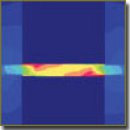
Nanoscale Modeling of Morphological Disordering of Mineral Matrix Elements
The aim of the investigation was to develop a nanoscale functional model of bone matrix by combining its mineral elements into a single structure of mineral compounds, analyze the axial disorder effect of its elements on the effective elastic modules, and the distribution of stress-strain fields in a modeling structure using a finite element technique.
Materials and Methods. We proposed a new morphological nanoscale model of bone tissue (at the level of collagen fibrils) taking into account the mineral bridges between the bonds of hydroxyapatite crystals based on Denisov-Nikolsky’s model. We analyzed the effect of variation of morphological characteristics on local stress-strain state and mechanical properties of the representative bone volume element by means of the direct finite element simulation and homogenization.
Results. The increase of minerals disorder degree was found to be accompanied by the increase of strain and stress in a modeled structure. An elastic modulus value is slightly affected by disordering. The biological relevance of the phenomenon is in that the mineral disorder, regardless of the force direction, always increases the risk of bone matrix damage.
Conclusion. We developed a nanoscale functional model of bone matrix by combining its mineral elements into a single structure using mineral compounds. We determined the impact of axial disordering of it elements on effective elastic modules, and the distribution of stress-strain fields in a modeling structure using a finite element technique. The analysis of the results showed the qualitative relationship of the findings with the experiment.
- Wallace J.M. Applications of atomic force microscopy for the assessment of nanoscale morphological and mechanical properties of bone. Bone 2012; 50(1): 420–427, http://dx.doi.org/10.1016/j.bone.2011.11.008.
- Milovanovic P., Potocnik J., Djonic D., Nikolic S., Zivkovic V., Djuric M., Rakocevic Z. Age-related deterioration in trabecular bone mechanical properties at material level: nanoindentation study of the femoral neck in women by using AFM. Exp Gerontol 2012; 47(2): 154–159, http://dx.doi.org/10.1016/j.exger.2011.11.011.
- Denisov-Nikol'skiy Yu.I., Mironov S.P., Omel'yanenko N.P., Matveychuk I.V. Aktual’nye problemy teoreticheskoy i klinicheskoy osteoartrologii [Current problems of theoretical and clinical osteoarthrology]. Moscow: OAO “Tipografiya Novosti”; 2005; 336 p.
- Knothe Tate M.L. Multiscale computational engineering of bones: state-of-the-art insights for the future. In: Engineering of functional skeletal tissues. Series title: Topics in bone biology. Vol. 3. Springer London; 2007; p. 141–160, http://dx.doi.org/10.1007/978-1-84628-366-6_10.
- Avrunin A.S., Semenov A.S., Fedorov I.V., Mel’nikov B.E., Doctorov A.A., Parshin L.K. Influence of the mineral bond between associations of crystallites on bone matrix mechanical properties. Modeling by the finite element method. Travmatologiya i ortopediya Rossii 2013; 2(68): 72–83.
- Doktorov A.A., Denisov-Nikol’skiy Yu.I. Relief features of mineralized surface of lacunes and canaliculi in lamellar bone. Bulleten’ eksperimental’noj biologii i mediciny 1993; 115(1): 61–65.
- Zhilkin B.A., Denisov-Nikol’skiy Yu.I., Doktorov A.A. Structure features of lamellar bone of human vertebrae in age involution and osteoporosis. Bulleten’ eksperimental’noj biologii i mediciny 2003; 135(4): 476–480.
- Awunin A.S., Tykhylov R.M., Abolin A.B., Shcherbak I.G. Levels of bone mineral matrix organization and the mechanisms determining parameters of its formation. Morfologiya 2005; 127(2): 78–82.
- Kornilov N.V., Avrunin A.S. Adaptatsionnye protsessy v organakh skeleta [Adaptive processes in skeletal organs]. Saint Petersburg: MORSAR AV; 2001; 296 p.
- Chatterji S., Wall C., Jeffery J. Changes in degree of orientation of bone minerals with age in the human femur. Experientia 1972; 28(2): 156–157, http://dx.doi.org/10.1007/bf01935727.
- Wang X., Li X., Shen X., Agrawal С.M. Age-related changes of noncalcified collagen in human cortical bone. Ann Biomed Eng 2003; 31(11): 1365–1371, http://dx.doi.org/10.1114/1.1623488.
- Wang X., Puram S. The toughness of cortical bone and its relationship with age. Ann Biomed Eng 2004; 32(1): 123–135, http://dx.doi.org/10.1023/b:abme.0000007797.92559.5e.
- Akkus O., Yeni Y.N., Wasserman N. Fracture mechanics of cortical bone tissue: a hierarchical perspective. Crit Rev Biomed Eng 2004; 32(5–6): 379–425, http://dx.doi.org/10.1615/critrevbiomedeng.v32.i56.10.
- Akkus O., Adar F., Schaffler M.B. Age-related changes in physicochemical properties of mineral crystals are related to impaired mechanical function of cortical bone. Bone 2004; 34(3): 443–453, http://dx.doi.org/10.1016/j.bone.2003.11.003.
- Currey J.D. Three analogies to explain the mechanical properties of bone. Biorheology 1964; 2: 1–10.
- Jäger I., Fratzl P. Mineralized collagen fibrils: a mechanical model with a staggered arrangement of mineral particles. Biophys J 2000; 79(4): 1737–1746, http://dx.doi.org/10.1016/S0006-3495(00)76426-5.
- Semenov A.S. PANTOCRATOR — konechno-elementnyy programmnyy kompleks, orientirovannyy na reshenie nelineynykh zadach mekhaniki. V kn.: Trudy V mezhdunarodnoy konferentsii “Nauchno-tekhnicheskie problemy prognozirovaniya nadezhnosti i dolgovechnosti konstruktsiy i metody ikh resheniya” [PANTOCRATOR — finite element program complex focused on solving non-linear problems of mechanics. In: Proceedings of V international conference “Scientific and technical problems of prediction of reliability and durability of constructions and the techniques for their solution”]. Saint Petersburg: Izd-vo SPbGPU; 2003; p. 466–480.
- N'yuman U., N'yuman M. Mineral’nyy obmen kosti [Bone mineral metabolism]. Moscow: Inostrannaya literatura; 1961; 269 p.
- Boyde A., Hobdell M.H. Scanning electron microscopy of lamellar bone. Z Zellforsch Mikrosk Anat 1969; 93(2): 213–231, http://dx.doi.org/10.1007/bf00336690.
- Boyde A. Scanning electron microscope studies of bone. In: The biochemistry and physiology of bone. Vol I: Structure. Edited by Bourne G.H. Elsevier BV; 1972; p. 259–310, http://dx.doi.org/10.1016/b978-0-12-119201-3.50015-9.
- Fazzalari N.L., Forwood M.R., Manthey B.A., Smith K., Kolesik P. Three-dimensional confocal images of microdamage in cancellous bone. Bone 1998; 23(4): 337–378, http://dx.doi.org/10.1016/s8756-3282(98)00111-2.
- Wenzel T.E., Schaffler M.B., Fyhrie D.P. In vivo trabecular microcracks in human vertebral bone. Bone 1996; 19(1): 89–95, http://dx.doi.org/10.1016/8756-3282(96)88871-5.
- Avrunin A.S., Tikhilov R.M., Parshin L.K., Melnikov B.E. Hierarchic skeletal organization — a factor regulating the structure of fatigue injuries. Part II. Hypothetical model of formation and distruption of bonds between crystallite associations. Travmatologiya i ortopediya Rossii 2010; 1(55): 48–57.