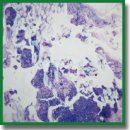
Effect of Collagen and GelMA on Preservation of the Costal Chondrocytes’ Phenotype in a Scaffold in vivo
The aim of the study was to compare type I collagen-based and methacryloyl gelatin-based (GelMA) hydrogels by their ability to form hyaline cartilage in animals after subcutaneous implantation of scaffolds.
Materials and Methods. Chondrocytes were isolated from the costal cartilage of newborn rats using 0.15% collagenase solution in DMEM. The cells was characterized by glycosaminoglycan staining with alcian blue. Chondrocyte scaffolds were obtained from 4% type I porcine atelocollagen and 10% GelMA by micromolding and then implanted subcutaneously into the withers of two groups of Wistar rats. Histological and immunohistochemical studies were performed on days 12 and 26 after implantation. Tissue samples were stained with hematoxylin and eosin, alcian blue; type I and type II collagens were identified by the corresponding antibodies.
Results. The implanted scaffolds induced a moderate inflammatory response in both groups when implanted in animals. By day 26 after implantation, both collagen and GelMA had almost completely resorbed. Cartilage tissue formation was observed in both animal groups. The newly formed tissue was stained intensively with alcian blue, and the cells were positive for both types of collagen. Cartilage tissue was formed among muscle fibers.
Conclusion. The ability of collagen type I and GelMA hydrogels to form hyaline cartilage in animals after subcutaneous implantation of scaffolds was studied. Both collagen and GelMA contributed to formation of hyaline-like cartilage tissue type in animals, but the chondrocyte phenotype is characterized as mixed. Additional detailed studies of possible mechanisms of chondrogenesis under the influence of each of the hydrogels are needed.
- Schneider M.C., Chu S., Randolph M.A., Bryant S.J. An in vitro and in vivo comparison of cartilage growth in chondrocyte-laden matrix metalloproteinase-sensitive poly (ethylene glycol) hydrogels with localized transforming growth factor β3. Acta Biomater 2019; 93: 97–110, https://doi.org/10.1016/j.actbio.2019.03.046.
- Sophia Fox A.J., Bedi A., Rodeo S.A. The basic science of articular cartilage: structure, composition, and function. Sports Health 2009; 1(6): 461–468, https://doi.org/10.1177/1941738109350438.
- Daly A.C., Critchley S.E., Rencsok E.M., Kelly D.J. A comparison of different bioinks for 3D bioprinting of fibrocartilage and hyaline cartilage. Biofabrication 2016; 8(4): 045002, https://doi.org/10.1088/1758-5090/8/4/045002.
- Davies R.L., Kuiper N.J. Regenerative medicine: a review of the evolution of autologous chondrocyte implantation (ACI) therapy. Bioengineering (Basel) 2019; 6(1): 22, https://doi.org/10.3390/bioengineering6010022.
- Kalson N.S., Gikas P.D., Briggs T.W.R. Current strategies for knee cartilage repair. Int J Clin Pract 2010; 64(10): 1444–1452, https://doi.org/10.1111/j.1742-1241.2010.02420.x.
- Brittberg M., Recker D., Ilgenfritz J., Saris D.B.F.; SUMMIT Extension Study Group. Matrix-applied characterized autologous cultured chondrocytes versus microfracture: five-year follow-up of a prospective randomized trial. Am J Sports Med 2018; 46(6): 1343–1351, https://doi.org/10.1177/0363546518756976.
- Marlovits S., Hombauer M., Truppe M., Vècsei V., Schlegel W. Changes in the ratio of type-I and type-II collagen expression during monolayer culture of human chondrocytes. J Bone Joint Surg Br 2004; 86(2): 286–295, https://doi.org/10.1302/0301-620x.86b2.14918.
- Schlegel W., Nürnberger S., Hombauer M., Albrecht C., Vécsei V., Marlovits S. Scaffold-dependent differentiation of human articular chondrocytes. Int J Mol Med 2008; 22(5): 691–699, https://doi.org/10.3892/ijmm_00000074.
