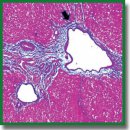
Клеточно-молекулярные механизмы токсического фиброза печени крыс в зависимости от стадий его развития
Цель исследования — изучить клеточно-молекулярные особенности токсического фиброза печени крыс в зависимости от стадий развития этого патологического состояния.
Материалы и методы. Фиброгенез печени у крыс-самцов линии Wistar индуцировали раствором тиоацетамида путем введения в желудок с помощью зонда в дозе 200 мг/кг массы тела животного 2 раза в неделю. Динамику процесса изучали в 5 временны́х точках (контроль, 3, 5, 7 и 9 нед). Уровень мРНК генов tweak,fn14, ang, vegfa, cxcl12, mmp-9 в печени выявляли методом полимеразной цепной реакции в режиме реального времени. Иммуногистохимическое исследование проводили на парафиновых срезах. В качестве маркеров использовали клетки CD31, CD34, СК19, α-SMA, FAP, CD68, CD206, СХ3СR1, CD45. Степень фиброза определяли в гистологических срезах, окрашенных по методу Маллори, согласно полуколичественной шкале K.G. Ishak.
Результаты. В печени крыс одновременно выявили две морфологически неоднородные популяции миофибробластов, экспрессирующие различные типы маркеров (FAP, α-SMA). До начала процесса трансформации фиброза в цирроз (F1–F4, 3–7 нед) клетки FAP+ и SMA+ локализовались в разных местах гистологических препаратов. Все стадии прогрессирования фиброза печени сопровождались увеличением количества (р=0,0000), изменением фенотипического состава и функциональных свойств макрофагов. Клетки СК19+ портальных зон дифференцировались в холангиоциты, формирующие междольковые желчные протоки и проточки, а также гепатоциты, формирующие зачатки новых печеночных микродолек. Установлены патологический венозный ангиогенез и гетерогенность эндотелиоцитов внутрипеченочного сосудистого русла. Определены два варианта изменения экспрессии мРНК выбранных генов. Уровень мРНК fn14 и mmp-9 на всех стадиях фиброза был выше (р=0,0000), чем у крыс контрольной группы. В отношении мРНК tweak, ang, vegfa, cxcl12 ситуация была противоположной — уровень генов упал (р=0,0000). Между исследуемыми генами-мишенями отмечены сильные и умеренные корреляции (р<0,05).
Заключение. Установлено, что стадии токсического фиброза имеют морфологические и молекулярно-генетические особенности. Клетки FAP+ вносят основной вклад в развитие портального и начального этапа мостовидного фиброза. В перспективе звездчатые макрофаги и инфильтрирующие моноциты/макрофаги могут быть использованы при разработке новых терапевтических стратегий для лечения патологии печени. При исследовании внутрипеченочного сосудистого русла необходимо учитывать особенности экспрессии маркеров эндотелиоцитами. Совместное относительно друг друга изучение генов является необходимым дополнительным параметром при проведении фундаментальных и доклинических исследований.
- Asrani S.K., Devarbhavi H., Eaton J., Kamath P.S. Burden of liver diseases in the world. J Hepatol 2019; 70(1): 151–171, https://doi.org/10.1016/j.jhep.2018.09.014.
- Roehlen N., Crouchet E., Baumert T.F. Liver fibrosis: mechanistic concepts and therapeutic perspectives. Cells 2020; 9(4): 875, https://doi.org/10.3390/cells9040875.
- Xu M., Xu H.H., Lin Y., Sun X., Wang L.J., Fang Z.P., Su X.H., Liang X.J., Hu Y., Liu Z.M., Cheng Y., Wei Y., Li J., Li L., Liu H.J., Cheng Z., Tang N., Peng C., Li T., Liu T., Qiao L., Wu D., Ding Y.Q., Zhou W.J. LECT2, a ligand for Tie1, plays a crucial role in liver fibrogenesis. Cell 2019; 178(6): 1478–1492.e20, https://doi.org/10.1016/j.cell.2019.07.021.
