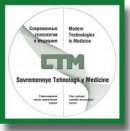
Экспериментальные модели для исследования структурно-функционального состояния печени при развитии патологии (обзор)
Патологии печени по-прежнему остаются одними из лидирующих причин смертности населения во всем мире. Несмотря на их широкое распространение, возможности диагностики, прогноза и терапии неалкогольной и алкогольной болезней печени до сих пор ограничены и требуют разработки новых методов и подходов. В лабораторных исследованиях для воссоздания патологических состояний печени используют различные модели, включающие клеточные культуры, сфероиды, органоиды, микрофлюидные системы, тканевые эксплантаты.
Рассмотрены наиболее часто используемые in vivo, in vitro и ex vivo модели для изучения неалкогольной жировой и алкогольной болезней печени, токсического повреждения печени и фиброза, описаны их достоинства, ограничения, а также перспективы применения. Большой упор сделан на механизмы развития патологических состояний в каждой модели, а также оценку возможности воссоздания различных ключевых аспектов патогенеза для всех указанных патологий.
В настоящее время нет единого мнения по поводу выбора наиболее адекватной модели для изучения патологии печени. Эффективная модель для исследования определяется конкретной целью и задачами эксперимента.
- Maiers J., Malhi H. Endoplasmic reticulum stress in metabolic liver diseases and hepatic fibrosis. Semin Liver Dis 2019; 39(2): 235–248, https://doi.org/10.1055/s-0039-1681032.
- Priore P., Cavallo A., Gnoni A., Damiano F., Gnoni G.V., Siculella L. Modulation of hepatic lipid metabolism by olive oil and its phenols in nonalcoholic fatty liver disease. IUBMB Life 2015; 67(1): 9–17, https://doi.org/10.1002/iub.1340.
- World Health Organization. Global status report on alcohol and health 2018. WHO; 2019.
- Toshikuni N., Tsutsumi M., Arisawa T. Clinical differences between alcoholic liver disease and nonalcoholic fatty liver disease. World J Gastoentrol 2014; 20(26): 8393–8406, https://doi.org/10.3748/wjg.v20.i26.8393.
- Altamirano J., Miquel R., Katoonizadeh A., Abraldes J.G., Duarte-Rojo A., Louvet A., Augustin S., Mookerjee R.P., Michelena J., Smyrk T.C., Buob D., Leteurtre E., Rincón D., Ruiz P., García-Pagán J.C., Guerrero-Marquez C., Jones P.D., Barritt A.S. IV, Arroyo V., Bruguera M., Bañares R., Ginès P., Caballería J., Roskams T., Nevens F., Jalan R., Mathurin P., Shah V.H., Bataller R. A histologic scoring system for prognosis of patients with alcoholic hepatitis. Gastroenterology 2014; 146(5): 1231–1239.e6, https://doi.org/10.1053/j.gastro.2014.01.018.
- Gamboa J.M., Leong K.W. In vitro and in vivo models for the study of oral delivery of nanoparticles. Adv Drug Deliv Rev 2013; 65(6): 800–810, https://doi.org/10.1016/j.addr.2013.01.003.
- Ramachandran A., Lebofsky M., Baines C.P., Lemasters J.J., Jaeschke H. Cyclophilin D deficiency protects against acetaminophen-induced oxidant stress and liver injury. Free Radic Res 2011; 45(2): 156–164, https://doi.org/10.3109/10715762.2010.520319.
- Sjögren E., Abrahamsson B., Augustijns P., Becker D., Bolger M.B., Brewster M., Brouwers J., Flanagan T., Harwood M., Heinen C., Holm R., Juretschke H.P., Kubbinga M., Lindahl A., Lukacova V., Munster U., Neuhoff S., Nguyen M.A., Peer A., Reppas C., Hodjegan A.R., Tannergren C., Weitschies W., Wilson C., Zane P., Lennernäs H., Langguth P. In vivo methods for drug absorption — comparative physiologies, model selection, correlations with in vitro methods (IVIVC), and applications for formulation excipient characterization including food effects. Eur J Pharm Sci 2014; 57: 99–151, https://doi.org/10.1016/j.ejps.2014.02.010.
- Akhtar A. The flaws and human harms of animal experimentation. Camb Q Healthc Ethics 2015; 24(4): 407–419, https://doi.org/10.1017/s0963180115000079.
- Lozoya-Agullo I., Araújo F., González-Álvarez I., Merino-Sanjuán M., González-Álvarez M., Bermejo M., Sarmento B. Usefulness of Caco-2/HT29- MTX and Caco-2/HT29-MTX/Raji B coculture models to predict intestinal and colonic permeability compared to Caco-2 monoculture. Mol Pharm 2017; 14(4): 1264–1270, https://doi.org/10.1021/acs.molpharmaceut.6b01165.
- Polli J.E. In vitro studies are sometimes better than conventional human pharmacokinetic in vivo studies in assessing bioequivalence of immediate-release solid oral dosage forms. AAPS J 2008; 10(2): 289–299, https://doi.org/10.1208/s12248-008-9027-6.
- Katt M.E., Placone A.L., Wong A.D., Xu Z.S., Searson P.C. In vitro tumor models: advantages, disadvantages, variables, and selecting the right platform. Front Bioeng Biotechnol 2016; 4: 12, https://doi.org/10.3389/fbioe.2016.00012.
- Cong Y., Han X., Wang Y., Chen Z., Lu Y., Liu T., Wu Z., Jin Y., Luo Y., Zhang X. Drug toxicity evaluation based on organ-on-a-chip technology: a review. Micromachines (Basel) 2020; 11(4): 381, https://doi.org/10.3390/mi11040381.
- Xu Y., Shrestha N., Préat V., Beloqui A. An overview of in vitro, ex vivo and in vivo models for studying the transport of drugs across intestinal barriers. Adv Drug Deliv Rev 2021; 175: 113795, https://doi.org/10.1016/j.addr.2021.05.005.
- Thomson A., Smart K., Somerville M.S., Lauder S.N., Appanna G., Horwood J., Sunder Raj L., Srivastava B., Durai D., Scurr M.J., Keita Å.V., Gallimore A.M., Godkin A. The Ussing chamber system for measuring intestinal permeability in health and disease. BMC Gastroenterol 2019; 19(1): 98, https://doi.org/10.1186/s12876-019-1002-4.
- Szalowska E., Stoopen G., Rijk J.C., Wang S., Hendriksen P.J., Groot M.J., Ossenkoppele J., Peijnenburg A.A. Effect of oxygen concentration and selected protocol factors on viability and gene expression of mouse liver slices. Toxicol in Vitro 2013; 27(5): 1513–1524, https://doi.org/10.1016/j.tiv.2013.03.007.
- Petrasek J., Bala S., Csak T., Lippai D., Kodys K., Menashy V., Barrieau M., Min S.Y., Kurt-Jones E.A., Szabo G. IL-1 receptor antagonist ameliorates inflammasomedependent alcoholic steatohepatitis in mice. J Clin Invest 2012; 122(10): 3476–3489, https://doi.org/10.1172/jci60777.
