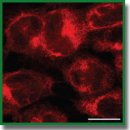
Model of Ovarian Adenocarcinoma Spheroids for Assessing Photodynamic Cytotoxicity
The aim of the study was to compare the relevance of ovarian adenocarcinoma spheroids with that of a monolayer culture for assessing photodynamic effect of the tetrakis(4-benzyloxyphenyl)tetracyanoporphyrazine photosensitizer.
Materials and Methods. The work was performed on SKOV-3 human ovary adenocarcinoma cells grown in vitro in a monolayer culture and in the form of tumor spheroids obtained using culture plates with ultra-low attachment. We determined the photoinduced toxicity of porphyrazine on a monolayer culture using the MTT assay; the effect on the spheroids was tested by assessing the dynamics of their growth. Cellular uptake of porphyrazine was analyzed by confocal microscopy.
Results. Porphyrazine has a pronounced photodynamic effect on SKOV-3 cells. When exposed to light at a dose of 20 J/cm2, the IC50 value 24 h after exposure was 2.3 μM for SKOV-3 monolayer culture. For the spheroids, the effect manifested after a latency period: significant growth retardation of the treated spheroids appeared no sooner than 5 and 9 days after exposure. Notably, no decrease in the initial size of the treated spheroids was observed under any of the photodynamic regimes. The penetration depth of porphyrazine into spheroids was 50–100 μm during 24 h incubation.
Conclusion. The limited penetration of the photosensitizer into the body of spheroids and its predominant accumulation in the surface layers can be one of the key factors behind the significant differences in the photodynamic response between the surface and deep layers of a spheroid. For cells located close to the spheroid surface, the photodynamic effect is comparable to that for a monolayer culture, while in deeper layers, the cells remain viable and support/maintain the growth of the spheroid even under intense photo-exposure. The fact that the in vitro distribution is similar to the inhomogeneous accumulation of photosensitizers in tumors in vivo allows us to consider spheroids more relevant than a monolayer culture for studying photodynamic anti-tumor effects.
- Triesscheijn M., Baas P., Schellens J.H.M., Stewart F.A. Photodynamic therapy in oncology. Oncologist 2006; 11(9): 1034–1044, https://doi.org/10.1634/theoncologist.11-9-1034.
- Hamblin M.R., Mroz P. History of PDT: the first hundred years. In: Hamblin M.R., Mroz P. (editors). Advances in photodynamic therapy: basic, translational and clinical. Norwood, MA: Artech House; 2008; p. 1–12.
- Robertson C.A., Evans D.H., Abrahamse H. Photodynamic therapy (PDT): a short review on cellular mechanisms and cancer research applications for PDT. J Photochem Photobiol B 2009; 96(1): 1–8, https://doi.org/10.1016/j.jphotobiol.2009.04.001.
- Krasnovsky A.A. Luminescence and photochemical studies of singlet oxygen photonics. J Photochem Photobiol A Chem 2008; 196(2): 210–218, https://doi.org/10.1016/j.jphotochem.2007.12.015.
- Brilkina A.A., Peskova N.N., Dudenkova V.V., Gorokhova A.A., Sokolova E.A., Balalaeva I.V. Monitoring of hydrogen peroxide production under photodynamic treatment using protein sensor HyPer. J Photochem Photobiol B 2018; 178: 296–301, https://doi.org/10.1016/j.jphotobiol.2017.11.020.
- Choi Y., Chang J.E., Jheon S., Han S.J., Kim J.K. Enhanced production of reactive oxygen species in HeLa cells under concurrent low-dose carboplatin and Photofrin® photodynamic therapy Oncol Rep 2018; 40(1): 339–345, https://doi.org/10.3892/or.2018.6415.
- Abrahamse H., Hamblin M.R. New photosensitizers for photodynamic therapy. Biochem J 2016; 473(4): 347–364, https://doi.org/10.1042/bj20150942.
- Garland M.J., Cassidy C.M., Woolfson D., Donnelly R.F. Designing photosensitizers for photodynamic therapy: strategies, challenges and promising developments. Future Med Chem 2009; 1(4): 667–691, https://doi.org/10.4155/fmc.09.55.
- Ethirajan M., Chen Y., Joshi P., Pandey R.K. The role of porphyrin chemistry in tumor imaging and photodynamic therapy. Chem Soc Rev 2011; 40(1): 340–362, https://doi.org/10.1002/chin.201117269.
- Ormond A.B., Freeman H.S. Dye sensitizers for photodynamic therapy. Materials 2013; 6(3): 817–840, https://doi.org/10.3390/ma6030817.
