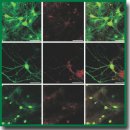
Effect of Photosensitizers Photosens, Photodithazine and Hypericin on Glioma Сells and Primary Neuronal Cultures: a Comparative Analysis
The aim of the study was to compare the effect of photosensitizers photosens, photodithazine, and hypericin on primary brain cell cultures, and assess their toxic effect on tumor and normal nervous cells in order to choose the optimal photodynamic agent for glioma therapy.
Materials and Methods. The cytotoxicity of photosens (NIOPIK, Russia), photodithazine (Veta-grand, Russia) and hypericin (Merck KGaA; Sigma-Aldrich, Germany) was assessed on primary brain cell cultures obtained from C57BL/6 mice (gestation day 18). On day 14 of cultivation, the tested photosensitizers were added to a culture medium at concentrations of 0.1, 1, 10, 50, and 100 µM. Then the cultures were placed in a СО2-incubator in the dark. The viability of primary neuronal cultures was estimated on days 3 and 7 after photosensitizer application. Using confocal microscopy, we analyzed the rate of entry and subcellular localization of the tested agents in the primary neuronal cells. Statistical analysis was performed in SigmaPlot 11.0 (Systat Software Inc., USA) using ANOVA.
Results. We analyzed the absorption and fluorescence spectra of the tested photosensitizers. Photosens and photodithazine showed the presence of absorption maximum in short- and long-wave spectral ranges. Hypericin was characterized by a complex spectrum with many peaks in both blue-violet and orange-red spectral ranges. Cell viability analysis revealed that high concentrations of photosensitizers caused a pronounced toxic effect on nervous cells. The most marked effect was shown for photodithazine. Photosens exhibited the lowest accumulation rate in primary neuronal cells. Photosens and hypericin were found to have a high phototoxic effect on glioma, and demonstrated low dark toxicity for normal brain cells.
Conclusion. The photosensitizers hypericin and photosens are the least toxic for nervous tissue, though effectively penetrating in tumor cells. These properties enable to consider them as prospective photodynamic agents for clinic.
- Ostrom Q.T., Gittleman H., Xu J., Kromer C., Wolinsky Y., Kruchko C., Barnholtz-Sloan J.S. CBTRUS statistical report: primary brain and other central nervous system tumors diagnosed in the United States in 2009–2013. Neuro Oncol 2016; 18(suppl_5): v1–v75, https://doi.org/10.1093/neuonc/now207.
- Ostrom Q.T., Gittleman H., Liao P., Vecchione-Koval T., Wolinsky Y., Kruchko C., Barnholtz-Sloan J.S. CBTRUS statistical report: primary brain and other central nervous system tumors diagnosed in the United States in 2010–2014. Neuro Oncol 2017; 19(suppl_5): v1–v88, https://doi.org/10.1093/neuonc/nox158.
- Cohen A.L., Colman H. Glioma biology and molecular markers. In: Raizer J., Parsa A. (editors). Current understanding and treatment of gliomas. Cancer treatment and research. Vol. 163. Springer, Cham; 2015; p. 15–30, https://doi.org/10.1007/978-3-319-12048-5_2.
- Kubasova I.Yu., Vakulovskaya E.G., Ermakova K.V., Smirnova Z.S. Fluorescent detection and photodynamic therapy in treatment of brain tumors. Rossiyskiy bioterapevticheskiy zhurnal 2006; 5(4): 54–67.
- Lacroix M., Abi-Said D., Fourney D.R., Gokaslan Z.L., Shi W., DeMonte F., Lang F.F., McCutcheon I.E., Hassenbusch S.J., Holland E., Hess K., Michael C., Miller D., Sawaya R. A multivariate analysis of 416 patients with glioblastoma multiforme: prognosis, extent of resection, and survival. J Neurosurg 2001; 95(2): 190–198, https://doi.org/10.3171/jns.2001.95.2.0190.
- Jiang H., Cui Y., Liu X., Ren X., Lin S. Patient-specific resection strategy of glioblastoma multiforme: choice based on a preoperative scoring scale. Ann Surg Oncol 2017; 24(7): 2006–2014, https://doi.org/10.1245/s10434-017-5843-1.
