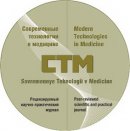
Endothelial Progenitor Cells in Cerebral Endothelium Development and Repair (Review)
The review covers the current concepts on the role of endothelial cells in the blood-brain barrier development and repair. The authors have characterized cerebral endothelial cells within a neurovascular unit, and endothelial progenitor cells, their development and migration to the brain, as well as molecular mechanisms of barriergenesis with a special focus on the modulation of intercellular communications. The review contains the analysis of application of endothelial progenitor cells of different origin in in vitro blood-brain barrier models. HIF-1-controlled regulation mechanisms of the regulation of functional activity of endothelial cells, angiogenesis, astrocyte-endothelial interactions, and cell metabolism within a neurovascular unit has been discussed. The authors have analyzed possible contribution mechanisms of these processes in endothelial dysfunction development as one of the pathogenetic components of neurodevelopmental disorders, ischemic brain injury, or neurodegeneration associated with alterations in key metabolic and transport processes, secretion of regulatory molecules, cell-to-cell communication in cerebral endothelial cells, as well as with endothelial cell death and the loss of structural and functional integrity of the blood-brain barrier. Deciphering the cellular and molecular mechanisms of barriergenesis in brain development, and its recovery after damage will provide new opportunities for pharmacotherapy of central nervous system disorders due to endothelial dysfunction.
- Atkins G.B., Jain M.K., Hamik A. Endothelial differentiation: molecular mechanisms of specification and heterogeneity. Arterioscler Thromb Vasc Biol 2011; 31(7): 1476–1484, http://dx.doi.org/10.1161/ATVBAHA.111.228999.
- Kuvacheva N.V., Salmina A.B., Komleva Yu.K., Malinovskaia N.A., Morgun A.V., Pozhilenkova E.A., Zamai G.S., Iauzina N.A., Petrova M.M. Permeability of the hematoencephalic barrier in normalcy, brain development pathology and neurodegeneration. Zhurnal nevrologii i psikhiatrii im. S.S. Korsakova 2013; 113(4): 80–85.
- Bundgaard M., Abbott N.J. All vertebrates started out with a glial blood-brain barrier 4-500 million years ago. Glia 2008; 56(7): 699–708, http://dx.doi.org/10.1002/glia.20642.
- Daneman R., Zhou L., Kebede A.A., Barres B.A. Pericytes are required for blood-brain barrier integrity during embryogenesis. Nature 2010; 468(7323): 562–566, http://dx.doi.org/10.1038/nature09513.
- Luissint A.C., Artus C., Glacial F., Ganeshamoorthy K., Couraud P.O. Tight junctions at the blood brain barrier: physiological architecture and disease-associated dysregulation. Fluids Barriers CNS 2012; 9(1): 23, http://dx.doi.org/10.1186/2045-8118-9-23.
- Ronaldson P.T., Davis T.P. Blood-brain barrier integrity and glial support: mechanisms that can be targeted for novel therapeutic approaches in stroke. Curr Pharm Des 2012; 18(25): 3624–3644, http://dx.doi.org/10.2174/138161212802002625.
- Morgun A.V., Kuvacheva N.V., Taranushenko T.E., Khilazheva E.D., Malinovskaya N.A., Gorina Ya.V., Pozhilenkova E.A., Frolova O.V., Salmina A.B. Current concepts of perinatal ischemic injury in the brain neurovascular unit: molecular targets for neuroprotection. Vestnik RAMN 2013; 12: 26–35.
- Nico B., Ribatti D. Morphofunctional aspects of the blood-brain barrier. Curr Drug Metab 2012; 13(1): 50–60, http://dx.doi.org/10.2174/138920012798356970.
- Mizee M.R., Wooldrik D., Lakeman K.A., van het Hof B., Drexhage J.A., Geerts D., Bugiani M., Aronica E., Mebius R.E., Prat A., de Vries H.E., Reijerkerk A. Retinoic acid induces blood-brain barrier development. J Neurosci 2013; 33(4): 1660–1671, http://dx.doi.org/10.1523/JNEUROSCI.1338-12.2013.
- Engelhardt B., Liebner S. Novel insights into the development and maintenance of the blood-brain barrier. Cell Tissue Res 2014; 355(3): 687–699, http://dx.doi.org/10.1007/s00441-014-1811-2.
