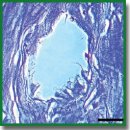
Development of a Two-Layer Porous Scaffold Based on Porcine Nasal Septal Cartilage for Orthopedics
The aim of the study was to design a construct based on a nasal septal cartilage plate providing required cell differentiation in different layers to replace a deep osteochondral defect and develop an algorithm of chemical and physical effect sequence to create non-immunogenic two-layer porous structure with requisite elasto-mechanical properties.
Materials and Methods. The plates derived from porcine nasal septal hyaline cartilage covered by perichondrium were multi-stage treated including freezing, equilibrating in a hypotonic saline solution (type I specimens); trypsinization, point IR-laser effect, re-trypsinization (type II specimens); a stabilizing effect of crosslinking agents — glyceraldehyde/ribose in an acidic medium — washing (type III specimens).
For all type specimens:
1) there were established stability parameters (collagen denaturation temperature using a thermal analysis; and Young’s modulus using a mechanical analysis);
2) there were determined morphological characteristics using light and polarization microscopy with classical staining and nonlinear optical microscopy in second-harmonic generation mode.
Results. Thermal, mechanical, and morphological properties in type I specimens slightly differed from those of the initial nasoseptal system. A considerable part of cells had destroyed membranes.
In type II specimens, thermal stability of collagen frame was significantly lower; Young’s modulus decreased more than fourfold compared to type I specimens. Collagen structure of hyaline cartilage appeared to be disarranged, although the morphological differences of the hyaline part and perichondrium preserved. The construct matrix was almost completely decellularized. Successive exposure to laser radiation and trypsin resulted in the formation of partial holes in the matrix, ~100 µm in diameter.
In type III specimens, both the thermal stability of the collagen frame and Young’s modulus (E) increased. Glyceraldehyde was more effective than ribose, E having reached the value typical for intact hyaline cartilage. Collagen fibers in type III specimens were thicker than in type I and II specimens. The morphological differences of the hyaline part and perichondrium and partial holes were preserved.
Conclusion. Due to sequential treatment by salts, trypsin, IR-laser radiation, and nontoxic crosslinking agents, nasal septal cartilage plate forms porous acellular construction consisting of two layers formed by type I (from perichondrium) and type II (from hyaline part) collagen fibers. In the present construction, stability, mechanical properties, and size of the partial holes can be assigned for cell colonization. It enables to use the construction to replace articular cartilage defects.
- Cheng A., Schwartz Z., Kahn A., Li X., Shao Z., Sun M., Ao Y., Boyan B.D., Chen H. Advances in porous scaffold design for bone and cartilage tissue engineering and regeneration. Tissue Eng Part B Rev 2019; 25(1): 14–29, https://doi.org/10.1089/ten.teb.2018.0119.
- Wang M., Yuan Z., Ma N., Hao C., Guo W., Zou G., Zhang Y., Chen M., Gao S., Peng J., Wang A., Wang Y., Sui X., Xu W., Lu S., Liu S., Guo Q. Advances and prospects in stem cells for cartilage regeneration. Stem Cells Int 2017; 2017: 4130607, https://doi.org/10.1155/2017/4130607.
- Izadifar Z., Chen X., Kulyk W. Strategic design and fabrication of engineered scaffolds for articular cartilage repair. J Funct Biomater 2012; 3(4): 799–838, https://doi.org/10.3390/jfb3040799.
- Cheng C.W., Solorio L.D., Alsberg E. Decellularized tissue and cell-derived extracellular matrices as scaffolds for orthopaedic tissue engineering. Biotechnol Adv 2014; 32(2): 462–484, https://doi.org/10.1016/j.biotechadv.2013.12.012.
- Yang Q., Peng J., Guo Q., Huang J., Zhang L., Yao J., Yang F., Wang S., Xu W., Wang A., Lu S. A cartilage ECM-derived 3-D porous acellular matrix scaffold for in vivo cartilage tissue engineering with PKH26-labeled chondrogenic bone marrow-derived mesenchymal stem cells. Biomaterials 2008; 29(15): 2378–2387, https://doi.org/10.1016/j.biomaterials.2008.01.037.
