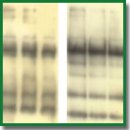
A New Cofilin-Dependent Mechanism for the Regulation of Brain Mitochondria Biogenesis and Degradation
The aim was to study the role of post-translational modifications of cofilin in the regulation of respiration and autophagy in murine brain mitochondria.
Materials and Methods. The experiments were performed with C57BL/6 mice. To obtain cytoplasmic and mitochondrial fractions of the brain tissue, differential centrifugation was used. Expressions of cofilin, phospho-cofilin, K48- and K63-associated chains of ubiquitin, and the autophagy marker LC3B were determined using electrophoresis, immunoprecipitation and Western blot methods. To study the processes of ubiquitination, we used PR619 — the inhibitor of deubiquitinating enzymes. Respiratory activity of brain mitochondria was evaluated using high-resolution fluorespirometry.
Results. Modification of cofilin by non-canonical K63 multiubiquitin chains in the cytoplasm and mitochondria from murine brain was demonstrated. Different levels of phospho-cofilin, cofilin, and its ubiquitinated proteoforms were found. PR619, the inhibitor of deubiquitinating enzymes, affects the expression of phosphorylated and ubiquitinated forms of cofilin in the mitochondria and cytoplasm, at the same time it changes the activity of tissue respiration and mitophagy.
Conclusion. The sensitivity of cofilin to the inhibitor of deubiquitinating enzymes indicates the existence of a new non-catabolic mechanism of cofilin modification, which may be involved in the regulation of mitochondrial functions, specifically, the mitochondrial respiration and autophagy. The data help understand the molecular mechanisms of mitochondrial function in normal and pathological conditions, which may be useful in developing novel methods for the treatment of diseases of the nervous system.
- Ding W.X., Yin X.M. Mitophagy: mechanisms, pathophysiological roles, and analysis. J Biol Chem 2012; 393(7): 547–564, https://doi.org/10.1515/hsz-2012-0119.
- Kubli D.A., Gustafsson A.B. Mitochondria and mitophagy: the yin and yang of cell death control. Circ Res 2012; 111(9): 1208–1221, https://doi.org/10.1161/circresaha.112.265819.
- Suzuki S.W., Onodera J., Ohsumi Y. Starvation induced cell death in autophagy-defective yeast mutants is caused by mitochondria dysfunction. PLoS One 2011; 6(2): e17412, https://doi.org/10.1371/journal.pone.0017412.
- Al-Hakim A., Escribano-Diaz C., Landry M.C., O’Donnell L., Panier S., Szilard R.K., Durocher D. The ubiquitous role of ubiquitin in the DNA damage response. DNA Repair (Amst) 2010; 9(12): 1229–1240, https://doi.org/10.1016/j.dnarep.2010.09.011.
- Popovic D., Vucic D., Dikic I. Ubiquitination in disease pathogenesis and treatment. Nat Med 2014; 20(11): 1242–1253, https://doi.org/10.1038/nm.3739.
- Bragoszewski P., Turek M., Chacinska A. Control of mitochondrial biogenesis and function by the ubiquitin-proteasome system. Open Biol 2017; 7(4): 170007, https://doi.org/10.1098/rsob.170007.
- Ashrafi G., Schlehe J.S., LaVoie M.J., Schwarz T.L. Mitophagy of damaged mitochondria occurs locally in distal neuronal axons and requires PINK1 and Parkin. J Cell Biol 2014; 206(5): 655–670, https://doi.org/10.1083/jcb.201401070.
- Kim K.H., Son J.H. PINK1 gene knockdown leads to increased binding of parkin with actin filament. Neurosci Lett 2010; 468(3): 272–276, https://doi.org/10.1016/j.neulet.2009.11.011.
- Kanellos G., Frame M.C. Cellular functions of the ADF/cofilin family at a glance. J Cell Sci 2016; 129: 3211–3218, https://doi.org/10.1242/jcs.187849.
- Kovaleva T.F., Maksimova N.S., Zhukov I.Y., Pershin V.I., Mukhina I.V., Gainullin M.R. Cofilin: molecular and cellular functions and its role in the functioning of the nervous system. Neurochem J 2019; 13 (1): 11–19, https://doi.org/10.1134/s1819712419010124.
- Kotiadis V.N., Leadsham J.E., Bastow E.L., Gheeraert A., Whybrew J.M., Bard M., Lappalainen P., Gourlay C.W. Identification of new surfaces of cofilin that link mitochondrial function to the control of multi-drug resistance. J Cell Sci 2012; 125(Pt 9): 2288–2299, https://doi.org/10.1242/jcs.099390.
- Palikaras K., Lionaki E., Tavernarakis N. Balancing mitochondrial biogenesis and mitophagy to maintain energy metabolism homeostasis. Cell Death Differ 2015; 22: 1399–1401, https://doi.org/10.1038/cdd.2015.86.
- Li G.B., Zhang H.W., Fu R.Q., Hu X.Y., Liu L., Li Y.N., Liu Y.X., Liu X., Hu J.J., Deng Q., Luo Q.S., Zhang R., Gao N. Mitochondrial fission and mitophagy depend on cofilin-mediated actin depolymerization activity at the mitochondrial fission site. Oncogene 2018; 37(11): 1485–1502, https://doi.org/10.1038/s41388-017-0064-4.
- Madineni A. Role of cofilin, an actin cytoskeletal protein, in ischemic conditions: potential therapeutic target for ischemic stroke [dissertation]. University of Toledo Health Science Campus; 2013.
- Rehklau K., Gurniak C.B., Conrad M., Friauf E., Ott M., Rust M.B. ADF/cofilin proteins translocate to mitochondria during apoptosis but are not generally required for cell death signaling. Cell Death Differ 2012; 19(6): 958–967, https://doi.org/10.1038/cdd.2011.180.
- Gainullin M.R., Zhukov I.Y., Zhou X., Mo Y., Astakhova L., Ernberg I., Matskova L. Degradation of cofilin is regulated by Cbl, AIP4 and Syk resulting in increased migration of LMP2A positive nasopharyngeal carcinoma cells. Sci Rep 2017; 7(1): 9012, https://doi.org/10.1038/s41598-017-09540-3.
- Myeku N., Figueiredo-Pereira M.E. Dynamics of the degradation of ubiquitinated proteins by proteasomes and autophagy: association with sequestosome 1/p62. J Biol Chem 2011; 286(25): 22426–22440, https://doi.org/10.1074/jbc.m110.149252.
- Komander D., Clague M.J., Urbé S. Breaking the chains: structure and function of the deubiquitinases. Nat Rev Mol Cell Biol 2009; 10(8): 550–563, https://doi.org/10.1038/nrm2731.
- Swatek K.N., Komander D. Ubiquitin modifications. Cell Res 2016; 26(4): 399–422, https://doi.org/10.1038/cr.2016.39.
- Seiberlich V., Borchert J., Zhukareva V., Richter-Landsberg C. Inhibition of protein deubiquitination by PR-619 activates the autophagic pathway in OLN-t40 oligodendroglial cells. Cell Biochem Biophys 2013; 67(1): 149–160, https://doi.org/10.1007/s12013-013-9622-8.
- Pershin V., Maksimova N., Mukhina I., Gainullin M., Yudintsev A., Sergeeva T. Effect of the ubiquitin-proteasome system inhibitors on the energy metabolism of nerve cells. FEBS Open Bio 2018; 8 (Suppl S1): 291.