- Chilakamarthi U., Giribabu L. Photodynamic therapy: past, present and future. Chem Rec 2017; 17(8): 775–802, https://doi.org/10.1002/tcr.201600121.
- Brilkina A.A., Dubasova L.V., Sergeeva E.A., Pospelov A.J., Shilyagina N.Y., Shakhova N.M., Balalaeva I.V. Photobiological properties of phthalocyanine photosensitizers Photosens, Holosens and Phthalosens: a comparative in vitro analysis. J Photochem Photobiol B 2019; 191: 128–134, https://doi.org/10.1016/j.jphotobiol.2018.12.020.
- Shilyagina N.Y., Peskova N.N., Lermontova S.A., Brilkina A.A., Vodeneev V.A., Yakimansky A.V., Klapshina L.G., Balalaeva I.V. Effective delivery of porphyrazine photosensitizers to cancer cells by polymer brush nanocontainers. J Biophotonics 2017; 10(9): 1189–1197, https://doi.org/10.1002/jbio.201600212.
- Lermontova S.A., Grigor’ev I.S., Ladilina E.Yu., Balalaeva I.V., Shilyagina N.Yu., Klapshina L.G. Porphyrazine structures with acceptor substituents as the basis of materials for photonics and biomedicine. Koordinatsionnaya khimiya 2018; 44(2): 151–166, https://doi.org/10.7868/s0132344x1802007x.
- Haidekker M.A., Theodorakis E.A. Environment-sensitive behavior of fluorescent molecular rotors. J Biol Eng 2010; 4(1): 11, https://doi.org/10.1186/1754-1611-4-11.
- Izquierdo M.A., Vyšniauskas A., Lermontova S.A., Grigoryev I.S., Shilyagina N.Y., Balalaeva I.V., Klapshina L.G., Kuimova M.K. Dual use of porphyrazines as sensitizers and viscosity markers during photodynamic therapy. J Mater Chem B 2015; 3(6): 1089–1096, https://doi.org/10.1039/c4tb01678e.
- Thoma C.R., Zimmermann M., Agarkova I., Kelm J.M., Krek W. 3D cell culture systems modeling tumor growth determinants in cancer target discovery. Adv Drug Deliv Rev 2014; 69–70: 29–41, https://doi.org/10.1016/j.addr.2014.03.001.
- Unger E., Porter T., Lindner J., Grayburn P. Cardiovascular drug delivery with ultrasound and microbubbles. Adv Drug Deliv Rev 2014; 72: 110–126, https://doi.org/10.1016/j.addr.2014.01.012.
- Sokolova E.A., Vodeneev V.A., Deyev S.M., Balalaeva I.V. 3D in vitro models of tumors expressing EGFR family receptors: a potent tool for studying receptor biology and targeted drug development. Drug Discov Today 2019; 24(1): 99–111, https://doi.org/10.1016/j.drudis.2018.09.003.
- Mohammad-Hadi L., MacRobert A.J., Loizidou M., Yaghini E. Photodynamic therapy in 3D cancer models and the utilisation of nanodelivery systems. Nanoscale 2018; 10(4): 1570–1581, https://doi.org/10.1039/c7nr07739d.
- Manoto S.L., Houreld N., Hodgkinson N., Abrahamse H. Modes of cell death induced by photodynamic therapy using zinc phthalocyanine in lung cancer cells grown as a monolayer and three-dimensional multicellular spheroids. Molecules 2017; 22(5): 791, https://doi.org/10.3390/molecules22050791.
- Khot M.I., Perry S.L., Maisey T., Armstrong G., Andrew H., Hughes T.A., Kapur N., Jayne D.G. Inhibiting ABCG2 could potentially enhance the efficacy of hypericin-mediated photodynamic therapy in spheroidal cell models of colorectal cancer. Photodiagnosis Photodyn Ther 2018; 23: 221–229, https://doi.org/10.1016/j.pdpdt.2018.06.027.
- Balalaeva I.V., Sokolova E.A., Puzhikhina A.D., Brilkina A.A., Deyev S.M. Spheroids of HER2-positive breast adenocarcinoma for studying anticancer immunotoxins in vitro. Acta Naturae 2017; 9(1): 38–43, https://doi.org/10.32607/20758251-2017-9-1-38-43.
- Lermontova S.A., Grigor’ev I.S., Ladilina E.Y., Klapshina L.G., Peskova N.N., Balalaeva I.V., Boyarskii V.P. New promising porphyrazine-based agents for optical theranostics of cancer. Russ J Gen Chem 2017; 87(3): 479–484, https://doi.org/10.1134/s1070363217030173.