- Mu L., Zeng J., Huang Y., Lin Y., Jiang H., Teng L. Experimental study on tissue engineered cartilage constructed by three-dimensional bioprinted human adipose-derived stem cells combined with gelatin methacryloyl. Zhongguo Xiu Fu Chong Jian Wai Ke Za Zhi 2021; 35(7): 896–903, https://doi.org/10.7507/1002-1892.202101049.
- Annabi N., Tamayol A., Uquillas J.A., Akbari M., Bertassoni L.E., Cha C., Camci-Unal G., Dokmeci M.R., Peppas N.A., Khademhosseini A. 25th anniversary article: rational design and applications of hydrogels in regenerative medicine. Adv Mater 2014; 26(1): 85–123, https://doi.org/10.1002/adma.201303233.
- Dzobo K., Motaung K.S.C.M., Adesida A. Recent trends in decellularized extracellular matrix bioinks for 3D printing: an updated review. Int J Mol Sci 2019; 20(18): 4628, https://doi.org/10.3390/ijms20184628.
- Perrier-Groult E., Pérès E., Pasdeloup M., Gazzolo L., Duc Dodon M., Mallein-Gerin F. Evaluation of the biocompatibility and stability of allogeneic tissue-engineered cartilage in humanized mice. PLoS One 2019; 14(5): e0217183, https://doi.org/10.1371/journal.pone.0217183.
- Benayahu D., Pomeraniec L., Shemesh S., Heller S., Rosenthal Y., Rath-Wolfson L., Benayahu Y. Biocompatibility of a marine collagen-based scaffold in vitro and in vivo. Mar Drugs 2020; 18(8): 420, https://doi.org/10.3390/md18080420.
- Campos F., Bonhome-Espinosa A.B., Chato-Astrain J., Sánchez-Porras D., García-García Ó.D., Carmona R., López-López M.T., Alaminos M., Carriel V., Rodriguez I.A. Evaluation of fibrin-agarose tissue-like hydrogels biocompatibility for tissue engineering applications. Front Bioeng Biotechnol 2020; 8: 596, https://doi.org/10.3389/fbioe.2020.00596.
- Levett P.A., Melchels F.P., Schrobback K., Hutmacher D.W., Malda J., Klein T.J. Chondrocyte redifferentiation and construct mechanical property development in single-component photocrosslinkable hydrogels. J Biomed Mater Res A 2014; 102(8): 2544–2553, https://doi.org/10.1002/jbm.a.34924.
- Thitiset T., Damrongsakkul S., Bunaprasert T., Leeanansaksiri W., Honsawek S. Development of collagen/demineralized bone powder scaffolds and periosteum-derived cells for bone tissue engineering application. Int J Mol Sci 2013; 14(1): 2056–2071, https://doi.org/10.3390/ijms14012056.
- Chang C.H., Chen C.C., Liao C.H., Lin F.H., Hsu Y.M., Fang H.W. Human acellular cartilage matrix powders as a biological scaffold for cartilage tissue engineering with synovium-derived mesenchymal stem cells. J Biomed Mater Res A 2014; 102(7): 2248–2257, https://doi.org/10.1002/jbm.a.34897.
- Lee H.J., Kim Y.B., Ahn S.H., Lee J.S., Jang C.H., Yoon H., Chun W., Kim G.H. A new approach for fabricating collagen/ECM-based bioinks using preosteoblasts and human adipose stem cells. Adv Healthc Mater 2015; 4(9): 1359–1368, https://doi.org/10.1002/adhm.201500193.
- Yang X., Lu Z., Wu H., Li W., Zheng L., Zhao J. Collagen-alginate as bioink for three-dimensional (3D) cell printing based cartilage tissue engineering. Mater Sci Eng C Mater Biol Appl 2018; 83: 195–201, https://doi.org/10.1016/j.msec.2017.09.002.
- Beketov E.E., Isaeva E.V., Yakovleva N.D., Demyashkin G.A., Arguchinskaya N.V., Kisel A.A., Lagoda T.S., Malakhov E.P., Kharlov V.I., Osidak E.O., Domogatsky S.P., Ivanov S.A., Shegay P.V., Kaprin A.D. Bioprinting of cartilage with bioink based on high-concentration collagen and chondrocytes. Int J Mol Sci 2021 22(21): 11351, https://doi.org/10.3390/ijms222111351.