- Friedman S.L., Pinzani M. Hepatic fibrosis 2022: unmet needs and a blueprint for the future. Hepatology 2022; 75(2): 473–488, https://doi.org/10.1002/hep.32285.
- Lin Y., Dong M.Q., Liu Z.M., Xu M., Huang Z.H., Liu H.J., Gao Y., Zhou W.J. A strategy of vascular-targeted therapy for liver fibrosis. J Hepatology 2022; 76(3): 660–675, https://doi.org/10.1002/hep.32299.
- Zhang D., Zhang Y., Sun B. The molecular mechanisms of liver fibrosis and its potential therapy in application. Int J Mol Sci 2022; 23(20): 12572, https://doi.org/10.3390/ijms232012572.
- Borrello M.T., Mann D. Chronic liver diseases: from development to novel pharmacological therapies: IUPHAR review 37. Br J Pharmacol 2022, https://doi.org/10.1111/bph.15853.
- Jangra A., Kothari A., Sarma P., Medhi B., Omar B.J., Kaushal K. Recent advancements in antifibrotic therapies for regression of liver fibrosis. Cells 2022; 11(9): 1500, https://doi.org/10.3390/cells11091500.
- Li Y., Yuan S.L., Yin J.Y., Yang K., Zhou X.G., Xie W., Wang Q. Differences of core genes in liver fibrosis and hepatocellular carcinoma: evidence from integrated bioinformatics and immunohistochemical analysis. World J Gastrointest Oncol 2022; 14(7): 1265–1280, https://doi.org/10.4251/wjgo.v14.i7.1265.
- Antar S.A., Ashour N.A., Marawan M.E., Al-Karmalawy A.A. Fibrosis: types, effects, markers, mechanisms for disease progression, and its relation with oxidative stress, immunity, and inflammation. Int J Mol Sci 2023; 24(4): 4004, https://doi.org/10.3390/ijms24044004.
- Parola M., Pinzani M. Liver fibrosis: pathophysiology, pathogenetic targets and clinical issues. Mol Aspects Med 2019; 65: 37–55, https://doi.org/10.1016/j.mam.2018.09.002.
- Li H. Angiogenesis in the progression from liver fibrosis to cirrhosis and hepatocelluar carcinoma. Expert Rev Gastroenterol Hepatol 2021; 15(3): 217–233, https://doi.org/10.1080/17474124.2021.1842732.
- Yang L., Yue W., Zhang H., Zhang Z., Xue R., Dong C., Liu F., Chang N., Yang L., Li L. Dual targeting of Angipoietin-1 and von Willebrand factor by microRNA-671-5p attenuates liver angiogenesis and fibrosis. Hepatol Commun 2022; 6(6): 1425–1442, https://doi.org/10.1002/hep4.1888.
- Wallace S.J., Tacke F., Schwabe R.F., Henderson N.C. Understanding the cellular interactome of non-alcoholic fatty liver disease. JHEP Rep 2022; 4(8): 100524, https://doi.org/10.1016/j.jhepr.2022.100524.
- Quintero-Fabián S., Arreola R., Becerril-Villanueva E., Torres-Romero J.C., Arana-Argáez V., Lara-Riegos J., Ramírez-Camacho M.A., Alvarez-Sánchez M.E. Role of matrix metalloproteinases in angiogenesis and cancer. Front Oncol 2019; 9: 1370, https://doi.org/10.3389/fonc.2019.01370.
- Hoeben A., Landuyt B., Highley M.S., Wildiers H., Van Oosterom A.T., De Bruijn E.A. Vascular endothelial growth factor and angiogenesis. Pharmacol Rev 2004; 56(4): 549–580, https://doi.org/10.1124/pr.56.4.3.
- Jones M.L., Ewing C.M., Isaacsa W.B., Getzenberg R.H. Prostate cancer-derived angiogenin stimulates the invasion of prostate fibroblasts. J Cell Mol Med 2012; 16(1): 193–201, https://doi.org/10.1111/j.1582-4934.2011.01283.x.