- Shilyagina N.Y., Plekhanov V.I., Shkunov I.V., Shilyagin P.А., Dubasova L.V., Brilkina А.А., Sokolova E.A., Turchin I.V., Balalaeva I.V. LED light source for in vitro study of photosensitizing agents for photodynamic therapy. Sovremennye tehnologii v medicine 2014; 6(2): 15–24.
- Mosmann T. Rapid colorimetric assay for cellular growth and survival: application to proliferation and cytotoxicity assays. J Immunol Methods 1983; 65(1–2): 55–63, https://doi.org/10.1016/0022-1759(83)90303-4.
- Yudintsev A.V., Shilyagina N.Yu., Dyakova D.V., Lermontova S.A., Klapshina L.G., Guryev E.L., Balalaeva I.V., Vodeneev V.A. Liposomal form of tetra(aryl)tetracyanoporphyrazine: physical properties and photodynamic activity in vitro. J Fluoresc 2018; 28(2): 513–522, https://doi.org/10.1007/s10895-018-2212-9.
- Huang B.W., Gao J.Q. Application of 3D cultured multicellular spheroid tumor models in tumor-targeted drug delivery system research. J Control Release 2018; 270: 246–259, https://doi.org/10.1016/j.jconrel.2017.12.005.
- Zhang D.Y., Zheng Y., Zhang H., He L., Tan C.P., Sun J.H., Zhang W., Peng X., Zhan Q., Ji L.N., Mao Z.W. Ruthenium complex-modified carbon nanodots for lysosome-targeted one- and two-photon imaging and photodynamic therapy. Nanoscale 2017; 9(47): 18966–18976, https://doi.org/10.1039/c7nr05349e.
- Kumari P., Rompicharla S.V.K., Bhatt H., Ghosh B., Biswas S. Development of chlorin e6-conjugated poly(ethylene glycol)-poly(d,l-lactide) nanoparticles for photodynamic therapy. Nanomedicine (Lond) 2019; 14(7): 819–834, https://doi.org/10.2217/nnm-2018-0255.
- Rizvi I., Celli J.P., Evans C.L., Abu-Yousif A.O., Muzikansky A., Pogue B.W., Finkelstein D., Hasan T. Synergistic enhancement of carboplatin efficacy with photodynamic therapy in a three-dimensional model for micrometastatic ovarian cancer. Cancer Res 2010; 70(22): 9319–9328, https://doi.org/10.1158/0008-5472.can-10-1783.
- Millard M., Yakavets I., Piffoux M., Brun A., Gazeau F., Guigner J.M., Jasniewski J., Lassalle H.P., Wilhelm C., Bezdetnaya L. mTHPC-loaded extracellular vesicles outperform liposomal and free mTHPC formulations by an increased stability, drug delivery efficiency and cytotoxic effect in tridimensional model of tumors. Drug Deliv 2018; 25(1): 1790–1801, https://doi.org/10.1080/10717544.2018.1513609.
- Choi I.K., Strauss R., Richter M., Yun C.O., Lieber A. Strategies to increase drug penetration in solid tumors. Front Oncol 2013; 3: 193, https://doi.org/10.3389/fonc.2013.00193.
- Namazi H., Kulish V.V., Wong A., Nazeri S. Mathematical based calculation of drug penetration depth in solid tumors. Biomed Res Int 2016; 2016: 8437247, https://doi.org/10.1155/2016/8437247.
- Yakavets I., Lassalle H.P., Scheglmann D., Wiehe A., Zorin V., Bezdetnaya L. Temoporfin-in-cyclodextrin-in-liposome — a new approach for anticancer drug delivery: the optimization of composition. Nanomaterials (Basel) 2018; 8(10): 847, https://doi.org/10.3390/nano8100847.
- Castano A.P., Demidova T.N., Hamblin M.R. Mechanisms in photodynamic therapy: part three — photosensitizer pharmacokinetics, biodistribution, tumor localization and modes of tumor destruction. Photodiagnosis Photodyn Ther 2005; 2(2): 91–106, https://doi.org/10.1016/s1572-1000(05)00060-8.
- Lee J., Kim J., Jeong M., Lee H., Goh U., Kim H., Kim B., Park J.H. Liposome-based engineering of cells to package hydrophobic compounds in membrane vesicles for tumor penetration. Nano Lett 2015; 15(5): 2938–2944, https://doi.org/10.1021/nl5047494.