- Bertola A., Mathews S., Ki S.H., Wang H., Gao B. Mouse model of chronic and binge ethanol feeding (the NIAAA model). Nat Protoc 2013; 8(3): 627–637, https://doi.org/10.1038/nprot.2013.032.
- Gao B., Xu M.J., Bertola A., Wang H., Zhou Z., Liangpunsakul S. Animal models of alcoholic liver disease: pathogenesis and clinical relevance. Gen Expr 2017; 17(3): 173–186, https://doi.org/10.3727/105221617x695519.
- Zhou Z., Xu M.J., Cai Y. Wang W., Jiang J.X., Varga Z.V., Feng D., Pacher P., Kunos G., Torok N.J., Gao B. Neutrophil-hepatic stellate cell interactions promote fibrosis in experimental steatohepatitis. Cell Mol Gastroenterol Hepatol 2018; 5(3): 399–413, https://doi.org/10.1016/j.jcmgh.2018.01.003.
- Khanova E., Wu R., Wang W., Yan R., Chen Y., French S.W., Llorente C., Pan S.Q., Yang Q., Li Y., Lazaro R., Ansong C., Smith R.D., Bataller R., Morgan T., Schnabl B., Tsukamoto H. Pyroptosis by caspase11/4-gasdermin-D pathway in alcoholic hepatitis in mice and patients. Hepatology 2018; 67(5): 1737–1753, https://doi.org/10.1002/hep.29645.
- Baraona E., Lieber C.S. Alcohol and lipids. Recent Dev Alcohol 1998; 14: 97–134, https://doi.org/10.1007/0-306-47148-5_5.
- Lieber C.S. Alcohol: its metabolism and interaction with nutrients. Annu Rev Nutr 2000; 20: 395–430, https://doi.org/10.1146/annurev.nutr.20.1.395.
- Kaplowitz N., Than T.A., Shinohara M., Ji C. Endoplasmic reticulum stress and liver injury. Semin Liver Dis 2007; 27(4): 367–377, https://doi.org/10.1055/s-2007-991513.
- Albano E. Oxidative mechanisms in the pathogenesis of alcoholic liver disease. Mol Aspects Med 2008; 29(1-2): 9–16, https://doi.org/10.1016/j.mam.2007.09.004.
- Petrasek J., Iracheta-Vellve A., Csak T., Satishchandran A., Kodys K., Kurt-Jones E.A., Fitzgerald K.A., Szabo G. STING-IRF3 pathway links endoplasmic reticulum stress with hepatocyte apoptosis in early alcoholic liver disease. Proc Natl Acad Sci U S A 2013; 110(41): 16544–16549, https://doi.org/10.1073/pnas.1308331110.
- Tsukamoto H., French S.W., Rektelberger R.D., Largman C. Cyclical pattern of blood alcohol levels during continuous intragastric ethanol infusion in rats. Alcohol Clin Exp Res 1985; 9(1): 31–37, https://doi.org/10.1111/j.1530-0277.1985.tb05046.x.
- French S.W. Intragastric ethanol infusion model for cellular and molecular studies of alcoholic liver disease. J Biomed Sci 2001; 8(1): 20–27, https://doi.org/10.1159/000054009.
- Palma E., Doornebal E.J., Chokshi S. Precision-cut liver slices: a versatile tool to advance liver research. Hepatol Int 2019; 13(1): 51–57, https://doi.org/10.1007/s12072-018-9913-7.
- Affò S., Dominguez M., Lozano J.J., Sancho-Bru P., Rodrigo-Torres D., Morales-Ibanez O., Moreno M., Millán C., Loaeza-del-Castillo A., Altamirano J., García-Pagán J.C., Arroyo V., Ginès P., Caballería J., Schwabe R.F., Bataller R. Transcriptome analysis identifies TNF superfamily receptors as potential therapeutic targets in alcoholic hepatitis. Gut 2013; 62(3): 452–460, https://doi.org/10.1136/gutjnl-2011-301146.
- Avila M.A., Dufour J.F., Gerbes A.L., Zoulim F., Bataller R., Burra P., Cortez-Pinto H., Gao B., Gilmore I., Mathurin P., Moreno C., Poznyak V., Schnabl B., Szabo G., Thiele M., Thursz M.R. Recent advances in alcohol-related liver disease (ALD): summary of a Gut round table meeting. Gut 2019; 69(4): 764–780, https://doi.org/10.1136/gutjnl-2019-319720.
- Weber L.W.D., Boll M., Stampfl A. Hepatotoxicity and mechanism of action of haloalkanes: carbon tetrachloride as a toxicological model. Crit Rev Toxicol 2003; 33(2): 105–136, https://doi.org/10.1080/713611034.
- Tanaka E., Terada M., Misawa S. Cytochrome P450 2E1: Its clinical and toxicological role. J Clin Pharm Ther 2000; 25(3): 165–175, https://doi.org/10.1046/j.1365-2710.2000.00282.x.
- Basu S. Carbon tetrachloride-induced lipid peroxidation: eicosanoid formation and their regulation by antioxidant nutrients. Toxicology 2003; 189(1–2): 113–127, https://doi.org/10.1016/s0300-483x(03)00157-4.
- Сastro J.A. Mechanistic studies and prevention of free radical cell injury. In: Proceedings of IUPHAR 9th International Congress on Pharmacology. Paton W., Mitchell J., Turner P. (editors). London; 1984; p. 243–250, https://doi.org/10.1007/978-1-349-86029-6_34.
- Heindryckx F., Colle I., Van Vlierberghe H. Experimental mouse models for hepatocellular carcinoma research. Int J Exp Pathol 2009; 90(4): 367–386, https://doi.org/10.1111/j.1365-2613.2009.00656.x.
- Iwaisako K., Jiang C., Zhang M., Cong M., Moore-Morris T.J., Park T.J., Liu X., Xu J., Wang P., Paik Y.H., Meng F., Asagiri M., Murray L.A., Hofmann A.F., Iida T., Glass C.K., Brenner D.A., Kisseleva T. Origin of myofibroblasts in the fibrotic liver in mice. Proc Natl Acad Sci U S A 2014; 111(32): 3297–3305, https://doi.org/10.1073/pnas.1400062111.
- Walkin L., Herrick S.E., Summers A., Brenchley P.E., Hoff C.M., Korstanje R., Margetts P.J. The role of mouse strain differences in the susceptibility to fibrosis: a systematic review. Fibrogenesis Tissue Repair 2013; 6(1): 18, https://doi.org/10.1186/1755-1536-6-18.
- Constandinou C., Henderson N., Iredale J.P. Modeling liver fibrosis in rodents. In: Fibrosis research. Methods and protocols. Varga J., Brenner D.A., Phan S.H. (editors). Humana Press; 2005; p. 237–250, https://doi.org/10.1385/1-59259-940-0:237.