- Wang M., Xie Z., Xu J., Feng Z. TWEAK/Fn14 axis in respiratory diseases. Clin Chim Acta 2020; 509: 139–148, https://doi.org/10.1016/j.cca.2020.06.007.
- Dwyer B.J., Jarman E.J., Gogoi-Tiwari J., Ferreira-Gonzalez S., Boulter L., Guest R.V., Kendall T.J., Kurian D., Kilpatrick A.M., Robson A.J., O’Duibhir E., Man T.Y., Campana L., Starkey Lewis P.J., Wigmore S.J., Olynyk J.K., Ramm G.A., Tirnitz-Parker J.E.E., Forbes S.J. TWEAK/Fn14 signalling promotes cholangiocarcinoma niche formation and progression. J Hepatol 2021; 74(4): 860–872, https://doi.org/10.1016/j.jhep.2020.11.018.
- Short C., Zhong A., Xu J., Mahdi E., Glazier A., Malkoff N., Noriega N., Yeo T., Asahina K., Wang K.S. TWEAK/FN14 promotes profibrogenic pathway activation in Prominin-1-expressing hepatic progenitor cells in biliary atresia. Hepatology 2023; 77(5): 1639–1653, https://doi.org/10.1097/hep.0000000000000026.
- Dhar D., Baglieri J., Kisseleva T., Brenner D.A. Mechanisms of liver fibrosis and its role in liver cancer. Exp Biol Med (Maywood) 2020; 245(2): 96–108, https://doi.org/10.1177/1535370219898141.
- Luo N., Li J., Wei Y., Lu J., Dong R. Hepatic stellate cell: a double-edged sword in the liver. Physiol Res 2021; 70(6): 821–829, https://doi.org/10.33549/physiolres.934755.
- Cheng D., Chai J., Wang H., Fu L., Peng S., Ni X. Hepatic macrophages: key players in the development and progression of liver fibrosis. Liver Int 2021; 41(10): 2279–2294, https://doi.org/10.1111/liv.14940.
- Wang C., Ma C., Gong L., Guo Y., Fu K., Zhang Y., Zhou H., Li Y. Macrophage polarization and its role in liver disease. Front Immunol 2021; 12: 803037, https://doi.org/10.3389/fimmu.2021.803037.
- Lafoz E., Ruart M., Anton A., Oncins A., Hernández-Gea V. The endothelium as a driver of liver fibrosis and regeneration. Cells 2020; 9(4): 929, https://doi.org/10.3390/cells9040929.
- Zadorozhna M., Di Gioia S., Conese M., Mangieri D. Neovascularization is a key feature of liver fibrosis progression: anti-angiogenesis as an innovative way of liver fibrosis treatment. Mol Biol Rep 2020; 47(3): 2279–2288, https://doi.org/10.1007/s11033-020-05290-0.
- Kumar S., Duan Q., Wu R., Harris E.N., Su Q. Pathophysiological communication between hepatocytes and non-parenchymal cells in liver injury from NAFLD to liver fibrosis. Adv Drug Deliv Rev 2021; 176: 113869, https://doi.org/10.1016/j.addr.2021.113869.
- Банин В.В., Белоусова Т.А., Быков В.Л. Terminologia Histologica. Международные термины по цитологии и гистологии человека с официальным списком русских эквивалентов: справочное пособие. Под ред. Банина В.В., Быкова В.Л. М: ГЭОТАР-Медиа; 2009; 272 с.
- Muthiah M.D., Huang D.Q., Zhou L., Jumat N.H., Choolani M., Chan J.K.Y., Wee A., Lim S.G., Dan Y.Y. A murine model demonstrating reversal of structural and functional correlates of cirrhosis with progenitor cell transplantation. Sci Rep 2019; 9(1): 15446, https://doi.org/10.1038/s41598-019-51189-7.
- Теоретические основы и практическое применение методов иммуногистохимии. Под ред. Коржевского Д.Э. СПб: СпецЛит; 2014; 119 с.