- Salmina A.B., Morgun A.V., Kuvacheva N.V., Komleva Yu.K., Pozhilenkova E.A., Kutishcheva I.A., Trufanova L.V., Taranushenko T.E., Martynova G.P. Cell-cell interactions in the developing blood-brain barrier. Pediatriya 2013; 2: 155–159.
- Siegenthaler J.A., Sohet F., Daneman R. ‘Sealing off the CNS’: cellular and molecular regulation of blood-brain barriergenesis. Curr Opin Neurobiol 2013; 23(6): 1057–1064, http://dx.doi.org/10.1016/j.conb.2013.06.006.
- Risau W., Wolburg H. Development of the blood-brain barrier. Trends Neurosci 1990; 13: 174–178, http://dx.doi.org/10.1016/0166-2236(90)90043-A.
- Wallace C.S., Withers G.S., Farnand A., Lobingier B.T., McCleery E.J. Evidence that angiogenesis lags behind neuron and astrocyte growth in experience-dependent plasticity. Dev Psychobiol 2011; 53(5): 435–442, http://dx.doi.org/10.1002/dev.20559.
- Lu J. A novel hypothesis of blood-brain barrier (BBB) development and in vitro BBB model: neural stem cell is the driver of BBB formation and maintenance. J Exp Integr Med 2012; 2(1): 39–43, http://dx.doi.org/10.5455/jeim.041211.hp.002.
- Lippmann E.S., Azarin S.M., Kay J.E., Nessler R.A., Wilson H.K., Al-Ahmad A., Palecek S.P., Shusta E.V. Derivation of blood-brain barrier endothelial cells from human pluripotent stem cells. Nat Biotechnol 2012; 30(8): 783–791, http://dx.doi.org/10.1038/nbt.2247.
- Timmermans F., Plum J., Yöder M.C., Ingram D.A., Vandekerckhove B., Case J. Endothelial progenitor cells: identity defined? J Cell Mol Med 2009; 13(1): 87–102, http://dx.doi.org/10.1111/j.1582-4934.2008.00598.x.
- Stankovich B.L., Aguayo E., Barragan F., Sharma A., Pallavicini M.G. Differential adhesion molecule expression during murine embryonic stem cell commitment to the hematopoietic and endothelial lineages. PLoS One 2011; 6(9): e23810, http://dx.doi.org/10.1371/journal.pone.0023810.
- Boyer-Di Ponio J., El-Ayoubi F., Glacial F., Ganeshamoorthy K., Driancourt C., Godet M., Perriére N., Guillevic O., Couraud P.O., Uzan G. Instruction of circulating endothelial progenitors in vitro towards specialized blood-brain barrier and arterial phenotypes. PLoS One 2014; 9(1): e84179, http://dx.doi.org/10.1371/journal.pone.0084179.
- Salmina A.B., Morgun A.V., Kuvacheva N.V., Lopatina O.L., Komleva Y.K., Malinovskaya N.A., Pozhilenkova E.A. Establishment of neurogenic microenvironment in the neurovascular unit: the connexin 43 story. Rev Neurosci 2014; 25(1): 97–111, http://dx.doi.org/10.1515/revneuro-2013-0044.
- Gubert F., Zaverucha-do-Valle C., Pimentel-Coelho P.M., Mendez-Otero R., Santiago M.F. Radial glia-like cells persist in the adult rat brain. Brain Res 2009; 1258: 43–52, http://dx.doi.org/10.1016/j.brainres.2008.12.021.
- Bjørnbak C., Brøchner C.B., Larsen L.A., Johansen J.S., Møllgård K. Brain barriers and a subpopulation of astroglial progenitors of developing human forebrain are immunostained for the glycoprotein YKL-40. J Histochem Cytochem 2014; 62(5): 369–388, http://dx.doi.org/10.1369/0022155414528514.
- Craig-Schapiro R., Perrin R.J., Roe C.M., Xiong C., Carter D., Cairns N.J., Mintun M.A., Peskind E.R., Li G., Galasko D.R., Clark C.M., Quinn J.F., D’Angelo G., Malone J.P., Townsend R.R., Morris J.C., Fagan A.M., Holtzman D.M. YKL-40: a novel prognostic fluid biomarker for preclinical Alzheimer’s disease. Biol Psychiatry 2010; 68(10): 903–912, http://dx.doi.org/10.1016/j.biopsych.2010.08.025.
