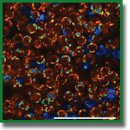
Fluorescence Lifetime Imaging of NAD(P)H T Cells Autofluorescence in the Lymphatic Nodes to Assess the Effectiveness of Anti-CTLA-4 Immunotherapy
The main problem in the field of tumor immunotherapy is the lack of reliable biomarkers that allow pre-determining the susceptibility of individual patients to treatment, as well as insufficient knowledge about the resistance mechanisms.
Biomarkers based on the autofluorescence of metabolic coenzymes in immune cells can become a powerful new predictor of early tumor response to treatment, whereas the optical FLIM method can be a tool to predict the effectiveness of immunotherapy, which allows preserving the spatial structure of the sample and obtaining results on the metabolic status of immune cells in real time.
The aim of the study is to conduct a metabolic autofluorescence imaging study of the NAD(P)H metabolic coenzyme in immune cells of freshly isolated lymph nodes as a potential marker for assessing the effectiveness of an early response to immunotherapy.
Materials and Methods. The study was carried out on C57Bl/6 FoxP3-EGFP mice with B16F0 melanoma implanted near the inguinal lymph node. The mice were injected with antibodies to CTLA-4 (Bio X Cell, USA) (250 μg per mouse, intraperitoneally on days 7, 8, 11, and 12 of the tumor growth). FLIM images in the nicotinamide adenine dinucleotide (phosphate) coenzyme (NAD(P)H) channel (excitation — 375 nm, reception — 435–485 nm) were received using an LSM 880 fluorescent confocal laser scanning microscope (Carl Zeiss, Germany) equipped with a FLIM Simple-Tau module 152 TCSPC (Becker & Hickl GmbH, Germany). Flow cytometry was conducted using a BD FACSAria III cell sorter (BD Biosciences, USA).
Results. Immunotherapy with checkpoint inhibitors resulted in marked metabolic rearrangements in T cells of freshly isolated lymph nodes in responder mice, with inhibition of the tumor growth. Fluorescence lifetime imaging data on NAD(P)H indicated an increase in the free fraction of NADH α1, a form associated with glycolysis to meet high demands of the activated T cells and pro-inflammatory cytokine synthesis. In contrast, non-responder mice with advanced tumors showed low values of the ratio of free fraction to bound α1/α2, which may be related to mechanisms of resistance to therapy.
The response to immunotherapy was verified by data on the expression of activation and proliferation markers by means of flow cytometry. The authors observed an increase in the production of the pro-inflammatory cytokine IFN-ã in effector T cells in responder mice compared to untreated controls and non-responders. In addition, an increase in the expression of the surface activation markers CD25 and CD69 was registered compared to untreated controls.
Conclusion. Use of the FLIM method allowed to demonstrate that autofluorescence of the NAD(P)H coenzyme is sensitive to the response to checkpoint immunotherapy and can be used as a reliable marker of the effectiveness of response to treatment.
- Huang A.C., Zappasodi R. A decade of checkpoint blockade immunotherapy in melanoma: understanding the molecular basis for immune sensitivity and resistance. Nat Immunol 2022; 23(5): 660–670, https://doi.org/10.1038/s41590-022-01141-1.
- Wolchok J.D., Chiarion-Sileni V., Gonzalez R., Grob J.J., Rutkowski P., Lao C.D., Cowey C.L., Schadendorf D., Wagstaff J., Dummer R., Ferrucci P.F., Smylie M., Butler M.O., Hill A., Márquez-Rodas I., Haanen J.B.A.G., Guidoboni M., Maio M., Schöffski P., Carlino M.S., Lebbé C., McArthur G., Ascierto P.A., Daniels G.A., Long G.V., Bas T., Ritchings C., Larkin J., Hodi F.S. Long-term outcomes with nivolumab plus ipilimumab or nivolumab alone versus ipilimumab in patients with advanced melanoma. J Clin Oncol 2022; 40(2): 127–137, https://doi.org/10.1200/jco.21.02229.
- Egen J.G., Ouyang W., Wu L.C. Human anti-tumor immunity: insights from immunotherapy clinical trials. Immunity 2020; 52(1): 36–54, https://doi.org/10.1016/j.immuni.2019.12.010.
- Bai R., Lv Z., Xu D., Cui J. Predictive biomarkers for cancer immunotherapy with immune checkpoint inhibitors. Biomark Res 2020; 8: 34, https://doi.org/10.1186/s40364-020-00209-0.
- Janus G.A., Ievleva A.G., Suspitsyn E.N., Tyurin V.I., Bizin I.V., Gorustovich O.A., Ni V.I., Kholmatov M.M., Laidus T.A., Chuynyshena S.A., Aleksakhina S.N., Imyanitov E.N. Predictive response markers for immune response blocks. Sibirskij onkologiceskij zurnal 2020; 19(4): 123–131, https://doi.org/10.21294/1814-4861-2020-19-4-123-131.
- Lei Y., Li X., Huang Q., Zheng X., Liu M. Progress and challenges of predictive biomarkers for immune checkpoint blockade. Front Oncol 2021; 11: 617335, https://doi.org/10.3389/fonc.2021.617335.