- Isaeva E.V., Beketov E.E., Demyashkin G.A., Yakovleva N.D., Arguchinskaya N.V., Kisel A.A., Lagoda T.S., Malakhov E.P., Smirnova A.N., Petriev V.M., Eremin P.S., Osidak E.O., Domogatsky S.P., Ivanov S.A., Shegay P.V., Kaprin A.D. Cartilage formation in vivo using high concentration collagen-based bioink with MSC and decellularized ECM granules. Int J Mol Sci 2022; 23(5): 2703, https://doi.org/10.3390/ijms23052703.
- Grogan S.P., Chung P.H., Soman P., Chen P., Lotz M.K., Chen S., D’Lima D.D. Digital micromirror device projection printing system for meniscus tissue engineering. Acta Biomater 2013; 9(7): 7218–7226, https://doi.org/10.1016/j.actbio.2013.03.020.
- Schuurman W., Levett P.A., Pot M.W., van Weeren P.R., Dhert W.J.A., Hutmacher D.W., Melchels F.P., Klein T.J., Malda J. Gelatin-methacrylamide hydrogels as potential biomaterials for fabrication of tissue-engineered cartilage constructs. Macromol Biosci 2013; 13(5): 551–561, https://doi.org/10.1002/mabi.201200471.
- Rothrauff B.B., Shimomura K., Gottardi R., Alexander P.G., Tuan R.S. Anatomical region-dependent enhancement of 3-dimensional chondrogenic differentiation of human mesenchymal stem cells by soluble meniscus extracellular matrix. Acta Biomater 2017; 49: 140–151, https://doi.org/10.1016/j.actbio.2016.11.046.
- Levato R., Webb W.R., Otto I.A., Mensinga A., Zhang Y., van Rijen M., van Weeren R., Khan I.M., Malda J. The bio in the ink: cartilage regeneration with bioprintable hydrogels and articular cartilage-derived progenitor cells. Acta Biomater 2017; 61: 41–53, https://doi.org/10.1016/j.actbio.2017.08.005.
- Narayanan N., Calve S. Extracellular matrix at the muscle–tendon interface: functional roles, techniques to explore and implications for regenerative medicine. Connect Tissue Res 2021; 62(1): 53–71, https://doi.org/10.1080/03008207.2020.1814263.
- Neff L.S., Bradshaw A.D. Cross your heart? Collagen cross-links in cardiac health and disease. Cell Signal 2021; 79: 109889, https://doi.org/10.1016/j.cellsig.2020.109889.
- Ruiz Martínez M.A., Peralta Galisteo S., Castán H., Morales Hernández M.E. Role of proteoglycans on skin ageing: a review. Int J Cosmet Sci 2020; 42(6): 529–535, https://doi.org/10.1111/ics.12660.
- Tiplea M.G., Lemnaru G.M., Trușcă R.D., Holban A., Kaya M.G.A., Dragu L.D., Ficai D., Ficai A., Bleotu C. Antimicrobial films based on chitosan, collagen, and ZnO for skin tissue regeneration. Biointerface Res Appl Chem 2021; 11(4): 11985–11995, https://doi.org/10.33263/briac114.1198511995.
- Krane S.M. The importance of proline residues in the structure, stability and susceptibility to proteolytic degradation of collagens. Amino Acids 2008: 35(4): 703–710, https://doi.org/10.1007/s00726-008-0073-2.
- Blidi O.E., Omari N.E., Balahbib A., Ghchime R., Menyiy N.E., Ibrahimi A., Kaddour K.B., Bouyahya A., Chokairi O., Barkiyou M. Extraction methods, characterization and biomedical applications of collagen: a review. Biointerface Res Appl Chem 2021; 11(5): 13587–13613, https://doi.org/10.33263/briac115.1358713613.