- Bonneh-Barkay D., Wang G., Starkey A., Hamilton R.L., Wiley C.A. In vivo CHI3L1 (YKL-40) expression in astrocytes in acute and chronic neurological diseases. J Neuroinflammation 2010; 7: 34, http://dx.doi.org/10.1186/1742-2094-7-34.
- Goldberg J.S., Hirschi K.K. Diverse roles of the vasculature within the neural stem cell niche. Regen Med 2009; 4(6): 879–897, http://dx.doi.org/10.2217/rme.09.61.
- Tavazoie M., Van der Veken L., Silva-Vargas V., Louissaint M., Colonna L., Zaidi B., Garcia-Verdugo J.M., Doetsch F. A specialized vascular niche for adult neural stem cells. Cell Stem Cell 2008; 3(3): 279–288, http://dx.doi.org/10.1016/j.stem.2008.07.025.
- Di Santo S., Seiler S., Fuchs A.L., Staudigl J., Widmer H.R. The secretome of endothelial progenitor cells promotes brain endothelial cell activity through PI3-kinase and MAP-kinase. PLoS One 2014; 9(4): e95731, http://dx.doi.org/10.1371/journal.pone.0095731.
- Liu L., Wei H., Chen F., Wang J., Dong J.F., Zhang J. Endothelial progenitor cells correlate with clinical outcome of traumatic brain injury. Crit Care Med 2011; 39 (7): 1760–1765, http://dx.doi.org/10.1097/CCM.0b013e3182186cee.
- Li L., Welser J.V., Dore-Duffy P., del Zoppo G.J., Lamanna J.C., Milner R. In the hypoxic central nervous system, endothelial cell proliferation is followed by astrocyte activation, proliferation, and increased expression of the alpha 6 beta 4 integrin and dystroglycan. Glia 2010; 58(10): 1157–1167, http://dx.doi.org/10.1002/glia.20995.
- Gómez-González B., Escobar A. Altered functional development of the blood-brain barrier after early life stress in the rat. Brain Res Bull 2009; 79(6): 376–387, http://dx.doi.org/10.1016/j.brainresbull.2009.05.012.
- Ek C.J., Dziegielewska K.M., Habgood M.D., Saunders N.R. Barriers in the developing brain and neurotoxicology. Neurotoxicology 2012; 33(3): 586–604, http://dx.doi.org/10.1016/j.neuro.2011.12.009.
- Lee H.S., Han J., Bai H.J., Kim K.W. Brain angiogenesis in developmental and pathological processes: regulation, molecular and cellular communication at the neurovascular interface. FEBS J 2009; 276(17): 4622–4635, http://dx.doi.org/10.1111/j.1742-4658.2009.07174.x.
- Stanimirovic D.B., Friedman A. Pathophysiology of the neurovascular unit: disease cause or consequence? J Cereb Blood Flow Metab 2012; 32(7): 1207–1221, http://dx.doi.org/10.1038/jcbfm.2012.25.
- Abbott N.J., Rönnbäck L., Hansson E. Astrocyte-endothelial interactions at the blood-brain barrier. Nat Rev Neurosci 2006; 7(1): 41–53, http://dx.doi.org/10.1038/nrn1824.
- Weidenfeller C., Svendsen C.N., Shusta E.V. Differentiating embryonic neural progenitor cells induce blood-brain barrier properties. J Neurochem 2007; 101(2): 555–565, http://dx.doi.org/10.1111/j.1471-4159.2006.04394.x.
- Ii M., Nishimura H., Sekiguchi H., Kamei N., Yokoyama A., Horii M., Asahara T. Concurrent vasculogenesis and neurogenesis from adult neural stem cells. Circ Res 2009; 105(9): 860–868, http://dx.doi.org/10.1161/CIRCRESAHA.109.199299.
- Ribatti D., Nico B., Crivellato E. The role of pericytes in angiogenesis. Int J Dev Biol 2011; 55(3): 261–268, http://dx.doi.org/10.1387/ijdb.103167dr.
