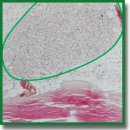
Selection of the Optimal Method for Creating Various Forms of Biocompatible Xenodermal Materials
The aim of the study was to select the optimal method for creating surgical porcine dermis-based biomaterials and to assess their biological safety.
Materials and Methods. To create xenodermal biomaterials, the native skin of a 4-month-old Landrace pig was used. The porcine dermis was processed with saline (protocol No.1), peroxide-alkaline (protocol No.2), and alkaline (protocol No.3) solutions. The obtained samples were stained with hematoxylin-eosin and a DAPI fluorescent dye. Quantitative DNA analysis and assessment of cytotoxicity by the LIVE/DEAD assay were also performed. Samples were implanted/injected subcutaneously to 6-month-old male Wistar rats (n=30) weighing 260±20 g and explanted on day 14 of the experiment. Histological sections were stained with hematoxylin-eosin. Computer morphometry was performed using GraphPad Prism v. 6.04.
Results. Samples of surgical materials obtained according to the three protocols had different physical characteristics: dermis treated according to protocol No.1 was dense and white in color after processing; samples processed by protocol No.2 were transparent and dense, and samples treated according to protocol No.3 had transparent gel-like structures. Histological analysis has shown oxyphilicity and extracellular matrix structure loss in all samples, and DAPI staining has revealed the destruction of cell nuclei. Nevertheless, DNA amount in the samples processed according to protocol No.1 did not meet the established quality criterion for decellularization (50 ng/mg dry weight). Further cytotoxicity assessment in vitro and in vivo was carried out only for samples fabricated according to protocols No.2 and No.3. According to the LIVE/DEAD analysis, both samples were not cytotoxic. On day 14 after the subcutaneous sample implantation, no signs of suppuration and immune rejection were found in the animals.
Conclusion. To obtain surgical materials in the form of bioplastic coatings, it is recommended to use alkaline-peroxide treatment of the dermis, while hydrogel coatings are produced by alkaline hydrolysis.
- Brocke T., Barr J. The history of wound healing. Surg Clin North Am 2020; 100(4): 787–806, https://doi.org/10.1016/j.suc.2020.04.004.
- Ushmarov D.I., Gumenyuk S.E., Gumenyuk A.S., Gayvoronskaya T.V., Karablina S.Y., Pomortsev А.V., Sotnichenko A.S., Melkonyan K.I., Grigoriev T.Е. Comparative evaluation of chitosan-based multifunctional wound dressings: a multistage randomised controlled experimental trial. Kubanskii nauchnyi meditsinskii vestnik 2021; 28(3): 78–96, https://doi.org/10.25207/1608-6228-2021-28-3-78-96.
- Chattopadhyay S., Raines R.T. Collagen-based biomaterials for wound healing. Biopolymers 2014; 101(8): 821–833, https://doi.org/10.1002/bip.22486.
- Choi J.S., Oh S.H., Kim Y.M., Lim J.Y. Hyaluronic acid/alginate hydrogel containing hepatocyte growth factor and promotion of vocal fold wound healing. Tissue Eng Regen Med 2020; 17(5): 651–658, https://doi.org/10.1007/s13770-020-00280-6.
- Li R., Xu J., Rao Z., Deng R., Xu Y., Qiu S., Long H., Zhu Q., Liu X., Bai Y., Quan D. Facilitate angiogenesis and neurogenesis by growth factors integrated decellularized matrix hydrogel. Tissue Eng Part A 2021; 27(11–12): 771–787, https://doi.org/10.1089/ten.tea.2020.0227.
- Kuznetsova T.A., Besednova N.N., Usov V.V., Andryukov B.G. Biocompatible and biodegradable wound dressings on the basis of seaweed polysaccharides (review of literature). Vestnik khirurgii im. I.I. Grekova 2020; 179(4): 109–115, https://doi.org/10.24884/0042-4625-2020-179-4-109-115.
- Shekhter A.B., Guller A.E., Istranov L.P., Istranova E.V., Butnaru D.V., Vinarov A.Z., Zakharkina O.L., Kurkov A.V., Kantimerov D.F., Antonov E.N., Marisov L.V., Glybochko P.V. Morphology of collagen matrices for tissue engineering (biocompatibility, biodegradation, tissue response). Arhiv patologii 2015; 77(6): 29–38, https://doi.org/10.17116/patol201577629-38.
- Hussey G.S., Dziki J.L., Badylak S.F. Extracellular matrix-based materials for regenerative medicine. Nat Rev Mater 2018; 3(7): 159–173, https://doi.org/10.1038/s41578-018-0023-x.
- Keane T.J., Badylak S.F. The host response to allogeneic and xenogeneic biological scaffold materials. J Tissue Eng Regen Med 2015; 9(5): 504–511, https://doi.org/10.1002/term.1874.
- Ivanov A.N., Saveleva M.S., Kozadaev M.N., Matveeva O.V., Salkovskiy Yu.E., Lyubun G.P., Gorin D.A., Norkin I.A. New approaches to scaffold biocompatibility assessment. BioNanoScience 2019; 9(2): 395–405, https://doi.org/10.1007/s12668-019-00613-3.
