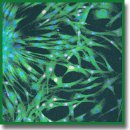
Transparent PEG-Fibrin Gel as a Flexible Tool for Cell Encapsulation
The aim of this study was to modify the chemical structure and to optimize the composition of the fibrin gel for effective cell encapsulation.
Materials and Methods. We prepared PEGylated fibrin gels using different fibrinogen concentrations (25–50 mg/ml) and PEG-fibrinogen molar ratio 10:1 and 5:1 and characterized them via Fourier transform infrared spectroscopy and differential scanning calorimetry. Within the gels, we encapsulated primary culture of fibroblasts and analyzed using light and laser confocal microscopy.
Results. PEGylation of fibrinogen allowed us to achieve the gel transparency and preserve its biocompatibility. We revealed that the gel prepared from PEGylated 5:1 fibrinogen (25 mg/ml) provided the most favorable microenvironment for spreading, growth, and proliferation of fibroblasts. This PEG-fibrin gel can be used for encapsulation of different cell types that is essential for various approaches in tissue engineering and diagnostic systems.
- Prewitz M.C., Seib F.P., von Bonin M., Friedrichs J., Stißel A., Niehage C., Müller K., Anastassiadis K., Waskow C., Hoflack B., Bornhäuser M., Werner C. Tightly anchored tissue-mimetic matrices as instructive stem cell microenvironments. Nat Methods 2013; 10(8): 788–794, https://doi.org/10.1038/nmeth.2523.
- Shaikh F.M., Callanan A., Kavanagh E.G., Burke P.E., Grace P.A., McGloughlin T.M. Fibrin: a natural biodegradable scaffold in vascular tissue engineering. Cells Tissues Organs 2008; 188(4): 333–346, https://doi.org/10.1159/000139772.
- Rowe S.L., Lee S., Stegemann J.P. Influence of thrombin concentration on the mechanical and morphological properties of cell-seeded fibrin hydrogels. Acta Biomater 2007; 3(1): 59–67, https://doi.org/10.1016/j.actbio.2006.08.006.
- Bryant S.J., Durand K.L., Anseth K.S. Manipulations in hydrogel chemistry control photoencapsulated chondrocyte behavior and their extracellular matrix production. J Biomed Mater Res 2003; 67A(4): 1430–1436, https://doi.org/10.1002/jbm.a.20003.
- Galler K.M., Cavender A.C., Koeklue U., Suggs L.J., Schmalz G., D’Souza R.N. Bioengineering of dental stem cells in a PEGilated fibrin gel. Regen Med 2011; 6(2): 191–200, https://doi.org/10.2217/rme.11.3.
- Touahir L., Chazalviel J.-N., Sam S., Moraillon A., Henry de Villeneuve C., Allongue P., Ozanam F., Gouget-Laemmel A.C. Kinetics of activation of carboxyls to succinimidyl ester groups in monolayers grafted on silicon: an in situ real-time infrared spectroscopy study. J Phys Chem C 2011; 115(14): 6782–6787, https://doi.org/10.1021/jp200150m.
- Frey B.L., Corn R.M. Covalent attachment and derivatization of poly(l-lysine) monolayers on gold surfaces as characterized by polarization–modulation FT-IR spectroscopy. Anal Chem 1996; 68(18): 3187–3193, https://doi.org/10.1021/ac9605861 .
- Hu X., Kaplan D., Cebe P. Determining beta-sheet crystallinity in fibrous proteins by thermal analysis and infrared spectroscopy. Macromolecules 2006; 39(18): 6161–6170, https://doi.org/10.1021/ma0610109.
- Shpichka A.I., Koroleva A.V., Deiwick A., Timashev P.S., Semenova E.F., Moiseeva I.Y., Konoplyannikov M.A., Chichkov B.N. Evaluation of the vasculogenic potential of hydrogels based on modified fibrin. Cell and Tissue Biology 2017; 11(1): 81–87, https://doi.org/10.1134/s1990519x17010126.
- Hoffman B.D., Grashoff C., Schwartz M.A. Dynamic molecular processes mediate cellular mechanotransduction. Nature 2011; 475(7356): 316–323, https://doi.org/10.1038/nature10316.
- Bensaïd W., Triffittb J.T., Blanchata C., Oudina K., Sedela L., Petite H. A biodegradable fibrin scaffold for mesenchymal stem cell transplantation. Biomaterials 2003; 24(14): 2497–2502, https://doi.org/10.1016/s0142-9612(02)00618-x.
- Dikovsky D., Bianco-Peled H., Seliktar D. The effect of structural alterations of PEG-fibrinogen hydrogel scaffolds on 3-D cellular morphology and cellular migration. Biomaterials 2006; 27(8): 1496–1506, https://doi.org/10.1016/j.biomaterials.2005.09.038.
- Hughes C.S., Postovit L.M., Lajoie G. Matrigel: a complex protein mixture required for optimal growth of cell culture. Proteomics 2010; 10(9): 1886–1890, https://doi.org/10.1002/pmic.200900758.
- Veronese F.M. Peptide and protein PEGylation: a review of problems and solutions. Biomaterials 2001; 22(5): 405–417, https://doi.org/10.1016/s0142-9612(00)00193-9.
- Zhang G., Wang X., Wang Z., Zhang J., Suggs L. A PEGylated fibrin patch for mesenchymal stem cell delivery. Tissue Eng 2006; 12(1): 9–19, https://doi.org/10.1089/ten.2006.12.9.