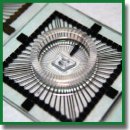
Study of Stimulus-Induced Plasticity in Neural Networks Cultured in Microfluidic Chips
Dissociated brain cell cultures on microelectrode arrays are widely used to study fundamental mechanisms of information processing and synaptic plasticity. It has been established that high frequency electrical stimulation causes functional changes in neural networks. However, complex and homogeneous morphological structure of cultured brain cell networks presents a significant challenge for further evaluation of the synaptic plasticity at the network level. In this study, we propose a new approach to studying neural network plasticity using microfluidic devices with specially designed channels. Microfluidic chips can guide axons and form neural circuits with two subnetworks connected by synaptic paths in the required direction. To induce synaptic plasticity, high frequency tetanic stimulation by two groups of electrodes located in the area of pre- and postsynaptic neurons was applied. The developed method of potentiation and depression of the required functional connectivity in the neural circuit can be used to further study network effects of synaptic plasticity induced in the local subpopulation of cells.
- Marom S., Shahaf G. Development, learning and memory in large random networks of cortical neurons: lessons beyond anatomy. Q Rev Biophys 2002; 35(01): 63–87, https://doi.org/10.1017/s0033583501003742.
- Le Feber J., Stegenga J., Rutten W.L.C. The effect of slow electrical stimuli to achieve learning in cultured networks of rat cortical neurons. PLoS One 2010; 5(1): e8871, https://doi.org/10.1371/journal.pone.0008871.
Pimashkin A., Gladkov A., Mukhina I., Kazantsev V. Adaptive enhancement of learning protocol in hippocampal cultured networks grown on multielectrode arrays. Front Neural Circuits 2013; 7: 87, https://doi.org/10.3389/fncir.2013.00087.- Li Y., Zhou W., Li X., Zeng S., Luo Q. Dynamics of learning in cultured neuronal networks with antagonists of glutamate receptors. Biophys J 2007; 93(12): 4151–4158, https://doi.org/10.1529/biophysj.107.111153.
- Bologna L.L., Nieus T., Tedesco M., Chiappalone M., Benfenati F., Martinoia S. Low-frequency stimulation enhances burst activity in cortical cultures during development. Neuroscience 2010; 165(3): 692–704, https://doi.org/10.1016/j.neuroscience.2009.11.018.
- Brewer G.J., Boehler M.D., Ide A.N., Wheeler B.C. Chronic electrical stimulation of cultured hippocampal networks increases spontaneous spike rates. J Neurosci Methods 2009; 184(1): 104–109, https://doi.org/10.1016/j.jneumeth.2009.07.031.
- Jimbo Y., Robinson H.P.C., Kawana A. Strengthening of synchronized activity by tetanic stimulation in cortical cultures: application of planar electrode arrays. IEEE Trans Biomed Eng 1998; 45(11): 1297–1304, https://doi.org/10.1109/10.725326.
- Jimbo Y., Tateno T., Robinson H.P.C. Simultaneous induction of pathway-specific potentiation and depression in networks of cortical neurons. Biophys J 1999; 76(2): 670–678, https://doi.org/10.1016/s0006-3495(99)77234-6.
- Wagenaar D.A., Pine J., Potter S.M. Searching for plasticity in dissociated cortical cultures on multi-electrode arrays. J Negat Results Biomed 2006; 5(1): 16, https://doi.org/10.1186/1477-5751-5-16.
- Chiappalone M., Massobrio P., Martinoia S. Network plasticity in cortical assemblies. Eur J Neurosci 2008; 28(1): 221–237, https://doi.org/10.1111/j.1460-9568.2008.06259.x.
- Chiappalone M., Bove M., Vato A., Tedesco M., Martinoia S. Dissociated cortical networks show spontaneously correlated activity patterns during in vitro development. Brain Res 2006; 1093(1): 41–53, https://doi.org/10.1016/j.brainres.2006.03.049.
- Feber J. le, Rutten W.L.C., Stegenga J., Wolters P.S., Ramakers G.J.A., Pelt J. van. Conditional firing probabilities in cultured neuronal networks: a stable underlying structure in widely varying spontaneous activity patterns. J Neural Eng 2007; 4(2): 54–67, https://doi.org/10.1088/1741-2560/4/2/006.
