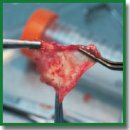
Application of Recellularized Non-Woven Materials from Collagen-Enriched Polylactide for Creation of Tissue-Engineered Diaphragm Constructs
The aim of the investigation was to study biocompatibility and biomechanical properties of recellularized non-woven materials based on collagen-filled polylactide under in vitro and in vivo conditions and to assess the potential of their use for diaphragm defect replacement in the experiment on small laboratory animals.
Materials and Methods. Non-woven materials were obtained by electroforming of polylactide in a tight box with subsequent specimen vacuumization. 9% aqueous solution of polylactide was prepared in the solvent mixture: chemically pure chloroform with 10% addition of chemically pure ethanol. The microfibrous material was enriched with collagen in a weight concentration of 0.1 and 0.5% with subsequent freezing at –40° or in liquid nitrogen. The structure of non-woven polylactide matrices was assessed by a scanning electron microscopy while mechanical properties were evaluated during cyclic tests for strength and fatigue properties using tensile-testing machine. The fabricated non-woven matrices were recellularized by mesenchymal stromal cells under static conditions. The quality of the fabricated tissue-engineered constructs was evaluated morphologically by routine histological investigations. The viability of the cells on the matrices and the cytotoxic properties of the matrices were determined using XTT tests. The least toxic specimens were orthotopically implanted into the rats with subsequent morphological examinations.
Results. The morphological analysis and viability study of the cells on the matrices of those specimens which underwent freezing in liquid nitrogen during forming were found to be inexpedient for orthotopic transplantation. The specimens frozen in the refrigeration unit demonstrated permissible cytotoxicity levels and were implanted. The morphological examination after explantation of the given specimen groups did not reveal significant damage to the structure and inflammatory changes though the signs of the marked adhesive process in the implantation area were evident.
Conclusion. In spite of the ability of the non-woven matrices to stimulate fibrinogenesis, absence of cytotoxic properties and evident reactions of transplant rejection allow the non-woven polylactide matrices undergone freezing in the refrigeration unit at –40° during the forming process to be considered promising for creation of tissue-engineered constructs of a diaphragm.
- De Coppi P., Deprest J. Regenerative medicine solutions in congenital diaphragmatic hernia, seminars in pediatric surgery. Semin Pediatr Surg 2017; 26(3): 171–177, https://doi.org/10.1053/j.sempedsurg.2017.04.009.
- De Coppi P. Regenerative medicine for congenital malformations. J Pediatr Surg 2013; 48(2): 273–280, https://doi.org/10.1016/j.jpedsurg.2012.11.005.
- Gubareva E.A., Sjöqvist S., Gilevich I.V., Sotnichenko A.S., Kuevda E.V., Lim M.L., Feliu N., Lemon G., Danilenko K.A., Nakokhov R.Z., Gumenyuk I.S., Grigoriev T.E., Krasheninnikov S.V., Pokhotko A.G., Basov A.A., Dzhimak S.S., Gustafsson Y., Bautista G., Beltrán Rodríguez A., Pokrovsky V.M., Jungebluth P., Chvalun S.N., Holterman M.J., Taylor D.A., Macchiarini P. Orthotopic transplantation of a tissue engineered diaphragm in rats. Biomaterials 2016; 77: 320–335, https://doi.org/10.1016/j.biomaterials.2015.11.020.
- Romao R.L., Nasr A., Chiu P.P., Langer J.C. What is the best prosthetic material for patch repair of congenital diaphragmatic hernia? Comparison and meta-analysis of porcine small intestinal submucosa and polytetrafluoroethylene. J Pediatr Surg 2012; 47(8): 1496–1500, https://doi.org/10.1016/j.jpedsurg.2012.01.009.
- Łukasiewicz A., Drewa T. Synthetic implants in hernia surgery. Adv Clin Exp Med 2014; 23(1): 135–142, https://doi.org/10.17219/acem/37037.
- Ghanavati Z., Neisi N., Bayati V., Makvandi M. The influence of substrate topography and biomaterial substance on skin wound healing. Anat Cell Biol 2015; 48(4): 251–257, https://doi.org/10.5115/acb.2015.48.4.251.
