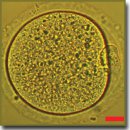
Determining the Range of Noninvasive Near-Infrared Femtosecond Laser Pulses for Mammalian Oocyte Nanosurgery
The aim of the investigation was to determine a noninvasive range of near-infrared femtosecond laser pulse exposure for mouse oocyte intracellular nanosurgery and to estimate oocyte developmental competence in vitro depending on pulse energy and total exposure time.
Materials and Methods. The model object was a preovulatory germinal vesicle mouse oocyte. Femtosecond laser radiation with a central wavelength of 780 nm was utilized in two modes: 1) single pulses with 30 fs duration and pulse energy up to 100 nJ; 2) trains of pulses with 100 fs duration, 80 MHz repetition rate (12.5 ns between pulses) and pulse energy from 0.5 to 2 nJ; total duration of the pulse trains ranged from 15 to 60 ms. Radiation was focused by a microscope objective lens (60×, 0.7 NA). Oocytes were cultured to the metaphase of the second meiotic division, which was used as invasiveness criteria. This stage was detected by polar bodies and metaphase plate formation.
Results. The threshold of invasiveness was determined for a single femtosecond pulse exposure. Cytoplasmic membrane rupture and cell disruption occurred under the exposure of a single 30 fs pulse with 100 nJ energy. The cytoplasm and nucleus exposure to femtosecond pulse trains did not induce permanent damage to the oocytes. The rate of gas-vapor bubble formation was measured after the action of pulse trains on the cytoplasm and nucleus chromatin of mammalian oocytes. The pulse energy of 1 nJ is a threshold value for bubble formation in the cytoplasm, and 2 nJ in the nucleus. Exposure to laser pulse trains with 80 MHz repetition rate and 100 fs duration didn’t change oocyte ability to achieve metaphase II stage within the studied range of pulse energies and pulse train durations.
Conclusion. Surgery with femtosecond pulse trains at 80 MHz repetition rate and pulse energy not more than twice as high as the threshold of cavitation or vapor bubble formation does not affect oocyte development to the metaphase II stage. Femtosecond laser nanosurgery can be regarded as a minimally invasive method of intracellular surgery within the investigated range of pulse energies and exposure times.
- Nadtochenko V.A., Mel’nikov M.Ya. Lazernaya nanokhirurgiya kletok i embrionov. V kn.: Sintez, stroenie i svoystva metall/poluprovodnik soderzhashchikh nanostrukturirovannykh kompozitov [Laser nanosurgery of the cells and embryos. In: Synthesis, structure and properties of metal/semiconductor-containing nanostructured composites]. Pod red. Trakhtenberg L.I., Mel’nikova M.Ya. [Trakhtenberg L.I., Mel’nikov M.Ya. (editors)]. Moscow: Tekhnosfera; 2016; p. 441–473.
- Nuzzo V., Maxwell I., Chung S., Mazur E., Heisterkamp A. Subcellular surgery and nanoneurosurgery using femtosecond laser pulses. In: Biophotonics: spectroscopy, imaging, sensing, and manipulation. DiBartolo B., Collins J. (editors). Springer; 2011; p. 203–218, https://doi.org/10.1007/978-90-481-9977-8_9.
- Osychenko A.A., Zalesskii A.D., Krivokharchenko A.S., Zhakhbazyan A.K., Ryabova A.V., Nadtochenko V.A. Fusion of blastomeres in mouse embryos under the action of femtosecond laser radiation.
Efficiency of blastocyst formation and embryo development. Quantum Electronics 2015; 45(5): 498–502, https://doi.org/10.1070/qe2015v045n05abeh015767. - Vogel A., Noack J., Hüttman G., Paltauf G. Mechanisms of femtosecond laser nanosurgery of cells and tissues. Applied Physics B 2005; 81(8): 1015–1047, https://doi.org/10.1007/s00340-005-2036-6.
- Shishova K.V., Khodarovich Y.M., Lavrentyeva E.A., Zatsepina O.V. High-resolution microscopy of active ribosomal genes and key members of the rRNA processing machinery inside nucleolus-like bodies of
fully-grown mouse oocytes. Experimental Cell Research 2015; 337(2): 208–218, https://doi.org/10.1016/j.yexcr.2015.07.024. - Shakhov A.M., Astafiev A.A., Plutenko D.O., Sarkisov O.M., Shushin A.I., Nadtochenko V.A. Femtosecond optical
trap-assisted nanopatterning through microspheres by a singleTi:Sapphire oscillator. The Journal of Physical Chemistry C 2015; 119(22): 12562–12571, https://doi.org/10.1021/acs.jpcc.5b00478. - Astaf’ev A.A., Shakhov A.M., Sarkisov O.M., Nadtochenko V.A. Microstructuring of polymer films by femtosecond pulses through optically trapped polystyrene microspheres. Quantum Electronics 2013; 43(4): 361–364, https://doi.org/10.1070/qe2013v043n04abeh015114.
Sarpe C., Köhler J., Winkler T., Wollenhaupt M., Baumert T. Real-time observation of transient electron density in water irradiated with tailored femtosecond laser pulses. New Journal of Physics 2012; 14(7): 075021, https://doi.org/10.1088/1367-2630/14/7/075021.