- Taylor A.M., Blurton-Jones M., Rhee S.W., Cribbs D.H., Cotman C.W., Jeon N.L. A microfluidic culture platform for CNS axonal injury, regeneration and transport. Nat Methods 2005; 2(8): 599–605, https://doi.org/10.1038/nmeth777.
- Kanagasabapathi T.T., Ciliberti D., Martinoia S., Wadman W.J., Decré M.M. Dual-compartment
neurofluidic system for electrophysiological measurements in physically segregated and functionally connected neuronal cell culture. Front Neuroeng 2011; 4: 13, https://doi.org/10.3389/fneng.2011.00013. - Takayama Y., Kotake N., Haga T., Suzuki T., Mabuchi K. Formation of one-way-structured cultured neuronal networks in microfluidic devices combining with micropatterning techniques. J Biosci Bioeng 2012; 114(1): 92–95, https://doi.org/10.1016/j.jbiosc.2012.02.011.
- Bisio M., Bosca A., Pasquale V., Berdondini L., Chiappalone M. Emergence of bursting activity in connected neuronal sub-populations. PLoS One 2014; 9(9): e107400, https://doi.org/10.1371/journal.pone.0107400.
- Le Feber J., Postma W., de Weerd E., Weusthof M., Rutten W.L.C. Barbed channels enhance unidirectional connectivity between neuronal networks cultured on multi electrode arrays. Front Neurosci 2015; 9: 412, https://doi.org/10.3389/fnins.2015.00412.
- Pan L., Alagapan S., Franca E., Leondopulos S.S., DeMarse T.B., Brewer G.J., Wheeler B.C. An in vitro method to manipulate the direction and functional strength between neural populations. Front Neural Circuits 2015; 9: 32, https://doi.org/10.3389/fncir.2015.00032.
- Yoshizumi Y., Honegger T., Berton K., Suzuki H., Peyrade D. Micromotors: trajectory control of self-propelled micromotors using AC electrokinetics (Small 42/2015). Small 2015; 11(42): 5629–5629, https://doi.org/10.1002/smll.201570255.
- Habibey R., Golabchi A., Latifi S., Difato F., Blau A. A microchannel device tailored to laser axotomy and long-term microelectrode array electrophysiology of functional regeneration. Lab Chip 2015; 15(24): 4578–4590, https://doi.org/10.1039/c5lc01027f.
- Pimashkin A., Kastalskiy I., Simonov A., Koryagina E., Mukhina I., Kazantsev V. Spiking signatures of spontaneous activity bursts in hippocampal cultures. Front Comput Neurosci 2011; 5: 46, https://doi.org/10.3389/fncom.2011.00046.
- Malishev E., Pimashkin A., Gladkov A., Pigareva Y., Bukatin A., Kazantsev V., Mukhina I., Dubina M. Microfluidic device for unidirectional axon growth. J Phys Condens Matter 2015; 643: 01202, https://doi.org/10.1088/1742-6596/643/1/012025.
- Bi G.Q., Poo M.M. Synaptic modifications in cultured hippocampal neurons: dependence on spike timing, synaptic strength, and postsynaptic cell type. J Neurosci 1998; 18(24): 10464–10472.
- Debanne D., Gahwiler B.H., Thompson S.M. Asynchronous pre- and postsynaptic activity induces associative long-term depression in area CA1 of the rat hippocampus in vitro. Proc Natl Acad Sci USA 1994; 91(3): 1148–1152, https://doi.org/10.1073/pnas.91.3.1148.
- Woodin M.A., Ganguly K., Poo M. Coincident pre- and postsynaptic activity modifies gabaergic synapses by postsynaptic changes in Cl− transporter activity. Neuron 2003; 39(5): 807–820, https://doi.org/10.1016/s0896-6273(03)00507-5.
- Chen X., Dzakpasu R. Observed network dynamics from altering the balance between excitatory and inhibitory neurons in cultured networks. Phys Rev E Stat Nonlin Soft Matter Phys 2010; 82(3): 031907, https://doi.org/10.1103/physreve.82.031907.
- Jinno S., Kosaka T. Stereological estimation of numerical densities of glutamatergic principal neurons in the mouse hippocampus. Hippocampus 2010; 20(7): 829–840, https://doi.org/10.1002/hipo.20685.