- Yanguas S.C., Cogliati B., Willebrords J., Maes M., Colle I., van den Bossche B., de Oliveira C.P.M.S., Andraus W., Alves V.A.F., Leclercq I., Vinken M. Experimental models of liver fibrosis. Arch Toxicol 2016; 90(5): 1025–1048, https://doi.org/10.1007/s00204-015-1543-4.
- Jang J.H., Kang K.J., Kim Y.H., Kang Y.N., Lee I.S. Reevaluation of experimental model of hepatic fibrosis induced by hepatotoxic drugs: an easy, applicable, and reproducible model. Transplant Proc 2008; 40(8): 2700–2703, https://doi.org/10.1016/j.transproceed.2008.07.040.
- Scholten D., Trebicka J., Liedtke C., Weiskirchen R. The carbon tetrachloride model in mice. Lab Anim 2015; 49(1 Suppl): 4–11, https://doi.org/10.1177/0023677215571192.
- Bertolini A., Ferrari A., Ottani A., Guerzoni S., Tacchi R., Leone S. Paracetamol: new vistas of an old drug. CNS Drug Rev 2006; 12(3–4): 250–275, https://doi.org/10.1111/j.1527-3458.2006.00250.x.
- Pu S., Ren L., Liu Q., Kuang J., Shen J., Cheng S., Zhang Y., Jiang W., Zhang Z., Jiang C., He J. Loss of 5-lipoxygenase activity protects mice against paracetamol-induced liver toxicity. Br J Pharmacol 2016; 173(1): 66–76, https://doi.org/10.1111/bph.13336.
- Abdul Hamid Z., Budin S.B., Wen Jie N., Hamid A., Husain K., Mohamed J. Nephroprotective effects of Zingiber zerumbet Smith ethyl acetate extract against paracetamol-induced nephrotoxicity and oxidative stress in rats. J Zhejiang Univ Sci B 2012; 13(3): 176–185, https://doi.org/10.1631/jzus.b1100133.
- Hohmann M.S., Cardoso R.D.R., Fattori V., Arakawa N.S., Tomaz J.C., Lopes N.P., Casagrande R., Verri W.A. Jr. Hypericum perforatum reduces paracetamol-induced hepatotoxicity and lethality in mice by modulating inflammation and oxidative stress. Phytother Res 2015; 29(7): 1097–1101, https://doi.org/10.1002/ptr.5350.
- Das S., Roy P., Ghosh Auddy R., Mukherjee A. Silymarin nanoparticle prevents paracetamol-induced hepatotoxicity. Int J Nanomed 2011; 6: 1291–1301, https://doi.org/10.2147/ijn.s15160.
- Naggayi M., Mukiibi N., Iliya E. The protective effects of aqueous extract of Carica papaya seeds in paracetamol induced nephrotoxicity in male Wistar rats. Afr Health Sci 2015; 15(2): 598–605, https://doi.org/10.4314/ahs.v15i2.37.
- Lahouel M., Boulkour S., Segueni N., Fillastre J.P. The flavonoids effect against vinblastine, cyclophosphamide and paracetamol toxicity by inhibition of lipid-peroxidation and increasing liver glutathione concentration. Pathol Biol (Paris) 2004; 52(6): 314–322, https://doi.org/10.1016/j.patbio.2004.01.001.
- Firdous A.P., Sindhu E.R., Kuttan R. Hepato-protective potential of carotenoid meso-zeaxanthin against paracetamol, CCl4 and ethanol induced toxicity. Indian J Exp Biol 2011; 49(1): 44–49.
- Arafa H.M. Carnitine deficiency: a possible risk factor in paracetamol hepatotoxicity. Arch Toxicol 2009; 83(2): 139–150, https://doi.org/10.1007/s00204-008-0330-x.
- Madkour F.F., Abdel-Daim M.M. Hepatoprotective and antioxidant activity of Dunaliella salina in paracetamol-induced acute toxicity in rats. Indian J Pharm Sci 2013; 75(6): 642.
- Zubairi M.B., Ahmed J.H., Al-Haroon S.S. Effect of adrenergic blockers, carvedilol, prazosin, metoprolol and combination of prazosin and metoprolol on paracetamolinduced hepatotoxicity in rabbits. Indian J Pharmacol 2014; 46(6): 644–648, https://doi.org/10.4103/0253-7613.144937.
- Ekor M., Odewabi A.O., Kale O.E., Bamidele T.O., Adesanoye O.A., Farombi E.O. Modulation of paracetamol-induced hepatotoxicity by phosphodiesterase isozyme inhibition in rats: a preliminary study. J Basic Clin Physiol Pharmacol 2013; 24(1): 73–79, https://doi.org/10.1515/jbcpp-2012-0043.
- Polat M., Cerrah S., Albayrak B., Ipek S., Arabul M., Aslan F., Yilmaz O. Assessing the effect of leptin on liver damage in case of hepatic injury associated with paracetamol poisoning. Gastroenterol Res Pract 2015; 2015: 357360, https://doi.org/10.1155/2015/357360.
- Wang X., Wu Q., Liu A., Anadón A., Rodríguez J.L., Martínez-Larrañaga M.R., Yuan Z., Martínez M.A. Paracetamol: overdose-induced oxidative stress toxicity, metabolism, and protective effects of various compounds in vivo and in vitro. Drug Metab Rev 2017; 49(4): 395–437, https://doi.org/10.1080/03602532.2017.1354014.
- Ihsan A., Wang X., Liu Z., Wang Y., Huang X., Liu Y., Yu H., Zhang H., Li T., Yang C., Yuan Z. Long-term mequindox treatment induced endocrine and reproductive toxicity via oxidative stress in male Wistar rats. Toxicol Appl Pharmacol 2011; 252(3): 281–288, https://doi.org/10.1016/j.taap.2011.02.020.
- Wang Y., Li D., Cheng N., Gao H., Xue X., Cao W., Sun L. Antioxidant and hepatoprotective activity of vitex honey against paracetamol induced liver damage in mice. Food Funct 2015; 6(7): 2339–2349, https://doi.org/10.1039/c5fo00345h.
- Wang X., Wu Q., Wan D., Liu Q., Chen D., Liu Z., Martínez-Larrañaga M.R., Martínez M.A., Anadón A., Yuan Z. Fumonisins: oxidative stress-mediated toxicity and metabolism in vivo and in vitro. Arch Toxicol 2016; 90(1): 81–101, https://doi.org/10.1007/s00204-015-1604-8.
- Karcioglu S.S., Palabiyik S.S., Bayir Y., Karakus E., Mercantepe T., Halici Z., Albayrak A. The role of RAAS inhibition by Aliskiren on paracetamol-induced hepatotoxicity model in rats. J Cell Biochem 2015; 117(3): 638–646, https://doi.org/10.1002/jcb.25313.
- Galal R.M., Zaki H.F., Seif El-Nasr M.M., Agha A.M. Potential protective effect of honey against paracetamol-induced hepatotoxicity. Arch Iran Med 2012; 15(11): 674–680.
- Karakus E., Halici Z., Albayrak A., Polat B., Bayir Y., Kiki I., Cadirci E., Topcu A., Aksak S. Agomelatine: an antidepressant with new potent hepatoprotective effects on paracetamol-induced liver damage in rats. Hum Exp Toxicol 2013; 32(8): 846–857, https://doi.org/10.1177/0960327112472994.