- Sarkaria J.N., Hu L.S., Parney I.F., Pafundi D.H., Brinkmann D.H., Laack N.N., Giannini C., Burns T.C., Kizilbash S.H., Laramy J.K., Swanson K.R., Kaufmann T.J., Brown P.D., Agar N.Y.R., Galanis E., Buckner J.C., Elmquist W.F. Is the blood-brain barrier really disrupted in all glioblastomas? A critical assessment of existing clinical data. Neuro Oncol 2018; 20(2): 184–191, https://doi.org/10.1093/neuonc/nox175.
- Kang J.H., Adamson C. Novel chemotherapeutics and other therapies for treating high-grade glioma. Expert Opin Investig Drugs 2015; 24(10): 1361–1379, https://doi.org/10.1517/13543784.2015.1048332.
- de Paula L.B., Primo F.L., Tedesco A.C. Nanomedicine associated with photodynamic therapy for glioblastoma treatment. Biophys Rev 2017; 9(5): 761–773, https://doi.org/10.1007/s12551-017-0293-3.
- Di Carlo D.T., Cagnazzo F., Benedetto N., Morganti R., Perrini P. Multiple high-grade gliomas: epidemiology, management, and outcome. A systematic review and meta-analysis. Neurosurg Rev 2019; 42(2): 263–275, https://doi.org/10.1007/s10143-017-0928-7.
- Kaneko S., Fujimoto S., Yamaguchi H., Yamauchi T., Yoshimoto T., Tokuda K. Photodynamic therapy of malignant gliomas. Prog Neurol Surg 2018; 32: 1–13, https://doi.org/10.1159/000469675.
- Li M., Deng H., Peng H., Wang Q. Functional nanoparticles in targeting glioma diagnosis and therapies. J Nanosci Nanotechnol 2014; 14(1): 415–432, https://doi.org/10.1166/jnn.2014.8757.
- Krysko D.V., Garg A.D., Kaczmarek A., Krysko O., Agostinis P., Vandenabeele P. Immunogenic cell death and DAMPs in cancer therapy. Nat Rev Cancer 2012; 12(12): 860–875, https://doi.org/10.1038/nrc3380.
- Halliwell B., Gutteridge J.M.C. Free radicals in biology and medicine. Clarendon Press, Oxford; 2007; 888 p.
- Fingar V.H. Vascular effects of photodynamic therapy. J Clin Laser Med Surg 1996; 14(5): 323–328, https://doi.org/10.1089/clm.1996.14.323.
- Byvaltsev V.A., Stepanov I.A., Kichigin A.I. Outcomes of 5-ALA fliorescece-guided surgery for high grade G. Sibirskij onkologiceskij zurnal 2018; 17(2): 18–26, https://doi.org/10.21294/1814-4861-2018-17-2-18-26.
- Couldwell W.T., Surnock A.A., Tobia A.J., Cabana B.E., Stillerman C.B., Forsyth P.A., Appley A.J., Spence A.M., Hinton D.R., Chen T.C. A phase 1/2 study of orally administered synthetic hypericin for treatment of recurrent malignant gliomas. Cancer 2011; 117(21): 4905–4915, https://doi.org/10.1002/cncr.26123.
- Garg A.D., Krysko D.V., Vandenabeele P., Agostinis P. Extracellular ATP and P2X7 receptor exert context-specific immunogenic effects after immunogenic cancer cell death. Cell Death Dis 2016; 7: e2097, https://doi.org/10.1038/cddis.2015.411.
- Zavadskaya T.S. Photodynamic therapy in the treatment of glioma. Exp Oncol 2015; 37(4): 234–241, https://doi.org/10.31768/2312-8852.2015.37(4):234-241.
- Medyanik I.А., Volovik М.G., Dydykin А.V., Yashin К.S., Kulakova К.V., Bugrov S.N., Karyakin N.N. Intraoperational thermal control of perifocal edema in photodynamic therapy of malignant brain tumors. Sovremennye tehnologii v medicine 2016; 8(3): 82–90, https://doi.org/10.17691/stm2016.8.3.09.
- Vedunova M., Sakharnova T., Mitroshina E., Perminova M., Pimashkin A., Zakharov Y., Dityatev A., Mukhina I. Seizure-like activity in hyaluronidase-treated dissociated hippocampal cultures. Front Cell Neurosci 2013; 7(149), https://doi.org/10.3389/fncel.2013.00149.
- Vedunova M.V., Mishchenko T.A., Mitroshina E.V., Mukhina I.V. TrkB-mediated neuroprotective and antihypoxic properties of brain-derived neurotrophic factor. Oxid Med Cell Longev 2015; 2015: 453901, https://doi.org/10.1155/2015/453901.