- Gaengel K., Genové G., Armulik A., Betsholtz C. Endothelial-mural cell signaling in vascular development and angiogenesis. Arterioscler Thromb Vasc Biol 2009; 29(5): 630–638, http://dx.doi.org/10.1161/ATVBAHA.107.161521.
- Greenberg J.I., Shields D.J., Barillas S.G., Acevedo L.M., Murphy E., Huang J., Scheppke L., Stockmann C., Johnson R.S., Angle N., Cheresh D.A. A role for VEGF as a negative regulator of pericyte function and vessel maturation. Nature 2008; 456(7223): 809–813, http://dx.doi.org/10.1038/nature07424.
- Siegenthaler J.A., Choe Y., Patterson K.P., Hsieh I., Li D., Jaminet S.C., Daneman R., Kume T., Huang E.J., Pleasure S.J. Foxc1 is required by pericytes during fetal brain angiogenesis. Biol Open 2013; 2(7): 647–659, http://dx.doi.org/10.1242/bio.20135009.
- Nakamura-Ishizu A., Kurihara T., Okuno Y., Ozawa Y., Kishi K., Goda N., Tsubota K., Okano H., Suda T., Kubota Y. The formation of an angiogenic astrocyte template is regulated by the neuroretina in a HIF-1-dependent manner. Dev Biol 2012; 363(1): 106–114, http://dx.doi.org/10.1016/j.ydbio.2011.12.027.
- Yan L.J., Xiao M., Chen R., Cai Z. Metabolic dysfunction of astrocyte: an initiating factor in beta-amyloid pathology? Aging Neurodegener 2013; 1(1): 7–14.
- Shearer K.D., Fragoso Y.D., Clagett-Dame M., McCaffery P.J. Astrocytes as a regulated source of retinoic acid for the brain. Glia 2012; 60(12): 1964–1976, http://dx.doi.org/10.1002/glia.22412.
- Hayakawa K., Pham L.D., Arai K., Lo E.H. Reactive astrocytes promote adhesive interactions between brain endothelium and endothelial progenitor cells via HMGB1 and beta-2 integrin signaling. Stem Cell Res 2014; 12(2): 531–538, http://dx.doi.org/10.1016/j.scr.2013.12.008.
- Fan X., Heijnen C.J., van der Kooij M.A., Groenendaal F., van Bel F. The role and regulation of hypoxia-inducible factor-1alpha expression in brain development and neonatal hypoxic-ischemic brain injury. Brain Res Rev 2009; 62(1): 99–108, http://dx.doi.org/10.1016/j.brainresrev.2009.09.006.
- Biron K.E., Dickstein D.L., Gopaul R., Jefferies W.A. Amyloid triggers extensive cerebral angiogenesis causing blood brain barrier permeability and hypervascularity in Alzheimer’s disease. PLoS One 2011; 6(8): e23789, http://dx.doi.org/10.1371/journal.pone.0023789.
- Lu Z., Kipnis J. Thrombospondin 1 — a key astrocyte-derived neurogenic factor. FASEB J 2010; 24(6): 1925–1934, http://dx.doi.org/10.1096/fj.09-150573.
- Tian W., Sawyer A., Kocaoglu F.B., Kyriakides T.R. Astrocyte-derived thrombospondin-2 is critical for the repair of the blood-brain barrier. Am J Pathol 2011; 179(2): 860–868, http://dx.doi.org/10.1016/j.ajpath.2011.05.002.
- Crawford D.C., Jiang X., Taylor A., Mennerick S. Astrocyte-derived thrombospondins mediate the development of hippocampal presynaptic plasticity in vitro. J Neurosci 2012; 32(38): 13100–13110, http://dx.doi.org/10.1523/JNEUROSCI.2604-12.2012.
- Qin Q., Qian J., Ge L., Shen L., Jia J., Jin J., Ge J. Effect and mechanism of thrombospondin-1 on the angiogenesis potential in human endothelial progenitor cells: an in vitro study. PLoS One 2014; 9(2): e88213, http://dx.doi.org/10.1371/journal.pone.0088213.
- Lawler P.R., Lawler J. Molecular basis for the regulation of angiogenesis by thrombospondin-1 and -2. Cold Spring Harb Perspect Med 2012; 2(5): a006627, http://dx.doi.org/10.1101/cshperspect.a006627.