- Zhang Y., Liu S., Guo W., Wang M., Hao C., Gao S., Zhang X., Li X., Chen M., Jing X., Wang Z., Peng J., Lu S., Guo Q. Human umbilical cord Wharton’s jelly mesenchymal stem cells combined with an acellular cartilage extracellular matrix scaffold improve cartilage repair compared with microfracture in a caprine model. Osteoarthritis Cartilage 2018; 26(7): 954–965, https://doi.org/10.1016/j.joca.2018.01.019.
- Graham M.E., Gratzer P.F., Bezuhly M., Hong P. Development and characterization of decellularized human nasoseptal cartilage matrix for use in tissue engineering. Laryngoscope 2016; 126(10): 2226–2231, https://doi.org/10.1002/lary.25884.
- Jia S., Zhang T., Xiong Z., Pan W., Lui J., Sun W. In vivo evaluation of a novel oriented scaffold-BMSC construct for enhancing full-thickness articular cartilage repair in a rabbit model. PLoS One 2015; 10(12): e0145667, https://doi.org/10.1371/journal.pone.0145667.
- Luo R., Eswaramoorthy K.J., Mulhall D.J., Kelly D.J. Decellularization of porcine articular cartilage explants and their subsequent repopulation with human chondroprogenitor cells. J Mech Behav Biomed Mater 2015; 55: 21–31, https://doi.org/10.1016/j.jmbbm.2015.10.002.
- Bowland P., Ingham E., Jennings L., Fisher J. Review of the biomechanics and biotribology of osteochondral grafts used for surgical interventions in the knee. Proc Inst Mech Eng H 2015; 229(12): 879–888, https://doi.org/10.1177/0954411915615470.
- Wang K.H., Wan R., Chiu L.H., Tsai Y.H., Fang C.L., Bowley J.F., Chen K.C., Shih H.N., Lai W.T. Effects of collagen matrix and bioreactor cultivation on cartilage regeneration of a fullthickness critical-size knee joint cartilage defects with subchondral bone damage in a rabbit model. PLoS One 2018; 13(5): e0196779, https://doi.org/10.1371/journal.pone.0196779.
- Rowland R., Colucci L.A., Guilak F. Fabrication of anatomically-shaped cartilage constructs using decellularized cartilage-derived matrix scaffolds. Biomaterials 2016; 91: 57–72, https://doi.org/10.1016/j.biomaterials.2016.03.012.
- Baranovsky D.S., Demchenko A.G., Oganesyan R.V., Lebedev G.V., Berseneva D.A., Balyasin M.V., Parshin V.D., Lyundup A.V. Acellular tracheal cartilaginous scaffold producing for tissue-engineered constructs. Vestnik Rossiiskoi akademii medetsinskikh nauk 2017; 72(4): 254–260, https://doi.org/10.15690/vramn723.
- Rowland C.R., Glass C.A., Ettyreddy A.R., Gloss C.C., Matthews J.R.L., Huynh N.P.T., Guilak F. Regulation of decellularized tissue remodeling via scaffold-mediated lentiviral delivery in anatomically-shaped osteochondral constructs. Biomaterials 2018; 177: 161–175, https://doi.org/10.1016/j.biomaterials.2018.04.049.
- Yang Q., Peng J., Lu S.B., Guo Q.Y., Zhao B., Zhang L., Wang A.Y., Xu W.J., Xia Q., Ma X.L., Hu Y.C., Xu B.S. Evaluation of an extracellular matrix-derived acellular biphasic scaffold/cell construct in the repair of a large articular high-load-bearing osteochondral defect in a canine model. Chin Med J (Engl) 2011; 124(23): 3930–3938.
