/ezv362.
- Etnel J.R.G., Suss P.H., Schnorr G.M., Veloso M., Colatusso D.F., Balbi Filho E.M., Costa F.D.A.D. Fresh decellularized versus standard cryopreserved pulmonary allografts for right ventricular outflow tract reconstruction during the Ross procedure: a propensity-matched study. Eur J Cardiothoracic Surg 2018; 54(3): 434–440, https://doi.org/10.1093/ejcts/ezy079.
- Edgar L., Altamimi A., García Sánchez M., Tamburrinia R., Asthana A., Gazia C., Orlando G. Utility of extracellular matrix powders in tissue engineering. Organogenesis 2018; 14(4): 172–186, https://doi.org/10.1080/15476278.2018.1503771.
- Abaci A., Guvendiren M. Designing decellularized extracellular matrix-based bioinks for 3D bioprinting. Adv Healthc Mater 2020; 9(24): e2000734, https://doi.org/10.1002/adhm.202000734.
- Visscher D.O., Bos E.J., Peeters M., Kuzmin N.V., Groot M.L., Helder M.N., van Zuijlen P.P.M. Cartilage tissue engineering: preventing tissue scaffold contraction using a 3D-printed polymeric cage. Tissue Eng Part C Methods 2016; 22(6): 573–584, https://doi.org/10.1089/ten.tec.2016.0073.
- Reing J.E., Brown B.N., Daly K.A., Freund J.M., Gilbert T.W., Hsiong S.X., Huber A., Kullas K.E., Tottey S., Wolf M.T., Badylak S.F. The effects of processing methods upon mechanical and biologic properties of porcine dermal extracellular matrix scaffolds. Biomaterials 2010; 31(33): 8626–8633, https://doi.org/10.1016/j.biomaterials.2010.07.083.
- Badylak S.F., Freytes D.O., Gilbert T.W. Reprint of: Extracellular matrix as a biological scaffold material: structure and function. Acta Biomater 2015; 23: S17–S26, https://doi.org/10.1016/j.actbio.2015.07.016.
- Murphy S.V., Atala A. 3D bioprinting of tissues and organs. Nat Biotechnol 2014; 32(8): 773–785, https://doi.org/10.1038/nbt.2958.
- Kesti M., Eberhardt C., Pagliccia G., Kenkel D., Grande D., Boss A., Zenobi-Wong M. Bioprinting complex cartilaginous structures with clinically compliant biomaterials. Adv Funct Mater 2015; 25(48): 7406–7417, https://doi.org/10.1002/adfm.201570305.
- Hospodiuk M., Dey M., Sosnoski D., Ozbolat I.T. The bioink: a comprehensive review on bioprintable materials. Biotechnol Adv 2017; 35(2): 217–239, https://doi.org/10.1016/j.biotechadv.2016.12.006.
- Zhang Y., Zhou D., Chen J., Zhang X., Li X., Zhao W., Xu T. Biomaterials based on marine resources for 3D bioprinting applications. Mar Drugs 2019; 17(10): 555, https://doi.org/10.3390/md17100555.
- Аргучинская Н.В., Бекетов Е.Е., Исаева Е.В., Сергеева Н.С., Шегай П.В., Иванов С.А., Каприн А.Д. Материалы для создания тканеинженерных конструкций методом 3D-биопечати при восстановлении хрящевой и мягких тканей. Вестник трансплантологии и искусственных органов 2021; 23(1): 60–74, https://doi.org/10.15825/1995-1191-2021-1-60-74.
- Jang J., Kim T.G., Kim B.S., Kim S.W., Kwon S.M., Cho D.W. Tailoring mechanical properties of decellularized extracellular matrix bioink by vitamin B2-induced photo-crosslinking. Acta Biomater 2016; 33: 88–95, https://doi.org/10.1016/j.actbio.2016.01.013.