- Hirota K., Semenza G.L. Rac1 activity is required for the activation of hypoxia-inducible factor 1. J Biol Chem 2001; 276(24): 21166–21172, http://dx.doi.org/10.1074/jbc.M100677200.
- Racchetti G., D’Alessandro R., Meldolesi J. Astrocyte stellation, a process dependent on Rac1 is sustained by the regulated exocytosis of enlargeosomes. Glia 2012; 60(3): 465–475, http://dx.doi.org/10.1002/glia.22280.
- Murthy S., Ryan A., He C., Mallampalli R.K., Carter A.B. Rac1-mediated mitochondrial H2O2 generation regulates MMP-9 gene expression in macrophages via inhibition of SP-1 and AP-1. J Biol Chem 2010; 285(32): 25062–25073, http://dx.doi.org/10.1074/jbc.M109.099655.
- Aslam M., Schluter K.D., Rohrbach S., Rafiq A., Nazli S., Piper H.M., Noll T., Schulz R., Gündüz D. Hypoxia-reoxygenation-induced endothelial barrier failure: role of RhoA, Rac1 and myosin light chain kinase. J Physiol 2013; 591(Pt 2): 461–473, http://dx.doi.org/10.1113/jphysiol.2012.237834.
- Wojciak-Stothard B., Tsang L.Y., Paleolog E., Hall S.M., Haworth S.G. Rac1 and RhoA as regulators of endothelial phenotype and barrier function in hypoxia-induced neonatal pulmonary hypertension. Am J Physiol Lung Cell Mol Physiol 2006; 290(6): 1173–1182, http://dx.doi.org/10.1152/ajplung.00309.2005.
- Sawada N., Kim H.H., Moskowitz M.A., Liao J.K. Rac1 is a critical mediator of endothelium-derived neurotrophic activity. Sci Signal 2009; 2(61): ra10, http://dx.doi.org/10.1126/scisignal.2000162.
- Shen L., Gao Y., Qian J., Wu Y., Zhou M., Sun A., Zou Y., Ge J. The role of SDF-1α/Rac pathway in the regulation of endothelial progenitor cell polarity; homing and expression of Rac1, Rac2 during endothelial repair. Mol Cell Biochem 2012; 365(1-2): 1–7, http://dx.doi.org/10.1007/s11010-011-1083-z.
- Shen L., Gao Y., Qian J., Sun A., Ge J. A novel mechanism for endothelial progenitor cells homing: the SDF-1/CXCR4-Rac pathway may regulate endothelial progenitor cells homing through cellular polarization. Med Hypotheses 2011; 76(2): 256–258, http://dx.doi.org/10.1016/j.mehy.2010.10.014.
- Sun X., Tsipis C.P., Benderro G.F., Xu K., Lamanna J.C. Defining the role of HIF and its downstream mediators in hypoxic-induced cerebral angiogenesis. Methods Mol Biol 2014; 1135: 251–260, http://dx.doi.org/10.1007/978-1-4939-0320-7_21.
- Ruan G.X., Kazlauskas A. Lactate engages receptor tyrosine kinases Axl, Tie2, and vascular endothelial growth factor receptor 2 to activate phosphoinositide 3-kinase/Akt and promote angiogenesis. J Biol Chem 2013; 288(29): 21161–21172, http://dx.doi.org/10.1074/jbc.M113.474619.
- Milovanova T.N., Bhopale V.M., Sorokina E.M., Moore J.S., Hunt T.K., Hauer-Jensen M., Velazquez O.C., Thom S.R. Lactate stimulates vasculogenic stem cells via the thioredoxin system and engages an autocrine activation loop involving hypoxia-inducible factor 1. Mol Cell Biol 2008; 28(20): 6248–6261, http://dx.doi.org/10.1128/MCB.00795-08.
- Hunt T.K., Aslam R., Hussain Z., Beckert S. Lactate, with oxygen, incites angiogenesis. Adv Exp Med Biol 2008; 614: 73-80, http://dx.doi.org/10.1007/978-0-387-74911-2_9.
- Patten D.A., Lafleur V.N., Robitaille G.A., Chan D.A., Giaccia A.J., Richard D.E. Hypoxia-inducible factor-1 activation in nonhypoxic conditions: the essential role of mitochondrial-derived reactive oxygen species. Mol Biol Cell 2010; 21(18): 3247–3257, http://dx.doi.org/10.1091/mbc.E10-01-0025.