- Hernigou J., Vertongen P., Chahidi E., Kyriakidis T., Dehoux J.P., Crutzen M., Boutry S., Larbanoix L., Houben S., Gaspard N., Koulalis D., Rasschaert J. Effects of press-fit biphasic (collagen and HA/βTCP) scaffold with cell-based therapy on cartilage and subchondral bone repair knee defect in rabbits. Intern Orthop 2018; 42(7): 1755–1767, https://doi.org/10.1007/s00264-018-3999-3.
- Bernhardt A., Paul B., Gelinsky M. Biphasic scaffolds from marine collagens for regeneration of osteochondral defects. Mar Drugs 2018; 16(3): 91–97, https://doi.org/10.3390/md16030091.
- Xu Y., Guo X., Yang Sh., Li L., Zhang P., Sun W., Liu C., Mi S. Construction of bionic tissue engineering cartilage scaffold based on three-dimensional printing and oriented frozen technology. J Biomed Mater Res Part A 2018; 106(6): 1664–1676, https://doi.org/10.1002/jbm.a.36368.
- Zakharkina O.L., Sergeeva E.A., Kirillin M.Yu., Ignatieva N.Y. Analysis of laser-induced modification of collagen structure using nonlinear optical microscopy. Quantum Electron 2020; 50(1): 76–80, https://doi.org/10.1070/qel17214.
- Sviridov A.P., Zakharkina O.L., Ignatieva N.Y., Vorobieva N.N., Bagratashvili N.V., Plyakin V.A., Kulik I.O., Sarukhanyan O.O., Minaev V.P., Lunin V.V., Bagratashvili V.N. Ex vivo laser thermoplasty of whole costal cartilages. Lasers Surg Med 2014; 46(4): 302–309, https://doi.org/10.1002/lsm.22233.
- Ignatieva N.Yu., Lunin V.V., Averkiev S.V., Maiorova A.F., Bagratashvili V.N., Sobol E.N. DSC investigation of connective tissues treated by IR-laser radiation. Thermochim Acta 2004; 422(1–2): 43–48, https://doi.org/10.1016/j.tca.2004.04.030.
- Lazernaya inzheneriya khryashchey [Cartilage laser engineering]. Bagratashvili V.N., Sobol E.N., Shekhter A.B. (editors). Moscow: FIZMATLIT; 2006.
- Schmidt M.B., Mow V.C., Chun L.E., Eyre D.R. Effects of proteoglycan extraction on the tensile behavior of articular cartilage. J Orthop Res 1990; 8(3): 353–363, https://doi.org/10.1002/jor.1100080307.
- Bailey A.J., Paul R.G., Knott L. Mechanisms of maturation and ageing of collagen. Mech Ageing Dev 1998; 106(1–2): 1–56, https://doi.org/10.1016/s0047-6374(98)00119-5.
- Danilov N.A., Ignatieva N.Yu., Iomdina E.N., Semenova S.A., Rudenskaya G.N., Grokhovskaya T.E., Lunin V.V. Stabilization of scleral collagen by glycerol aldehyde cross-linking. Biochim Biophys Acta 2008; 1780(5): 764–772, https://doi.org/10.1016/j.bbagen.2008.01.014.
- Sung H.W., Chang Y., Chiu C.T., Chen C.N., Liang H.C. Crosslinking characteristics and mechanical properties of a bovine pericardium fixed with a naturally occurring crosslinking agent. J Biomed Mater Res 1999; 47(2): 116–126, https://doi.org/10.1002/(sici)1097-4636(199911)47:2116::aid-jbm23.0.co;2-j.
- Bai P., Phua K., Hardt T., Cernadas M., Brodsky B. Glycation alters collagen fibril organization. Connect Tissue Res 1992; 28(1–2): 1–12, https://doi.org/10.3109/03008209209014224.
- Lee K.W., Simpson G., Ortwerth B. A systematic approach to evaluate the modification of lens proteins by glycation-induced crosslinking. Biochim Biophys Acta 1999; 1453(1): 141–151, https://doi.org/10.1016/s0925-4439(98)00097-0.