- Das S., Kim S.W., Choi Y.J., Lee S., Lee S.H., Kong J.S., Park H.J., Cho D.W., Jang J. Decellularized extracellular matrix bioinks and the external stimuli to enhance cardiac tissue development in vitro. Acta Biomater 2019; 95: 188–200, https://doi.org/10.1016/j.actbio.2019.04.026.
- Won J.Y., Lee M.H., Kim M.J., Min K.H., Ahn G., Han J.S., Jin S., Yun W.S., Shim J.H. A potential dermal substitute using decellularized dermis extracellular matrix derived bio-ink. Artif Cells Nanomed Biotechnol 2019; 47(1): 644–649, https://doi.org/10.1080/21691401.2019.1575842.
- Mao Q., Wang Y., Li Y., Juengpanich S., Li W., Chen M., Yin J., Fu J., Cai X. Fabrication of liver microtissue with liver decellularized extracellular matrix (dECM) bioink by digital light processing (DLP) bioprinting. Mater Sci Eng C Mater Biol Appl 2020; 109: 110625, https://doi.org/10.1016/j.msec.2020.110625.
- Shin Y.J., Shafranek R.T., Tsui J.H., Walcott J., Nelson A., Kim D.H. 3D bioprinting of mechanically tuned bioinks derived from cardiac decellularized extracellular matrix. Acta Biomater 2021; 119: 754–788, https://doi.org/10.1016/j.actbio.2020.11.006.
- Yu C., Ma X., Zhu W., Wang P., Miller K.L., Stupin J., Koroleva-Maharajh A., Hairabedian A., Chen S. Scanningless and continuous 3D bioprinting of human tissues with decellularized extracellular matrix. Biomaterials 2019; 194: 1–13, https://doi.org/10.1016/j.biomaterials.2018.12.009.
- Beck E.C., Barragan M., Tadros M.H., Gehrke S.H., Detamore M.S. Approaching the compressive modulus of articular cartilage with a decellularized cartilage-based hydrogel. Acta Biomater 2016; 38: 94–105, https://doi.org/10.1016/j.actbio.2016.04.019.
- Zhang X., Liu Y., Luo C., Zhai C., Li Z., Zhang Y., Yuan T., Dong S., Zhang J., Fan W. Crosslinker-free silk/decellularized extracellular matrix porous bioink for 3D bioprinting-based cartilage tissue engineering. Mater Sci Eng C Mater Biol Appl 2021; 118: 111388, https://doi.org/10.1016/j.msec.2020.111388.
- Kim M.K., Jeong W., Lee S.M., Kim J.B., Jin S., Kang H.W. Decellularized extracellular matrix-based bio-ink with enhanced 3D printability and mechanical properties. Biofabrication 2020; 12(2): 025003, https://doi.org/10.1088/1758-5090/ab5d80.
- Visscher D.O., Lee H., van Zuijlen P.P.M., Helder M.N., Atala A., Yoo J.J., Lee J.S. A photo-crosslinkable cartilage-derived extracellular matrix bioink for auricular cartilage tissue engineering. Acta Biomater 2021; 121: 193–203, https://doi.org/10.1016/j.actbio.2020.11.029.
- Sood D., Chwalek K., Stuntz E., Pouli D., Du C., Tang-Schomer M., Georgakoudi I., Black L.D. III, Kaplan D.L. Fetal brain extracellular matrix boosts neuronal network formation in 3D bioengineered model of cortical brain tissue. ACS Biomater Sci Eng 2016; 2(1): 131–140, https://doi.org/10.1021/acsbiomaterials.5b00446.
- Nyambat B., Manga Y.B., Chen C.H., Gankhuyag U., Pratomo Wp A., Kumar Satapathy M., Chuang E.Y. New insight into natural extracellular matrix: genipin cross-linked adipose-derived stem cell extracellular matrix gel for tissue engineering. Int J Mol Sci 2020; 21(14): 4864, https://doi.org/10.3390/ijms21144864.