- Bellis S.L. Advantages of RGD peptides for directing cell association with biomaterials. Biomaterials 2011; 32(18): 4205–4210, https://doi.org/10.1016/j.biomaterials.2011.02.029.
- Marques C.F., Diogo G.S., Pina S., Oliveira J.M., Silva T.H., Reis R.L. Collagen-based bioinks for hard tissue engineering applications: a comprehensive review. J Mater Sci Mater Med 2019; 30(3): 32, https://doi.org/10.1007/s10856-019-6234-x.
- Kulakov A., Kogan E., Brailovskaya T., Vedyaeva A., Zharkov N., Krasilnikova O., Krasheninnikov M., Baranovskii D., Rasulov T., Klabukov I. Mesenchymal stromal cells enhance vascularization and epithelialization within 7 days after gingival augmentation with collagen matrices in rabbits. Dent J (Basel) 2021; 9(9): 101, https://doi.org/10.3390/dj9090101.
- Young S., Wong M., Tabata Y., Mikos A.G. Gelatin as a delivery vehicle for the controlled release of bioactive molecules. J Control Release 2005; 109(1–3): 256–274, https://doi.org/10.1016/j.jconrel.2005.09.023.
- Gorgieva S., Kokol V. Collagen- vs. gelatine-based biomaterials and their biocompatibility: review and perspectives. In: Biomaterials applications for nanomedicine. Pignatello R. (editor). London: InTechOpen; 2011; p. 17–52, https://doi.org/10.5772/24118.
- Van den Steen P.E., Dubois B., Nelissen I., Rudd P.M., Dwek R.A., Opdenakker G. Biochemistry and molecular biology of gelatinase B or matrix metalloproteinase-9 (MMP-9). Crit Rev Biochem Mol Biol 2002; 37(6): 375–536, https://doi.org/10.1080/10409230290771546.
- Boere K.W., Visser J., Seyednejad H., Rahimian S., Gawlitta D., van Steenbergen M.J., Dhert W.J., Hennink W.E., Vermonden T., Malda J. Covalent attachment of a three-dimensionally printed thermoplast to a gelatin hydrogel for mechanically enhanced cartilage constructs. Acta Biomater 2014; 10(6): 2602–2611, https://doi.org/10.1016/j.actbio.2014.02.041.
- Levato R., Visser J., Planell J.A., Engel E., Malda J., Mateos-Timoneda M.A. Biofabrication of tissue constructs by 3D bioprinting of cell-laden microcarriers. Biofabrication 2014; 6(3): 035020, https://doi.org/10.1088/1758-5082/6/3/035020.
- Yue K., Trujillo-de Santiago G., Alvarez M.M., Tamayol A., Annabi N., Khademhosseini A. Synthesis, properties, and biomedical applications of gelatin methacryloyl (GelMA) hydrogels. Biomaterials 2015; 73: 254–271, https://doi.org/10.1016/j.biomaterials.2015.08.045.
- Osidak E.O., Karalkin P.A., Osidak M.S., Parfenov V.A., Sivogrivov D.E., Pereira F.D.A.S., Gryadunova A.A., Koudan E.V., Khesuani Y.D., Кasyanov V.A., Belousov S.I., Krasheninnikov S.V., Grigoriev T.E., Chvalun S.N., Bulanova E.A., Mironov V.A., Domogatsky S.P. Viscoll collagen solution as a novel bioink for direct 3D bioprinting. J Mater Sci Mater Med 2019; 30(3): 31, https://doi.org/10.1007/s10856-019-6233-y.
- Levorson E.J., Hu O., Mountziaris P.M., Kasper F.K., Mikos A.G. Cell-derived polymer/extracellular matrix composite scaffolds for cartilage regeneration, part 2: construct devitalization and determination of chondroinductive capacity. Tissue Eng Part C Methods 2014; 20(4): 358–372, https://doi.org/10.1089/ten.tec.2013.0288.
- Jiang X., Zhong Y., Zheng L., Zhao J. Nano-hydroxyapatite/collagen film as a favorable substrate to maintain the phenotype and promote the growth of chondrocytes cultured in vitro. Int J Mol Med 2018; 41(4): 2150–2158, https://doi.org/10.3892/ijmm.2018.3431.