- Arutyunyan I.V., Tenchurin T.Kh., Kananykhina E.Y., Chernikov V.P., Vasyukova O.A., Elchaninov A.V., Makarov A.V., Korshunov A.A., Burov A.A., Podurovskaya Y.L., Chuprynin V.D., Uvarova E.V., Degtyarev D.N., Shepelev A.D., Mamagulashvili V.G., Kamyshinskiy R.A., Krasheninnikov S.V., Chvalun S.N., Fatkhudinov T.Kh. Nonwoven polycaprolactone scaffolds for tissue engineering: the choice of the structure and the method of cell seeding. Genes and Cells 2017; 12(1)1: 62–71, https://doi.org/10.23868/201703009.
- Zhao W., Ju Y.M., Christ G., Atala A., Yoo J.J., Lee S.J. Diaphragmatic muscle reconstruction with an aligned electrospun poly(ε-caprolactone)/collagen hybrid scaffold. Biomaterials 2013; 34(33): 8235–8240, https://doi.org/10.1016/j.biomaterials.2013.07.057.
- Pawar R.P., Tekale S.U., Shisodia S.U., Totre J.T., Domb A.J. Biomedical applications of poly(lactic acid). Recent Pat Regen Med 2014; 4(1): 40–51, https://doi.org/10.2174/2210296504666140402235024.
- Hanson S., D’Souza R.N., Hematti P. Biomaterial-mesenchymal stem cell constructs for immunomodulation in composite tissue engineering. Tissue Eng Part A 2014; 20(15–16): 2162–2168, https://doi.org/10.1089/ten.tea.2013.0359.
- Baker S.R., Banerjee S., Bonin K., Guthold M. Determining the mechanical properties of electrospun poly-ε-caprolactone (PCL) nanofibers using AFM and a novel fiber anchoring technique. Mater Sci Eng C Mater Biol Appl 2016; 59: 203–112, https://doi.org/10.1016/j.msec.2015.09.102.
- Bagaeva V.V., Popova V.M., Pashkova G.S., Isadzhanyan K.E., Nikitin V.V., Zhilenkov E.L. The study the efficacy and safety of antimicrobial agents. Issledovaniya i praktika v meditsine 2015; 2(3): 35–42, https://doi.org/10.17709/2409-2231-2015-2-3-35-42.
- Yamanaka K., Yamamoto K., Sakai Y., Suda Y., Shigemitsu Y., Kaneko T., Kato K., Kumagai T., Kato Y. Seeding of mesenchymal stem cells into inner part of interconnected porous biodegradable scaffold by a new method with a filter paper. Dent Mater J 2015; 34(1): 78–85, https://doi.org/10.4012/dmj.2013-330.
- Lv X.G., Feng C., Fu Q., Xie H., Wang Y., Huang J.W., Xie M.K., Atala A., Xu Y.M., Zhao W.X. Comparative study of different seeding methods based on a multilayer SIS scaffold: which is the optimal procedure for urethral tissue engineering? J Biomed Mater Res B Appl Biomater 2016; 104(6): 1098–1108, https://doi.org/10.1002/jbm.b.33460.
- Brouwer K.M., Daamen W.F., van Lochem N., Reijnen D., Wijnen R.M., van Kuppevelt T.H. Construction and in vivo evaluation of a dual layered collagenous scaffold with a radial pore structure for repair of the diaphragm. Acta Biomater 2013; 9(6): 6844–6851, https://doi.org/10.1016/j.actbio.2013.03.003.
- Urita Y., Komuro H., Chen G., Shinya M., Saihara R., Kaneko M. Evaluation of diaphragmatic hernia repair using PLGA mesh-collagen sponge hybrid scaffold: an experimental study in a rat model. Pediatr Surg Int 2008; 24(9): 1041–1045, https://doi.org/10.1007/s00383-008-2212-y.
- Conconi M.T., De Coppi P., Bellini S., Zara G., Sabatti M., Marzaro M., Zanon G.F., Gamba P.G., Parnigotto P.P., Nussdorfer G.G. Homologous muscle acellular matrix seeded with autologous myoblasts as a tissue-engineering approach to abdominal wall-defect repair. Biomaterials 2005; 26(15): 2567–2574, https://doi.org/10.1016/j.biomaterials.2004.07.035.
- Romanova O.A., Grigor’ev T.E., Goncharov M.E., Rudyak S.G., Solov’yova E.V., Krasheninnikov S.V., Saprykin V.P., Sytina E.V., Chvalun S.N., Pal’tsev M.A., Panteleev A.A. Chitosan as a modifying component of artificial scaffold for tissue engineering of human skin. Kletochnye tekhnologii v biologii i meditsine 2015; 2: 103–113.