- Nagao R.J., Xu J., Luo P., Xue J., Wang Y., Kotha S., Zeng W., Fu X., Himmelfarb J., Zheng Y. Decellularized human kidney cortex hydrogels enhance kidney microvascular endothelial cell maturation and quiescence. Tissue Eng Part A 2016; 22(19–20): 1140–1150, https://doi.org/10.1089/ten.tea.2016.0213.
- Jang J., Park H.J., Kim S.W., Kim H., Park J.Y., Na S.J., Kim H.J., Park M.N., Choi S.H., Park S.H., Kim S.W., Kwon S.M., Kim P.J., Cho D.W. 3D printed complex tissue construct using stem cell-laden decellularized extracellular matrix bioinks for cardiac repair. Biomaterials 2017; 112: 264–274, https://doi.org/10.1016/j.biomaterials.2016.10.026.
- Pati F., Jang J., Ha D.H., Won Kim S., Rhie J.W., Shim J.H., Kim D.H., Cho D.W. Printing three-dimensional tissue analogues with decellularized extracellular matrix bioink. Nat Commun 2014; 5: 3935, https://doi.org/10.1038/ncomms4935.
- Pati F., Cho D.W. Bioprinting of 3D tissue models using decellularized extracellular matrix bioink. Methods Mol Biol 2017; 1612: 381–390, https://doi.org/10.1007/978-1-4939-7021-6_27.
- Almeida H.V., Eswaramoorthy R., Cunniffe G.M., Buckley C.T., O’Brien F.J., Kelly D.J. Fibrin hydrogels functionalized with cartilage extracellular matrix and incorporating freshly isolated stromal cells as an injectable for cartilage regeneration. Acta Biomater 2016; 36: 55–62, https://doi.org/10.1016/j.actbio.2016.03.008.
- Chang C.H., Chen C.C., Liao C.H., Lin F.N., Hsu Y., Fang H.W. Human acellular cartilage matrix powders as a biological scaffold for cartilage tissue engineering with synovium-derived mesenchymal stem cells. J Biomed Mater Res A 2014; 102(7): 2248–2257, https://doi.org/10.1002/jbm.a.34897.
- Yin H., Wang Y., Sun Z., Sun X., Xu Y., Li P., Meng H., Yu X., Xiao B., Fan T., Wang Y., Xu W., Wang A., Guo Q., Peng J., Lu S. Induction of mesenchymal stem cell chondrogenic differentiation and functional cartilage microtissue formation for in vivo cartilage regeneration by cartilage extracellular matrix-derived particles. Acta Biomater 2016; 33: 96–109, https://doi.org/10.1016/j.actbio.2016.01.024.
- Rothrauff B.B., Shimomura K., Gottardi R., Alexander P.G., Tuan R.S. Anatomical region-dependent enhancement of 3-dimensional chondrogenic differentiation of human mesenchymal stem cells by soluble meniscus extracellular matrix. Acta Biomater 2017; 49: 140–151, https://doi.org/10.1016/j.actbio.2016.11.046.
- Toprakhisar B., Nadernezhad A., Bakirci E., Khani N., Skvortsov G.A., Koc B. Development of bioink from decellularized tendon extracellular matrix for 3D bioprinting. Macromol Biosci 2018; 18(10): e1800024, https://doi.org/10.1002/mabi.201800024.
- Zhao F., Cheng J., Sun M., Yu H., Wu N., Li Z., Zhang J., Li Q., Yang P., Liu Q., Hu X., Ao Y. Digestion degree is a key factor to regulate the printability of pure tendon decellularized extracellular matrix bio-ink in extrusion-based 3D cell printing. Biofabrication 2020; 12(4): 045011, https://doi.org/10.1088/1758-5090/aba411.