- Isaeva E.V., Beketov E.E., Yuzhakov V.V., Arguchinskaya N.V., Kisel A.A., Malakhov E.P., Lagoda T.S., Yakovleva N.D., Shegai P.V., Ivanov S.A., Kaprin A.D. The use of collagen with high concentration in cartilage tissue engineering by means of 3D-bioprinting. Cell Tissue Biol 2021; 15(5): 493–502, https://doi.org/10.1134/s1990519x21050059.
- Bialik-Wąs K., Pluta K., Malina D., Barczewski M., Malarz K., Mrozek-Wilczkiewicz A. Advanced SA/PVA-based hydrogel matrices with prolonged release of aloe vera as promising wound dressings. Mater Sci Eng C Mater Biol Appl 2021; 120: 111667, https://doi.org/10.1016/j.msec.2020.111667.
- Mok C.F., Ching Y.C., Muhamad F., Abu Osman N.A., Hai N.D., Che Hassan C.R. Adsorption of dyes using poly (vinyl alcohol) (PVA) and PVA-based polymer composite adsorbents: a review. J Polym Environ 2020; 28: 775–793, https://doi.org/10.1007/s10924-020-01656-4.
- Baranovsky D.S., Lyundup A.V., Balyasin M.V., Klabukov I.D., Krasilnikova O.A., Krasheninnikov M.E., Parshin V.D. Interleukin IL-1β stimulates revitalization of cartilage matrix in vitro with human nasal chondrocytes. Vestnik transplantologii i iskusstvennyh organov 2019; 21(4): 88–95, https://doi.org/10.15825/1995-1191-2019-4-88-95.
- Balyasin M.V., Baranovsky D.S., Demchenko A.G., Fayzullin A.L., Krasilnikova O.A., Klabukov I.D., Krasheninnikov M.E., Lyundup A.V., Parshin V.D. Experimental orthotopic implantation of tissue-engineered tracheal graft created based on devitalized scaffold seeded with mesenchymal and epithelial cells. Vestnik transplantologii i iskusstvennyh organov 2019; 21(4): 96–107, https://doi.org/10.15825/1995-1191-2019-4-96-107.
- Rigogliuso S., Salamone M., Barbarino E., Barbarino M., Nicosia A., Ghersi G. Production of injectable marine collagen-based hydrogel for the maintenance of differentiated chondrocytes in tissue engineering applications. Int J Mol Sci 2020; 21(16): 5798, https://doi.org/10.3390/ijms21165798.
- Okubo R., Asawa Y., Watanabe M., Nagata S., Nio M., Takato T., Hikita A., Hoshi K. Proliferation medium in three-dimensional culture of auricular chondrocytes promotes effective cartilage regeneration in vivo. Regen Ther 2019; 11: 306–315, https://doi.org/10.1016/j.reth.2019.10.002.
- Jin G.Z., Kim H.W. Efficacy of collagen and alginate hydrogels for the prevention of rat chondrocyte dedifferentiation. J Tissue Eng 2018; 9: 2041731418802438, https://doi.org/10.1177/2041731418802438.
- Schlegel W., Nürnberger S., Hombauer M., Albrecht C., Vécsei V., Marlovits S. Scaffold-dependent differentiation of human articular chondrocytes. Int J Mol Med 2008; 22(5): 691–699.
- Pahoff S., Meinert C., Bas O., Nguyen L., Klein T.J., Hutmacher D.W. Effect of gelatin source and photoinitiator type on chondrocyte redifferentiation in gelatin methacryloyl-based tissue-engineered cartilage constructs. J Mater Chem B 2019; 7(10): 1761–1772, https://doi.org/10.1039/c8tb02607f.
- Fedorovich N.E., Oudshoorn M.H., van Geemen D., Hennink W.E., Alblas J., Dhert W.J. The effect of photopolymerization on stem cells embedded in hydrogels. Biomaterials 2009; 30(3): 344–353, https://doi.org/10.1016/j.biomaterials.2008.09.037.