- Rasool M.K., Sabina E.P., Ramya S.R. Hepatoprotective and antioxidant effects of gallic acid in paracetamol-induced liver damage in mice. J Pharm Pharmacol 2010; 62(5): 638–643, https://doi.org/10.1211/jpp.62.05.0012.
- Anstee Q.M., Goldin R.D. Mouse models in non-alcoholic fatty liver disease and steatohepatitis research. Int J Exp Pathol 2006; 87(1): 1–16, https://doi.org/10.1111/j.0959-9673.2006.00465.x.
- Farrell G.C., Mridha A.R., Yeh M.M., Arsov T., Van Rooyen D.M., Brooling J., Nguyen T., Heydet D., Delghingaro-Augusto V., Nolan C.J., Shackel N.A., McLennan S.V., Teoh N.C., Larter C.Z. Strain dependence of diet-induced NASH and liver fibrosis in obese mice is linked to diabetes and inflammatory phenotype. Liver Int 2014; 34(7): 1084–1093, https://doi.org/10.1111/liv.12335.
- Hansen B.C., Liang Z., Sun F., Yang Z., Tang C., Chen Z., Yubo S., Yao Z., Wu M., Chen Y., Gao F., Zeng W. Nonalcoholic fatty liver disease (NAFLD) in obese rhesus monkeys provides the first animal model that accurately reflects the human condition. FASEB J 2017; 31(S1): 895.6–895.6, https://doi.org/10.1096/fasebj.31.1_supplement.895.6.
- Itagaki H., Shimizu K., Morikawa S., Ogawa K., Ezaki T. Morphological and functional characterization of non-alcoholic fatty liver disease induced by a methionine-choline-deficient diet in C57BL/6 mice. Int J Clin Exp Pathol 2013; 6(12): 2683–2696.
- Cole L.K., Vance J.E., Vance D.E. Phosphatidylcholine biosynthesis and lipoprotein metabolism. Biochim Biophys Acta 2012; 1821(5): 754–761, https://doi.org/10.1016/j.bbalip.2011.09.009.
- Gibbons G.F., Wiggins D., Brown A.M., Hebbachi A.M. Synthesis and function of hepatic very-low-density lipoprotein. Biochem Soc Trans 2004; 32(1): 59–64, https://doi.org/10.1042/bst0320059.
- Lu S.C. Regulation of glutathione synthesis. Mol Asp Med 2009; 30(1–2): 42–59, https://doi.org/10.1016/j.mam.2008.05.005.
- Greene M.W., Burrington C.M., Lynch D.T., Davenport S.K., Johnson A.K., Horsman M.J., Chowdhry S., Zhang J., Sparks J.D., Tirrell P.C. Lipid metabolism, oxidative stress and cell death are regulated by PKC delta in a dietary model of nonalcoholic steatohepatitis. PLoS One 2014; 9(1): e85848, https://doi.org/10.1371/journal.pone.0085848.
- Tosello-Trampont A.C., Landes S.G., Nguyen V., Novobrantseva T.I., Hahn Y.S. Kuppfer cells trigger nonalcoholic steatohepatitis development in dietinduced mouse model through tumor necrosis factor-α production. J Biol Chem 2012; 287(48): 40161–40172, https://doi.org/10.1074/jbc.m112.417014.
- Ramadori P., Weiskirchen R., Trebicka J., Streetz K. Mouse models of metabolic liver injury. Lab Anim 2015; 49(Suppl 1): 47–58, https://doi.org/10.1177/0023677215570078.
- Rinella M.E., Green R.M. The methionine-choline deficient dietary model of steatohepatitis does not exhibit insulin resistance. J Hepatol 2004; 40(1): 47–51, https://doi.org/10.1016/j.jhep.2003.09.020.
- Larter C.Z., Yeh M.M., Williams J., Bell-Anderson K.S., Farrell G.C. MCD-induced steatohepatitis is associated with hepatic adiponectin resistance and adipogenic transformation of hepatocytes. J Hepatol 2008; 49(3): 407–416, https://doi.org/10.1016/j.jhep.2008.03.026.
- Yamazaki Y., Kakizaki S., Takizawa D., Ichikawa T., Sato K., Takagi H., Mori M. Interstrain differences in susceptibility to non-alcoholic steatohepatitis. J Gastroenterol Hepatol 2008; 23(2): 276–282, https://doi.org/10.1111/j.1440-1746.2007.05150.x.
- Passman A.M., Strauss R.P., McSpadden S.B., Finch-Edmondson M.L., Woo K.H., Diepeveen L.A., London R., Callus B.A., Yeoh G.C. A modified choline-deficient, ethionine-supplemented diet reduces morbidity and retains a liver progenitor cell response in mice. Dis Model Mech 2015; 8(12): 1635–1641, https://doi.org/10.1242/dmm.022020.
- Ochoa-Callejero L., Pérez-Martínez L., Rubio-Mediavilla S., Oteo J.A., Martínez A., Blanco J.R. Maraviroc, a CCR5 antagonist, prevents development of hepatocellular carcinoma in a mouse model. PLoS One 2013; 8(1): e53992, https://doi.org/10.1371/journal.pone.0053992.
- Gogoi-Tiwari J., Köhn-Gaone J., Giles C., Schmidt-Arras D., Gratte F.D., Elsegood C.L., McCaughan G.W., Ramm G.A., Olynyk J.K., Tirnitz-Parker J.E.E. The murine choline-deficient, ethionine-supplemented (CDE) diet model of chronic liver injury. J Vis Exp 2017; 128: e56138, https://doi.org/10.3791/56138.
- Passman A.M., Strauss R.P., McSpadden S.B., Finch-Edmondson M.L., Woo K.H., Diepeveen L.A., London R., Callus B.A., Yeoh G.C. A modified choline-deficient, ethionine-supplemented diet reduces morbidity and retains a liver progenitor cell response in mice. Dis Model Mech 2015; 8(12): 1635–1641, https://doi.org/10.1242/dmm.022020.
- Nevzorova Y.A., Boyer-Diaz Z., Cubero F.J., Gracia-Sancho J. Animal models for liver disease — a practical approach for translational research. J Hepatol 2020; 73(2): 423–440, https://doi.org/10.1016/j.jhep.2020.04.011.
- Hansen H.H., Feigh M., Veidal S.S., Rigbolt K.T., Vrang N., Fosgerau K. Mouse models of nonalcoholic steatohepatitis in preclinical drug development. Drug Discov Today 2017; 22(11): 1707–1718, https://doi.org/10.1016/j.drudis.2017.06.007.
- Hebbard L., George J. Animal models of nonalcoholic fatty liver disease. Nat Rev Gastroenterol Hepatol 2011; 8: 35–44, https://doi.org/10.1038/nrgastro.2010.191.