- Perez-Valle A., Del Amo C., Andia I. Overview of current advances in extrusion bioprinting for skin applications. Int J Mol Sci 2020; 21(18): 6679, https://doi.org/10.3390/ijms21186679.
- Zuo H., Peng D., Zheng B., Liu X., Wang Y., Wang L., Zhou X., Liu J. Regeneration of mature dermis by transplanted particulate acellular dermal matrix in a rat model of skin defect wound. J Mater Sci Mater Med 2012; 23(12): 2933–2944, https://doi.org/10.1007/s10856-012-4745-9.
- Kim H., Park M.N., Kim J., Jang J., Kim H.K., Cho D.W. Characterization of cornea-specific bioink: high transparency, improved in vivo safety. J Tissue Eng 2019; 10: 2041731418823382, https://doi.org/10.1177/2041731418823382.
- Young D.A., Ibrahim D.O., Hu D., Christman K.L. Injectable hydrogel scaffold from decellularized human lipoaspirate. Acta Biomater 2011; 7(3): 1040–1049, https://doi.org/10.1016/j.actbio.2010.09.035.
- Thitiset T., Damrongsakkul S., Bunaprasert T., Leeanansaksiri W., Honsawek S. Development of collagen/demineralized bone powder scaffolds and periosteum-derived cells for bone tissue engineering application. Int J Mol Sci 2013; 14(1): 2056–2071, https://doi.org/10.3390/ijms14012056.
- Lee H.J., Kim Y.B., Ahn S.H., Lee J.S., Jang C.H., Yoon H., Chun W., Kim G.H. A new approach for fabricating collagen/ECM-based bioinks using preosteoblasts and human adipose stem cells. Adv Healthc Mater 2015; 4(9): 1359–1368, https://doi.org/10.1002/adhm.201500193.
- Zhang X., Dong J. Direct comparison of different coating matrix on the hepatic differentiation from adipose-derived stem cells. Biochem Biophys Res Commun 2015; 456(4): 938–944, https://doi.org/10.1016/j.bbrc.2014.11.004.
- Barthold J.E., St. Martin B.M., Sridhar S.L., Vernerey F., Schneider S.E., Wacquez A., Ferguson V.L., Calve S., Neu C.P. Recellularization and integration of dense extracellular matrix by percolation of tissue microparticles. Adv Funct Mater 2021; 31(35): 2103355, https://doi.org/10.1002/adfm.202103355.
- Cheung H.K., Han T.T., Marecak D.M., Watkins J.F., Amsden B.G., Flynn L.E. Composite hydrogel scaffolds incorporating decellularized adipose tissue for soft tissue engineering with adipose-derived stem cells. Biomaterials 2014; 35(6): 1914–1923, https://doi.org/10.1016/j.biomaterials.2013.11.067.
- French K.M., Boopathy A.V., DeQuach J.A., Chingozha L., Lu H., Christman K.L., Davis M.E. A naturally derived cardiac extracellular matrix enhances cardiac progenitor cell behavior in vitro. Acta Biomater 2012; 8(12): 4357–4364, https://doi.org/10.1016/j.actbio.2012.07.033.
- Bonandrini B., Figliuzzi M., Papadimou E., Morigi M., Perico N., Casiraghi F., Dipl C., Sangalli F., Conti S., Benigni A., Remuzzi A., Remuzzi G. Recellularization of well-preserved acellular kidney scaffold using embryonic stem cells. Tissue Eng Part A 2014; 20(9–10): 1486–1498, https://doi.org/10.1089/ten.tea.2013.0269.
- Daly A.B., Wallis J.M., Borg Z.D., Bonvillain R.W., Deng B., Ballif B.A., Jaworski D.M., Allen G.B., Weiss D.J. Initial binding and recellularization of decellularized mouse lung scaffolds with bone marrow-derived mesenchymal stromal cells. Tissue Eng Part A 2012; 18(1–2): 1–16, https://doi.org/10.1089/ten.tea.2011.0301.