- Buytaert E., Dewaele M., Agostinis P. Molecular effectors of multiple cell death pathways initiated by photodynamic therapy. Biochim Biophys Acta 2007; 1776(1): 86–107, https://doi.org/10.1016/j.bbcan.2007.07.001.<
- Agostinis P., Berg K., Cengel K.A., Foster T.H., Girotti A.W., Gollnick S.O., Hahn S.M., Hamblin M.R., Juzeniene A., Kessel D., Korbelik M., Moan J., Mroz P., Nowis D., Piette J., Wilson B.C., Golab J. Photodynamic therapy of cancer: an update. CA Cancer J Clin 2011; 61(4): 250–281, https://doi.org/10.3322/caac.20114.
- Wank M., Schilling D., Schmid T.E., Meyer B., Gempt J., Barz M., Schlegel J., Liesche F., Kessel K.A., Wiestler B., Bette S., Zimmer C., Combs S.E. Human glioma migration and infiltration properties as a target for personalized radiation medicine. Cancers 2018; 10(11): 456, https://doi.org/10.3390/cancers10110456.
- de Leeuw C.N., Vogelbaum M.A. Supratotal resection in glioma: a systematic review. Neuro Oncol 2019; 21(2): 179–188, https://doi.org/10.1093/neuonc/noy166.
- Nicholls J.G., Martin A.R., Wallace B.G., Fuchs P.A. Ot neyrona k mozgu [From neuron to brain]. Moscow: Izd-vo Librokom; 2012; 672 p.
- Singh A., Kukreti R., Saso L., Kukreti S. Oxidative stress: a key modulator in neurodegenerative diseases. Molecules 2019; 24(8): 1583, https://doi.org/10.3390/molecules24081583.
- Márquez J., Alonso F.J., Matés J.M., Segura J.A., Martín-Rufián M., Campos-Sandoval J.A. Glutamine addiction in gliomas. Neurochem Res 2017; 42(6): 1735–1746, https://doi.org/10.1007/s11064-017-2212-1.
- Rivera J.F., Sridharan S.V., Nolan J.K., Miloro S.A., Alam M.A., Rickus J.L., Janes D.B. Real-time characterization of uptake kinetics of glioblastoma vs. astrocytes in 2D cell culture using microelectrode array. Analyst 2018; 143(20): 4954–4966, https://doi.org/10.1039/c8an01198b.
- Brilkina A.A., Dubasova L.V., Balalaeva I.V., Orlova A.G., Sergeeva E.A., Katichev A.R., Shakhova N.M. The study of subcellular distribution of three types of photosensitizers in human cancer cells by laser scanning microscopy. Tekhnologii zhivykh sistem 2011; 8(8): 32–39.
- Castano A.P., Demidova T.N., Hamblin M.R. Mechanisms in photodynamic therapy: part two-cellular signaling, cell metabolism and modes of cell death. Photodiagnosis Photodyn Ther 2005; 2(1): 1–23, https://doi.org/10.1016/s1572-1000(05)00030-x.
- Garg A.D., Krysko D.V., Vandenabeele P., Agostinis P. Hypericin-based photodynamic therapy induces surface exposure of damage-associated molecular patterns like HSP70 and calreticulin. Cancer Immunol Immunother 2012; 61(2): 215–221, https://doi.org/10.1007/s00262-011-1184-2.
- Mishchenko T., Mitroshina E., Balalaeva I., Krysko O., Vedunova M., Krysko D.V. An emerging role for nanomaterials in increasing immunogenicity of cancer cell death. Biochim Biophys Acta Rev Cancer 2019; 1871(1): 99–108, https://doi.org/10.1016/j.bbcan.2018.11.004.
- Li W., Yang J., Luo L., Jiang M., Qin B., Yin H., Zhu C., Yuan X., Zhang J., Luo Z., Du Y., Li Q., Lou Y., Qiu Y., You J. Targeting photodynamic and photothermal therapy to the endoplasmic reticulum enhances immunogenic cancer cell death. Nat Commun 2019; 10(1): 3349, https://doi.org/10.1038/s41467-019-11269-8.
- Hirschberg H., Berg K., Peng Q. Photodynamic therapy mediated immune therapy of brain tumors. Neuroimmunol Neuroinflamm 2018; 5, https://doi.org/10.20517/2347-8659.2018.31.