- Pedersen H.D., Galsgaard E.D., Christoffersen B.Ø., Cirera S., Holst D., Fredholm M., Latta M. NASH-inducing diets in Göttingen minipigs. J Clin Exp Hepatol 2020; 10(3): 211–221, https://doi.org/10.1016/j.jceh.2019.09.004.
- Hariri N., Thibault L. High-fat diet-induced obesity in animal models. Nutr Res Rev 2010; 23(2): 270–299, https://doi.org/10.1017/s0954422410000168.
- White P.J., Marette A. Potential role of omega-3-derived resolution mediators in metabolic inflammation. Immun Cell Biol 2014; 92(4): 324–330, https://doi.org/10.1038/icb.2013.112.
- Ito M., Suzuki J., Tsujioka S., Sasaki M., Gomori A., Shirakura T., Hirose H., Ito M., Ishihara A., Iwaasa H., Kanatani A. Longitudinal analysis of murine steatohepatitis model induced by chronic exposure to high-fat diet. Hepatol Res 2007; 37(1): 50–57, https://doi.org/10.1111/j.1872-034x.2007.00008.x.
- Asgharpour A., Cazanave S.C., Pacana T., Seneshaw M., Vincent R., Banini B.A., Kumar D.P., Daita K., Min H.K., Mirshahi F. A diet-induced animal model of non-alcoholic fatty liver disease and hepatocellular cancer. J Hepatol 2016; 65(3): 579–588, https://doi.org/10.1016/j.jhep.2016.05.005.
- Montgomery M.K., Hallahan N.L., Brown S.H., Liu M., Mitchell T.W., Cooney G.J., Turner N. Mouse strain-dependent variation in obesity and glucose homeostasis in response to high-fat feeding. Diabetologia 2013; 56(5): 1129–1139, https://doi.org/10.1007/s00125-013-2846-8.
- Ibrahim S.H., Hirsova P., Malhi H., Gores G.J. Animal models of nonalcoholic steatohepatitis: eat, delete, and inflame. Dig Dis Sci 2016; 61(5): 1325–1336, https://doi.org/10.1007/s10620-015-3977-1.
- Ichimura M., Kawase M., Masuzumi M., Sakaki M., Nagata Y., Tanaka K., Suruga K., Tamaru S., Kato S., Tsuneyama K., Omagari K. High-fat and high-cholesterol diet rapidly induces non-alcoholic steatohepatitis with advanced fibrosis in Sprague-Dawley rats. Hepatol Res 2014; 45(4): 458–469, https://doi.org/10.1111/hepr.12358.
- Kopp W. How Western diet and lifestyle drive the pandemic of obesity and civilization diseases. Diabetes Metab Syndr Obes 2019; 12: 2221–2236, https://doi.org/10.2147/dmso.s216791.
- Kohli R., Kirby M., Xanthakos S.A., Softic S., Feldstein A.E., Saxena V., Tang P.H., Miles L., Miles M.V., Balistreri W.F., Woods S.C., Seeley R.J. High-fructose, medium chain trans fat diet induces liver fibrosis and elevates plasma coenzyme Q9 in a novel murine model of obesity and nonalcoholic steatohepatitis. Hepatology 2010; 52(3): 934–944, https://doi.org/10.1002/hep.23797.
- Charlton M., Krishnan A., Viker K., Sanderson S., Cazanave S., McConico A., Masuoko H., Gores G. Fast food diet mouse: novel small animal model of NASH with ballooning, progressive fibrosis, and high physiological fidelity to the human condition. Am J Physiol Gastrointest Liver Physiology 2011; 301(5): G825–G834, https://doi.org/10.1152/ajpgi.00145.2011.
- Tsuchiya H., Ebata Y., Sakabe T., Hama S., Kogure K., Shiota G. High-fat, high-fructose diet induces hepatic iron overload via a hepcidin-independent mechanism prior to the onset of liver steatosis and insulin resistance in mice. Metabolism 2013; 62(1): 62–69, https://doi.org/10.1016/j.metabol.2012.06.008.
- Ramboer E., Vanhaecke T., Rogiers V., Vinken M. Immortalized human hepatic cell lines for in vitro testing and research purposes. Methods Mol Biol 2015; 1250: 53–76, https://doi.org/10.1007/978-1-4939-2074-7_4.
- Soret P.A., Magusto J., Housset C., Gautheron J. In vitro and in vivo models of non-alcoholic fatty liver disease: a critical appraisal. J Clin Med 2021; 10(1): 36, https://doi.org/10.3390/jcm10010036.
- Schyschka L., Martínez Sánchez J.J., Wang Z., Burkhardt B., Müller-Vieira U., Zeilinger K., Bachmann A., Nadalin S., Damm G., Nussler A.K. Hepatic 3D cultures but not 2D cultures preserve specific transporter activity for acetaminophen-induced hepatotoxicity. Arch Toxicol 2013; 87(8): 1581–1593, https://doi.org/10.1007/s00204-013-1080-y.
- Sathaye S., Bagul Y., Gupta S., Kaur H., Redkar R. Hepatoprotective effects of aqueous leaf extract and crude isolates of Murraya koenigii against in vitro ethanol-induced hepatotoxicity model. Exp Toxicol Pathol 2011; 63(6): 587–591, https://doi.org/10.1016/j.etp.2010.04.012.
- Lee J., Choi B., No da Y., Lee G., Lee S.R., Oh H., Lee S.H. A 3D alcoholic liver disease model on a chip. Integr Biol (Camb) 2016; 8(3): 302–308, https://doi.org/10.1039/c5ib00298b.
- Deng J., Chen Z., Zhang X., Luo Y., Wu Z., Lu Y., Liu T., Zhao W., Lin B. A liver-chip-based alcoholic liver disease model featuring multi-non-parenchymal cells. Biomed Microdevices 2019; 21(3): 57, https://doi.org/10.1007/s10544-019-0414-9.
- Corrado B., De Gregorio V., Imparato G., Attanasio C., Urciuolo F., Netti P.A. A three-dimensional microfluidized liver system to assess hepatic drug metabolism and hepatotoxicity. Biotechnol Bioeng 2019; 116(5): 1152–1163, https://doi.org/10.1002/bit.26902.
- Jang K.J., Otieno M.A., Ronxhi J., Lim H.K., Ewart L., Kodella K.R., Petropolis D.B., Kulkarni G., Rubins J.E., Conegliano D., Nawroth J., Simic D., Lam W., Singer M., Barale E., Singh B., Sonee M., Streeter A.J., Manthey C., Jones B., Srivastava A., Andersson L.C., Williams D., Park H., Barrile R., Sliz J., Herland A., Haney S., Karalis K., Ingber D.E., Hamilton G.A. Reproducing human and cross-species drug toxicities using a Liver-Chip. Sci Transl Med 2019; 11(517): eaax5516.
- Poli G., Gravela E. Lipid peroxidation in isolated hepatocytes. In: Free radicals, lipid peroxidation and cancer. McBrien D.C.H., Slater T.F. (editors). Academic Press; 1982; p. 215–224.