- Bergersen L.H., Gjedde A. Is lactate a volume transmitter of metabolic states of the brain? Front Neuroenergetics 2012; 4: 5, http://dx.doi.org/10.3389/fnene.2012.00005.
- Groschner L.N., Waldeck-Weiermair M., Malli R., Graier W.F. Endothelial mitochondria — less respiration, more integration. Pflugers Arch 2012; 464(1): 63–76, http://dx.doi.org/10.1007/s00424-012-1085-z.
- Brix B., Mesters J.R., Pellerin L., Jöhren O. Endothelial cell-derived nitric oxide enhances aerobic glycolysis in astrocytes via HIF-1α-mediated target gene activation. J Neurosci 2012; 32(28): 9727–9735, http://dx.doi.org/10.1523/JNEUROSCI.0879-12.2012.
- Polet F., Feron O. Endothelial cell metabolism and tumour angiogenesis: glucose and glutamine as essential fuels and lactate as the driving force. J Intern Med 2013; 273(2): 156–165, http://dx.doi.org/10.1111/joim.12016.
- Weidemann A., Krohne T.U., Aguilar E., Kurihara T., Takeda N., Dorrell M.I., Simon M.C., Haase V.H., Friedlander M., Johnson R.S. Astrocyte hypoxic response is essential for pathological but not developmental angiogenesis of the retina. Glia 2010; 58(10): 1177–1185, http://dx.doi.org/10.1002/glia.20997.
- Huang Y., Lei L., Liu D., Jovin I., Russell R., Johnson R.S., Di Lorenzo A., Giordano F.J. Normal glucose uptake in the brain and heart requires an endothelial cell-specific HIF-1α-dependent function. PNAS USA 2012; 109(43): 17478–17483, http://dx.doi.org/10.1073/pnas.1209281109.
- Tang F., Lane S., Korsak A., Paton J.F., Gourine A.V., Kasparov S., Teschemacher A.G. Lactate-mediated glia-neuronal signalling in the mammalian brain. Nat Commun 2014; 5: 3284, http://dx.doi.org/10.1038/ncomms4284.
- Lim J.H., Lee Y.M., Chun Y.S., Chen J., Kim J.E., Park J.W. Sirtuin 1 modulates cellular responses to hypoxia by deacetylating hypoxia-inducible factor 1alpha. Mol Cell 2010; 38(6): 864–878, http://dx.doi.org/10.1016/j.molcel.2010.05.023.
- Gräff J., Rei D., Guan J.S., Wang W.Y., Seo J., Hennig K.M., Nieland T.J., Fass D.M., Kao P.F., Kahn M., Su S.C., Samiei A., Joseph N., Haggarty S.J., Delalle I., Tsai L.H. An epigenetic blockade of cognitive functions in the neurodegenerating brain. Nature 2012; 483(7388): 222–226, http://dx.doi.org/10.1038/nature10849.
- Salmina A.B., Inzhutova A.I., Morgun A.V., Okuneva O.S., Malinovskaya N.A., Lopatina O.L., Petrova M.M., Taranushenko T.E., Fursov A.A., Kuvacheva N.V. NAD+-converting enzymes in neuronal and glial cells: CD38 as a novel target for neuroprotection. Vestnik RAMN 2012; 10: 29–37.
- Latham T., Mackay L., Sproul D., Karim M., Culley J., Harrison D.J., Hayward L., Langridge-Smith P., Gilbert N., Ramsahoye B.H. Lactate, a product of glycolytic metabolism, inhibits histone deacetylase activity and promotes changes in gene expression. Nucleic Acids Res 2012; 40(11): 4794–4803, http://dx.doi.org/10.1093/nar/gks066.
- Goyal M.S., Hawrylycz M., Miller J.A., Snyder A.Z., Raichle M.E. Aerobic glycolysis in the human brain is associated with development and neotenous gene expression. Cell Metab 2014; 19(1): 49–57, http://dx.doi.org/10.1016/j.cmet.2013.11.020.
- Petit J.M., Gyger J., Burlet-Godinot S., Fiumelli H., Martin J.L., Magistretti P.J. Genes involved in the astrocyte-neuron lactate shuttle (ANLS) are specifically regulated in cortical astrocytes following sleep deprivation in mice. Sleep 2013; 36(10): 1445–1458, http://dx.doi.org/10.5665/sleep.3034.