- Terzini M., Bignardi C., Castagnoli C., Cambieri I., Zanetti E.M., Audenino A.L. Ex vivo dermis mechanical behavior in relation to decellularization treatment length. Open Biomed Eng J 2016; 10: 34–42, https://doi.org/10.2174/1874120701610010034.
- Saghizadeh M., Winkler M.A., Kramerov A.A., Hemmati D.M., Ghiam C.A., Dimitrijevich S.D., Sareen D., Ornelas L., Ghiasi H., Brunken W.J., Maguen E., Rabinowitz Y.S., Svendsen C.N., Jirsova K., Ljubimov A.V. A simple alkaline method for decellularizing human amniotic membrane for cell culture. PloS One 2013; 8(11): e79632, https://doi.org/10.1371/journal.pone.0079632.
- Rieder E., Steinacher-Nigisch A., Weigel G. Human immune-cell response towards diverse xenogeneic and allogeneic decellularized biomaterials. Int J Surg 2016; 36(Pt A): 347–351, https://doi.org/10.1016/j.ijsu.2016.06.042.
- Rodrigues F.T., Martins V.C.A., Plepis A.M.G. Porcine skin as a source of biodegradable matrices: alkaline treatment and glutaraldehyde crosslinking. Polímeros 2010; 20(2): 92–97, https://doi.org/10.1590/s0104-14282010005000013.
- Antipova L.V., Storublevtsev S.A. Method for producing collagen base with aseptic properties. Patent RU 2739396. 2020.
- Crapo P.M., Gilbert T.W., Badylak S.F. An overview of tissue and whole organ decellularization processes. Biomaterials 2011; 32(12): 3233–3243, https://doi.org/10.1016/j.biomaterials.2011.01.057.
- Lange P., Greco K., Partington L., Carvalho C., Oliani S., Birchall M.A., Sibbons P.D., Lowdell M.W., Ansari T. Pilot study of a novel vacuum-assisted method for decellularization of tracheae for clinical tissue engineering applications. J Tissue Eng Regen Med 2017; 11(3): 800–811, https://doi.org/10.1002/term.1979.
- Keane T.J., Swinehart I., Badylak S.F. Methods of tissue decellularization used for preparation of biologic scaffolds and in vivo relevance. Methods 2015; 84: 25–34, https://doi.org/10.1016/j.ymeth.2015.03.005.
- Li D., Sun W.Q., Wang T., Gao Y., Wu J., Xie Z., Zhao J., He C., Zhu M., Zhang S., Wang P., Mo X. Evaluation of a novel tilapia-skin acellular dermis matrix rationally processed for enhanced wound healing. Mater Sci Eng C Mater Biol Appl 2021; 127: 112202, https://doi.org/10.1016/j.msec.2021.112202.
- Hoganson D.M., O’Doherty E.M., Owens G.E., Harilal D.O., Goldman S.M., Bowley C.M., Neville C.M., Kronengold R.T., Vacanti J.P. The retention of extracellular matrix proteins and angiogenic and mitogenic cytokines in a decellularized porcine dermis. Biomaterials 2010; 31(26): 6730–6737, https://doi.org/10.1016/j.biomaterials.2010.05.019.
- Gilbert T.W., Freund J.M., Badylak S.F. Quantification of DNA in biologic scaffold materials. J Surg Res 2009; 152(1): 135–139, https://doi.org/10.1016/j.jss.2008.02.013.
- Leow-Dyke S.F., Rooney P., Kearney J.N. Evaluation of copper and hydrogen peroxide treatments on the biology, biomechanics, and cytotoxicity of decellularized dermal allografts. Tissue Eng Part C Methods 2016; 22(3): 290–300, https://doi.org/10.1089/ten.tec.2015.0271.
- Bishtakov R.B., Garifullina R.A., Sadykova G.U., Lomakin S.P. About collagen and hydrolysates of derma collagen. Stolica nauki 2021; 2: 23–27. URL: https://ftp.scientific-capital.ru/feb2021/0022021.pdf#page=24.
- Schwarz S., Koerber L., Elsaesser A.F., Goldberg-Bockhorn E., Seitz A.M., Dürselen L., Ignatius A., Walther P., Breiter R., Rotter N. Decellularized cartilage matrix as a novel biomatrix for cartilage tissue-engineering applications. Tissue Eng Part A 2012; 18(21–22): 2195–2209, https://doi.org/10.1089/ten.tea.2011.0705.
- Kalmykova N.V., Demyanenko I.A., Shevlyagina N.V., Andreevskaya S.G., Suslov A.P. The comparative efficiency analysis of simple and multicomponent alkaline decellularization on the example of purification of the fibrous extracellular matrix of the derma. Morfologicheskie vedomosti 2016; 24(4): 36–45.
- Fernández-Pérez J., Ahearne M. The impact of decellularization methods on extracellular matrix derived hydrogels. Sci Rep 2019; 9(1): 14933, https://doi.org/10.1038/s41598-019-49575-2.
- Bordbar S., Lotfi Bakhshaiesh N., Khanmohammadi M., Sayahpour F.A., Alini M., Baghaban Eslaminejad M. Production and evaluation of decellularized extracellulеar matrix hydrogel for cartilage regeneration derived from knee cartilage. J Biomed Mater Res A 2020; 108(4): 938–946, https://doi.org/10.1002/jbm.a.36871.