- Boll M., Weber L.W., Becker E., Stampfl A. Mechanism of carbon tetrachloride-induced hepatotoxicity. Hepatocellular damage by reactive carbon tetrachloride metabolites. Z Naturforsch C J Biosci 2001; 56(7–8): 649–659, https://doi.org/10.1515/znc-2001-7-826.
- Perrissoud D., Auderset G., Reymond O., Maignan M.F. The effect of carbon tetrachloride on isolated rat hepatocytes. Virchows Arch B Cell Pathol Incl Mol Pathol 1981; 35(2): 83–91, https://doi.org/10.1007/bf02889151.
- Cai Y., Gong L.K., Qi X.M., Li X.H., Ren J. Apoptosis initiated by carbon tetrachloride in mitochondria of rat primary cultured hepatocytes. Acta Pharmacol Sin 2005; 26(8): 969–975, https://doi.org/10.1111/j.1745-7254.2005.00143.x.
- Jamil I., Symonds A., Lynch S., Alalami O., Smyth M., Martin J. Divergent effects of paracetamol on reactive oxygen intermediate and reactive nitrogen intermediate production by U937 cells. Int J Mol Med 1999; 4(3): 309–321, https://doi.org/10.3892/ijmm.4.3.309.
- Perez M.J., Gonzalez-Sanchez E., Gonzalez-Loyola A., Gonzalez-Buitrago J.M., Marin J.J.G. Mitochondrial genome depletion dysregulates bile acid- and paracetamol-induced expression of the transporters Mdr1, Mrp1 and Mrp4 in liver cells. Br J Pharmacol 2011; 162(8): 1686–1699, https://doi.org/10.1111/j.1476-5381.2010.01174.x.
- Simeonova R., Vitcheva V., Kondeva-Burdina M., Krasteva I., Manov V., Mitcheva M. Hepatoprotective and antioxidant effects of saponarin, isolated from Gypsophila trichotoma Wend. on paracetamol-induced liver damage in rats. Biomed Res Int 2013; 2013: 757126, https://doi.org/10.1155/2013/757126.
- Bader A., Petters O., Keller M., Pavlica S. Paracetamol treatment increases telomerase activity in rat embryonic liver cells. Pharmacol Rep 2011; 63(6): 1435–1441, https://doi.org/10.1016/s1734-1140(11)70707-1.
- Zhou X., Cheung C.M., Yang J.M., Or P.M., Lee W.Y., Yeung J.H. Danshen (Salvia miltiorrhiza) water extract inhibits paracetamol-induced toxicity in primary rat hepatocytes via reducing CYP2E1 activity and oxidative stress. J Pharm Pharmacol 2015; 67(7): 980–989, https://doi.org/10.1111/jphp.12381.
- Pierce R.H., Franklin C.C., Campbell J.S., Tonge R.P., Chen W., Fausto N., Bruschi S.A. Cell culture model for acetaminophen-induced hepatocyte death in vivo. Biochem Pharmacol 2002; 64(3): 413–424, https://doi.org/10.1016/s0006-2952(02)01180-2.
- Clarke G.A., Hartse B.X., Niaraki Asli A.E., Taghavimehr M., Hashemi N., Abbasi Shirsavar M., Montazami R., Alimoradi N., Nasirian V., Ouedraogo L.J. Hashemi N.N. Advancement of sensor integrated organ-on-chip devices. Sensors (Basel) 2021; 21(4): 1367, https://doi.org/10.3390/s21041367.
- Foster A.J., Chouhan B., Regan S.L., Rollison H., Amberntsson S., Andersson L.C., Srivastava A., Darnell M., Cairns J., Lazic S.E., Jang K.J., Petropolis D.B., Kodella K., Rubins J.E., Williams D., Hamilton G.A., Ewart L., Morgan P. Integrated in vitro models for hepatic safety and metabolism: evaluation of a human Liver-Chip and liver spheroid. Arch Toxicol 2019; 93(4): 1021–1037, https://doi.org/10.1007/s00204-019-02427-4.
- Rodimova S., Elagin V., Karabut M., Koryakina I., Timin A., Zagainov V., Zyuzin M., Zagaynova E., Kuznetsova D. Toxicological analysis of hepatocytes using FLIM technique: in vitro versus ex vivo models. Cells 2021; 10(11): 2894, https://doi.org/10.3390/cells10112894.
- Prot J.M., Bunescu A., Elena-Herrmann B., Aninat C., Snouber L.C., Griscom L., Razan F., Bois F.Y., Legallais C., Brochot C., Corlu A., Dumas M.E., Leclerc E. Predictive toxicology using systemic biology and liver microfluidic “on chip” approaches: application to acetaminophen injury. Toxicol Appl Pharmacol 2012; 259(3): 270–280, https://doi.org/10.1016/j.taap.2011.12.017.
- Gall W.E., Beebe K., Lawton K.A., Adam K.P., Mitchell M.W., Nakhle P.J., Ryals J.A., Milburn M.V., Nannipieri M., Camastra S., Natali A., Ferrannini E.; RISC Study Group. α-Hydroxybutyrate is an early biomarker of insulin resistance and glucose intolerance in a nondiabetic population. PLoS One 2010; 5(5): e10883, https://doi.org/10.1371/journal.pone.0010883.
- Beger R.D., Sun J., Schnackenberg L.K. Metabolomics approaches for discovering biomarkers of drug-induced hepatotoxicity and nephrotoxicity. Toxicol Applied Pharmacol 2010; 243(2): 154–166, https://doi.org/10.1016/j.taap.2009.11.019.
- Soga T., Baran R., Suematsu M., Ueno Y., Ikeda S., Sakurakawa T., Kakazu Y., Ishikawa T., Robert M., Nishioka T., Tomita M. Differential metabolomics reveals ophthalmic acid as an oxidative stress biomarker indicating hepatic glutathione consumption. J Biol Chem 2006; 281(24): 16768–16776, https://doi.org/10.1074/jbc.m601876200.
- Aoudjehane L., Gautheron J., Le Goff W., Goumard C., Gilaizeau J., Nget C.S., Savier E., Atif M., Lesnik P., Morichon R., Chrétien Y., Calmus Y., Scatton O., Housset C., Conti F. Novel defatting strategies reduce lipid accumulation in primary human culture models of liver steatosis. Dis Model Mech 2020; 13(4): dmm042663, https://doi.org/10.1242/dmm.042663.
- Wang L., Chen J., Ning C., Lei D., Ren J. Endoplasmic reticulum stress related molecular mechanisms in nonalcoholic fatty liver disease (NAFLD). Curr Drug Targets 2018; 19(9): 1087–1094, https://doi.org/10.2174/1389450118666180516122517.
- Lin Y., Ding D., Huang Q., Liu Q., Lu H., Lu Y., Chi Y., Sun X., Ye G., Zhu H., Wei J., Dong S. Downregulation of MiR-192 causes hepatic steatosis and lipid accumulation by inducing SREBF1: novel mechanism for bisphenol A-triggered non-alcoholic fatty liver disease. Biochim Biophys Acta Mol Cell Biol Lipids 2017; 1862(9): 869–882, https://doi.org/10.1016/j.bbalip.2017.05.001.