- Zheng C., Luo J., Yang Y., Dong R., Yu F.X., Zheng S. YAP activation and implications in patients and a mouse model of biliary atresia. Front Pediatr 2021; 8: 618226, https://doi.org/10.3389/fped.2020.618226.
- Everhart J.E., Wright E.C., Goodman Z.D., Dienstag J.L., Hoefs J.C., Kleiner D.E., Ghany M.G., Mills A.S., Nash S.R., Govindarajan S., Rogers T.E., Greenson J.K., Brunt E.M., Bonkovsky H.L., Morishima C., Litman H.J.; HALT-C Trial Group. Prognostic value of Ishak fibrosis stage: findings from the hepatitis C antiviral long-term treatment against cirrhosis trial. Hepatology 2010; 51(2): 585–594, https://doi.org/10.1002/hep.23315.
- Лебедева Е.И., Бабенко А.С., Щастный А.Т. Динамика стабильности экспрессии генов sdha, hprt, prl3d1 и hes1 в рамках моделирования фиброза печени крыс. Биомедицина 2022; 18(2): 17–30, https://doi.org/10.33647/2074-5982-18-2-17-30.
- Younis N.S., Ghanim A.M.H., Elmorsy M.A., Metwaly H. Taurine ameliorates thioacetamide induced liver fibrosis in rats via modulation of toll like receptor 4/nuclear factor kappa B signaling pathway. Sci Rep 2021; 11(1): 12296, https://doi.org/10.1038/s41598-021-91666-6.
- Wu Y., Li Z., Xiu A.Y. Meng D.X., Wang S.N., Zhang C.Q. Carvedilol attenuates carbon tetrachloride-induced liver fibrosis and hepatic sinusoidal capillarization in mice. Drug Des Devel Ther 2019; 13: 2667–2676, https://doi.org/10.2147/dddt.s210797.
- Yang A.T., Kim Y.O., Yan X.Z., Abe H., Aslam M., Park K.S., Zhao X.Y., Jia J.D., Klein T., You H., Schuppan D. Fibroblast activation protein activates macrophages and promotes parenchymal liver inflammation and fibrosis. Cell Mol Gastroenterol Hepatol 2023; 15(4): 841–867, https://doi.org/10.1016/j.jcmgh.2022.12.005.
- Fuji H., Miller G., Nishio T., Koyama Y., Lam K., Zhang V., Loomba R., Brenner D., Kisseleva T. The role of mesothelin signaling in portal fibroblasts in the pathogenesis of cholestatic liver fibrosis. Front Mol Biosci 2021; 8: 790032, https://doi.org/10.3389/fmolb.2021.790032.
- Dong X., Liu J., Xu Y., Cao H. Role of macrophages in experimental liver injury and repair in mice. Exp Ther Med 2019; 17(5): 3835–3847, https://doi.org/10.3892/etm.2019.7450.
- Kamimoto K., Nakano Y., Kaneko K., Miyajima A., Itoh T. Multidimensional imaging of liver injury repair in mice reveals fundamental role of the ductular reaction. Commun Biol 2020; 3(1): 289, https://doi.org/10.1038/s42003-020-1006-1.
- Sato K., Marzioni M., Meng F., Francis H., Glaser S., Alpini G. Ductular reaction in liver diseases: pathological mechanisms and translational significances. Hepatology 2019; 69(1): 420–430, https://doi.org/10.1002/hep.30150.
- Augustin H.G., Koh G.Y. Organotypic vasculature: from descriptive heterogeneity to functional pathophysiology. Science 2017; 357(6353): eaal2379, https://doi.org/10.1126/science.aal2379.
- Zhou W., Guo S., Liu M., Burow M.E., Wang G. Targeting CXCL12/CXCR4 axis in tumor immunotherapy. Curr Med Chem 2019; 26(17): 3026–3041, https://doi.org/10.2174/0929867324666170830111531.
- Zhang Y., Zeng W., Xia Y. TWEAK/Fn14 axis is an important player in fibrosis. J Cell Physiol 2021; 236(5): 3304–3316, https://doi.org/10.1002/jcp.30089.