- Rey S., Semenza G.L. Hypoxia-inducible factor-1-dependent mechanisms of vascularization and vascular remodelling. Cardiovasc Res 2010; 86(2): 236–242, http://dx.doi.org/10.1093/cvr/cvq045.
- Chen W., Jadhav V., Tang J., Zhang J.H. HIF-1α inhibition ameliorates neonatal brain injury in a rat pup hypoxic-ischemic model. Neurobiol Dis 2008; 31(3): 433–441, http://dx.doi.org/10.1016/j.nbd.2008.05.020.
- Yan J., Zhou B., Taheri S., Shi H. Differential effects of HIF-1 inhibition by YC-1 on the overall outcome and blood-brain barrier damage in a rat model of ischemic stroke. PLoS One 2011; 6(11): e27798, http://dx.doi.org/10.1371/journal.pone.0027798.
- Reischl S., Li L., Walkinshaw G., Flippin L.A., Marti H.H., Kunze R. Inhibition of HIF prolyl-4-hydroxylases by FG-4497 reduces brain tissue injury and edema formation during ischemic stroke. PLoS One 2014; 9(1): e84767, http://dx.doi.org/10.1371/journal.pone.0084767.
- Liebner S., Czupalla C.J., Wolburg H. Current concepts of blood-brain barrier development. Int J Dev Biol 2011; 55(4–5): 467–476, http://dx.doi.org/10.1387/ijdb.103224sl.
- Levy A.F., Zayats M., Guerrero-Cazares H., Quiñones-Hinojosa A., Searson P.C. Influence of basement membrane proteins and endothelial cell-derived factors on the morphology of human fetal-derived astrocytes in 2D. PLoS One 2014; 9(3): e92165, http://dx.doi.org/10.1371/journal.pone.0092165.
- Lippmann E.S., Al-Ahmad A., Palecek S.P., Shusta E.V. Modeling the blood-brain barrier using stem cell sources. Fluids Barriers CNS 2013; 10(1): 2, http://dx.doi.org/10.1186/2045-8118-10-2.
- Lippmann E.S., Weidenfeller C., Svendsen C.N., Shusta E.V. Blood-brain barrier modeling with co-cultured neural progenitor cell-derived astrocytes and neurons. J Neurochem 2011; 119(3): 507–520, http://dx.doi.org/10.1111/j.1471-4159.2011.07434.x.
- Wurmser A.E., Nakashima K., Summers R.G., Toni N., D’Amour K.A., Lie D.C., Gage F.H. Cell fusion-independent differentiation of neural stem cells to the endothelial lineage. Nature 2004; 430(6997): 350–356, http://dx.doi.org/10.1038/nature02604.
- Lippmann E.S., Al-Ahmad A., Azarin S.M., Palecek S.P., Shusta E.V. A retinoic acid-enhanced, multicellular human blood-brain barrier model derived from stem cell sources. Sci Rep 2014; 4: 4160, http://dx.doi.org/10.1038/srep04160.
- Cecchelli R., Aday S., Sevin E., Almeida C., Culot M., Dehouck L., Coisne C., Engelhardt B., Dehouck M.P., Ferreira L. A stable and reproducible human blood-brain barrier model derived from hematopoietic stem cells. PLoS One 2014; 9(6): e99733, http://dx.doi.org/10.1371/journal.pone.0099733.
- Yamanaka S., Takahashi K. Induction of pluripotent stem cells from mouse fibroblast cultures. Tanpakushitsu Kakusan Koso 2006; 51(15): 2346–2351.
- Takahashi K., Okita K., Nakagawa M., Yamanaka S. Induction of pluripotent stem cells from fibroblast cultures. Nat Protoc 2007; 2(12): 3081–3089, http://dx.doi.org/10.1038/nprot.2007.418.
- Yagi T., Kosakai A., Ito D., Okada Y., Akamatsu W., Nihei Y., Nabetani A., Ishikawa F., Arai Y., Hirose N., Okano H., Suzuki N. Establishment of induced pluripotent stem cells from centenarians for neurodegenerative disease research. PLoS One 2012; 7(7): e41572, http://dx.doi.org/10.1371/journal.pone.0041572.