- Ling J., Lewis J., Douglas D., Kneteman N.M., Vance D.E. Characterization of lipid and lipoprotein metabolism in primary human hepatocytes. Biochim Biophys Acta 2013; 1831(2): 387–397, https://doi.org/10.1016/j.bbalip.2012.08.012.
- Barbero-Becerra V.J., Giraudi P.J., Chávez-Tapia N.C., Uribe M., Tiribelli C., Rosso N. The interplay between hepatic stellate cells and hepatocytes in an in vitro model of NASH. Toxicol in Vitro 2015; 29(7): 1753–1758, https://doi.org/10.1016/j.tiv.2015.07.010.
- Suurmond C.E., Lasli S., van den Dolder F.W., Ung A., Kim H.J., Bandaru P., Lee K., Cho H.J., Ahadian S., Ashammakhi N., Dokmeci M.R., Lee J., Khademhosseini A. In vitro human liver model of nonalcoholic steatohepatitis by coculturing hepatocytes, endothelial cells, and kupffer cells. Adv Healthc Mater 2019; 8(24): e1901379, https://doi.org/10.1002/adhm.201901379.
- Giraudi P.J., Becerra V.J., Marin V., Chavez-Tapia N.C., Tiribelli C., Rosso N. The importance of the interaction between hepatocyte and hepatic stellate cells in fibrogenesis induced by fatty accumulation. Exp Mol Pathol 2015; 98(1): 85–92, https://doi.org/10.1016/j.yexmp.2014.12.006.
- Feaver R.E., Cole B.K., Lawson M.J., Hoang S.A., Marukian S., Blackman B.R., Figler R.A., Sanyal A.J., Wamhoff B.R., Dash A. Development of an in vitro human liver system for interrogating non-alcoholic steatohepatitis. JCI Insight 2016; 1(20): e90954, https://doi.org/10.1172/jci.insight.90954.
- Chen Y., Ma K. NLRC4 inflammasome activation regulated by TNF-α promotes inflammatory responses in non-alcoholic fatty liver disease. Biochem Biophys Res Commun 2019; 511(3): 524–530, https://doi.org/10.1016/j.bbrc.2019.02.099.
- Müller F.A., Sturla S.J. Human in vitro models of nonalcoholic fatty liver disease. Curr Opin Toxicol 2019; 16: 9–16, https://doi.org/10.1016/j.cotox.2019.03.001.
- Gori M., Simonelli M.C., Giannitelli S.M., Businaro L., Trombetta M., Rainer A. Investigating nonalcoholic fatty liver disease in a liver-on-a-chip microfluidic device. PLoS One 2016; 11(7): e0159729, https://doi.org/10.1371/journal.pone.0159729.
- Banaeiyan A.A., Theobald J., Paukštyte J., Wölfl S., Adiels C.B., Goksör M. Design and fabrication of a scalable liver-lobule-on-a-chip microphysiological platform. Biofabrication 2017; 9(1): 015014, https://doi.org/10.1088/1758-5090/9/1/015014.
- Hassan S., Sebastian S., Maharjan S., Lesha A., Carpenter A.M., Liu X., Xie X., Livermore C., Zhang Y.S., Zarrinpar A. Liver-on-a-chip models of fatty liver disease. Hepatology 2020; 71(2): 733–740, https://doi.org/10.1002/hep.31106.
- Kostrzewski T., Cornforth T., Snow S.A., Ouro-Gnao L., Rowe C., Large E.M., Hughes D.J. Three-dimensional perfused human in vitro model of non-alcoholic fatty liver disease. World J Gastroenterol 2017; 23(2): 204–215, https://doi.org/10.3748/wjg.v23.i2.204.
- Braunersreuther V., Viviani G.L., Mach F., Montecucco F. Role of cytokines and chemokines in non-alcoholic fatty liver disease. World J Gastroenterol 2012; 18(8): 727–735, https://doi.org/10.3748/wjg.v18.i8.727.
- Lee S.Y., Sung J.H. Gut–liver on a chip toward an in vitro model of hepatic steatosis. Biotechnol Bioeng 2018; 115(11): 2817–2827, https://doi.org/10.1002/bit.26793.
- Freag M.S., Namgung B., Reyna Fernandez M.E., Gherardi E., Sengupta S., Jang H.L. Human nonalcoholic steatohepatitis on a chip. Hepatol Commun 2020; 5(2): 217–233, https://doi.org/10.1002/hep4.1647.
- Kleiner D.E., Brunt E.M., Van Natta M., Behling C., Contos M.J., Cummings O.W., Ferrell L.D., Liu Y.C., Torbenson M.S., Unalp-Arida A., Yeh M., McCullough A.J., Sanyal A.J.; Nonalcoholic Steatohepatitis Clinical Research Network. Design and validation of a histological scoring system for nonalcoholic fatty liver disease. Hepatology 2005; 41(6): 1313–1321, https://doi.org/10.1002/hep.20701.
- Bracken M.B. Why animal studies are often poor predictors of human reactions to exposure. J R Soc Med 2009; 102(3): 120–122, https://doi.org/10.1258/jrsm.2008.08k033.
- Wu X., Roberto J.B., Knupp A., Kenerson H.L., Truong C.D., Yuen S.Y., Brempelis K.J., Tuefferd M., Chen A., Horton H., Yeung R.S., Crispe I.N. Precision-cut human liver slice cultures as an immunological platform. J Immunol Methods 2018; 455: 71–79, https://doi.org/10.1016/j.jim.2018.01.012.
- Fisher R.L., Vickers A.E. Preparation and culture of precision-cut organ slices from human and animal. Xenobiotica 2013; 43(1): 8–14, https://doi.org/10.3109/00498254.2012.728013.
- De Graaf I.A.M., Olinga P., de Jager M.H., Merema M.T., de Kanter R., van de Kerkhof E.G., Groothuis G.M. Preparation and incubation of precision-cut liver and intestinal slices for application in drug metabolism and toxicity studies. Nat Protoc 2010; 5(9): 1540–1551, https://doi.org/10.1038/nprot.2010.111.
- Klassen L.W., Thiele G.M., Duryee M.J., Schaffert C.S., DeVeney A.L., Hunter C.D., Olinga P., Tuma D.J. An in vitro method of alcoholic liver injury using precision cut liver slices from rats. Biochem Pharmacol 2008; 76(3): 426–436, https://doi.org/10.1016/j.bcp.2008.05.012.
- Schaffert C.S., Todero S.L., McVicker B.L., Tuma P.L., Sorrell M.F., Tuma D.J. WIF-B cells as a model for alcohol-induced hepatocyte injury. Biochem Pharmacol 2004; 67(11): 2167–2674, https://doi.org/10.1016/j.bcp.2004.01.022.
- Bruguera M., Bertran A., Bombi J. A., Rodes J. Giant mitochondria in hepatocytes: a diagnostic hint for alcoholic liver disease. Gastroenterology 1977; 73(6): 1383–1387, https://doi.org/10.1016/s0016-5085(19)31518-5.
- Teli M.R., Day C.P., Burt A.D., Bennett M.K., James O.F. Determinants of progression to cirrhosis or fibrosis in pure alcoholic fatty liver. Lancet 1995; 346(8981): 987–990, https://doi.org/10.1016/S0140-6736(95)91685-7.
- Palma E., Riva A., Mudan S., Manyakin N., Morrison D., Moreno C., Degré D., Trepo E., Sancho-Bru P., Altamirano J., Caballeria J., Odena G., Bataller R., Williams R., Chokshi S. Perturbation of mitochondrial dynamics in alcoholic liver disease. J World Mitochondria Soc 2016; 2(2): 1–4, https://doi.org/10.18143/jwms_v2i2_1948.
- Palma E., Riva A., Moreno C., Odena G., Mudan S., Manyakin N., Manyakin N., Miquel R., Degré D., Trepo E., Sancho-Bru P., Altamirano J., Caballeria J., Zamalloa A., Menon K., Heaton N., Williams R., Bataller R., Chokshi S. Perturbations in mitochondrial dynamics are closely involved in the progression of alcoholic liver disease. Alcohol Clin Exp Res 2020; 44(4): 856–865, https://doi.org/10.1111/acer.14299.
- Li F., Zhou J., Li Y., Sun K., Chen J. Mitochondrial damage and Drp1 overexpression in rifampicin- and isoniazid-induced liver injury cell model. J Clin Transl Hepatol 2019; 7(1): 40–45, https://doi.org/10.14218/jcth.2018.00052.
- Granitzny A., Knebel J., Schaudien D., Braun A., Steinberg P., Dasenbrock C., Hansen T. Maintenance of high quality rat precision cut liver slices during culture to study hepatotoxic responses: acetaminophen as a model compound. Toxicol in Vitro 2017; 42: 200–213, https://doi.org/10.1016/j.tiv.2017.05.001.
- Ji J., Yu F., Ji Q., Li Z., Wang K., Zhang J., Lu J., Chen L., E Q., Zeng Y., Ji Y., Qun E. Comparative proteomic analysis of rat hepatic stellate cell activation: a comprehensive view and suppressed immune response. Hepatology 2012; 56(1): 332–349, https://doi.org/10.1002/hep.25650.
- Li J.T., Wang H., Pan X.L., Yan Y.E., Guo Y., Zhang B.J. Establishment of an acetaminophen-induced hepatotoxicity model of precision-cut liver slices and the regulation of cytochrome P450 2E1. Chin J Clin Pharmacol Ther 2008; 13(1): 46.
- McGill M.R., Williams C.D., Xie Y., Ramachandran A., Jaeschke H. Acetaminophen-induced liver injury in rats and mice: comparison of protein adducts, mitochondrial dysfunction, and oxidative stress in the mechanism of toxicity. Toxicol Appl Pharmacol 2012; 264(3): 387–394, https://doi.org/10.1016/j.taap.2012.08.015.
- Dewyse L., De Smet V., Verhulst S., Eysackers N., Kunda R., Messaoudi N., Reynaert H., van Grunsven L.A. Improved precision-cut liver slice cultures for testing drug-induced liver fibrosis. Front Med (Lausanne) 2022; 9:862185, https://doi.org/10.3389/fmed.2022.862185.
- Schreiter T., Sowa J.P., Schlattjan M., Treckmann J., Paul A., Strucksberg K.H., Baba H.A., Odenthal M., Gieseler R.K., Gerken G., Arteel G.E., Canbay A. Human ex-vivo liver model for acetaminophen-induced liver damage. Sci Rep 2016; 6(1): 31916, https://doi.org/10.1038/srep31916.
- Kanani T., Isherwood J., Issa E., Chung W.Y., Ravaioli M., Oggioni M.R., Garcea G., Dennison A. A narrative review of the applications of ex-vivo human liver perfusion. Cureus 2023; 15(2): e34804, https://doi.org/10.7759/cureus.34804.
- Van de Bovenkamp M., Groothuis G.M., Draaisma A.L., Merema M.T., Bezuijen J.I., van Gils M.J., Meijer D.K.F., Friedman S.L., Olinga P. Precision-cut liver slices as a new model to study toxicity-induced hepatic stellate cell activation in a physiologic milieu. Toxicol Sci 2005; 85(1): 632–638, https://doi.org/10.1093/toxsci/kfi127.
- Matsuzaki K. Smad phosphoisoform signals in acute and chronic liver injury: similarities and differences between epithelial and mesenchymal cells. Cell Tissue Res 2012; 347(1): 225–243, https://doi.org/10.1007/s00441-011-1178-6.
- Green C.J., Parry S.A., Gunn P.J., Ceresa C.D.L., Rosqvist F., Piché M.E., Hodson L. Studying non-alcoholic fatty liver disease: the ins and outs of in vivo, ex vivo and in vitro human models. Horm Mol Biol Clin Investig 2020; 41(1): 20180038, https://doi.org/10.1515/hmbci-2018-0038.
- Graulet B., Gruffat D., Durand D., Bauchart D. Fatty acid metabolism and very low density lipoprotein secretion in liver slices from rats and preruminant calves. J Biochem 1998; 124(6): 1212–1219, https://doi.org/10.1093/oxfordjournals.jbchem.a022240.
- Pullen D.L., Liesman J.S., Emery R.S. A species comparison of liver slice synthesis and secretion of triacylglycerol from nonesterified fatty acids in media. J Anim Sci 1990; 68: 1395–1399, https://doi.org/10.2527/1990.6851395x.
- Alam S., Mustafa G., Alam M., Ahmad N. Insulin resistance in development and progression of nonalcoholic fatty liver disease. World J Gastrointest Pathophysiol 2016; 7(2): 211–217, https://doi.org/10.4291/wjgp.v7.i2.211.
- Prins G.H., Luangmonkong T., Oosterhuis D., Mutsaers H.A.M., Dekker F.J., Olinga P. A pathophysiological model of non-alcoholic fatty liver disease using precision-cut liver slices. Nutrients 2019; 11(3): 507, https://doi.org/10.3390/nu11030507.
- Ly L.D., Xu S., Choi S.K., Ha C.M., Thoudam T., Cha S.K., Wiederkehr A., Wollheim C.B., Lee I.K., Park K.S. Oxidative stress and calcium dysregulation by palmitate in type 2 diabetes. Exp Mol Med 2017; 49(2): e291, https://doi.org/10.1038/emm.2016.157.
- Prins G.H., Rios-Morales M., Gerding A., Reijngoud D.J., Olinga P., Bakker B.M. The effects of butyrate on induced metabolic-associated fatty liver disease in precision-cut liver slices. Nutrients 2021; 13(12): 4203, https://doi.org/10.3390/nu13124203.
- Lake B.G., Price R.J. Evaluation of the metabolism and hepatotoxicity of xenobiotics utilizing precision-cut slices. Xenobiotica 2012; 43(1): 41–53, https://doi.org/10.3109/00498254.2012.734643.