- Li Y.Z., Zhang H.M. Recent advances in primary resistance mechanisms against immune checkpoint inhibitors. Curr Opin Oncol 2022; 34(1): 95–106, https://doi.org/10.1097/cco.0000000000000802.
- Leone R.D., Powell J.D. Metabolism of immune cells in cancer. Nat Rev Cancer 2020; 20: 516–531, https://doi.org/10.1038/s41568-020-0273-y.
- Hu C., Xuan Y., Zhang X., Liu Y., Yang S., Yang K. Immune cell metabolism and metabolic reprogramming. Mol Biol Rep 2022; 49(10): 9783–9795, https://doi.org/10.1007/s11033-022-07474-2.
- Kolenc O.I., Quinn K.P. Evaluating cell metabolism through autofluorescence imaging of NAD(P)H and FAD. Antioxid Redox Signal 2019; 30(6): 875–889, https://doi.org/10.1089/ars.2017.7451.
- Shirshin E.A., Shirmanova M.V., Gayer A.V., Lukina M.M., Nikonova E.E., Yakimov B.P., Budylin G.S., Dudenkova V.V., Ignatova N.I., Komarov D.V., Yakovlev V.V., Becker W., Zagaynova E.V., Shcheslavskiy V.I., Scully M.O. Label-free sensing of cells with fluorescence lifetime imaging: the quest for metabolic heterogeneity. Proc Natl Acad Sci U S A 2022; 119(9): e2118241119, https://doi.org/10.1073/pnas.2118241119.
- Datta R., Heaster T.M., Sharick J.T., Gillette A.A., Skala M.C. Fluorescence lifetime imaging microscopy: fundamentals and advances in instrumentation, analysis, and applications. J Biomed Opt 2020; 25(7): 1–43, https://doi.org/10.1117/1.jbo.25.7.071203.
- Kalinina S., Freymueller C., Naskar N., von Einem B., Reess K., Sroka R., Rueck A. Bioenergetic alterations of metabolic redox coenzymes as NADH, FAD and FMN by means of fluorescence lifetime imaging techniques. Int J Mol Sci 2021; 22(11): 5952, https://doi.org/10.3390/ijms22115952.
- Lukina M.M., Dudenkova V.V., Ignatova N.I., Druzhkova I.N., Shimolina L.E., Zagaynova E.V., Shirmanova M.V. Metabolic cofactors NAD(P)H and FAD as potential indicators of cancer cell response to chemotherapy with paclitaxel. Biochim Biophys Acta Gen Subj 2018; 1862(8): 1693–1700, https://doi.org/10.1016/j.bbagen.2018.04.021.
- Kuznetsova D.S., Rodimova S.A., Gulin A., Reunov D., Bobrov N., Polozova A.V., Vasin A., Shcheslavskiy V.I., Vdovina N., Zagainov V.E., Zagaynova E.V. Metabolic imaging and secondary ion mass spectrometry to define the structure and function of liver with acute and chronic pathology. J Biomed Opt 2019; 25(1): 1–14, https://doi.org/10.1117/1.jbo.25.1.014508.
- Hu L., Wang N., Cardona E., Walsh A.J. Fluorescence intensity and lifetime redox ratios detect metabolic perturbations in T cells. Biomed Opt Express 2020; 11(10): 5674–5688, https://doi.org/10.1364/boe.401935.
- Heaster T.M., Humayun M., Yu J., Beebe D.J., Skala M.C. Autofluorescence imaging of 3D tumor-macrophage microscale cultures resolves spatial and temporal dynamics of macrophage metabolism. Cancer Res 2020; 80(23): 5408–5423, https://doi.org/10.1158/0008-5472.can-20-0831.
- Miskolci V., Tweed K.E., Lasarev M.R., Britt E.C., Walsh A.J., Zimmerman L.J., McDougal C.E., Cronan M.R., Fan J., Sauer J.D., Skala M.C., Huttenlocher A. In vivo fluorescence lifetime imaging of macrophage intracellular metabolism during wound responses in zebrafish. Elife 2022; 11: e66080, https://doi.org/10.7554/elife.66080.
- Walsh A.J., Mueller K.P., Tweed K., Jones I., Walsh C.M., Piscopo N.J., Niemi N.M., Pagliarini D.J., Saha K., Skala M.C. Classification of T-cell activation via autofluorescence lifetime imaging. Nat Biomed Eng 2021; 5(1): 77–88, https://doi.org/10.1038/s41551-020-0592-z.
- Lemire S., Thoma O.M., Kreiss L., Völkl S., Friedrich O., Neurath M.F., Schürmann S., Waldner M.J. Natural NADH and FAD autofluorescence as label-free biomarkers for discriminating subtypes and functional states of immune cells. Int J Mol Sci 2022; 23(4): 2338, https://doi.org/10.3390/ijms23042338.
- Izosimova A.V., Shirmanova M.V., Shcheslavskiy V.I., Sachkova D.A., Mozherov A.M., Sharonov G.V., Zagaynova E.V., Yuzhakova D.V. FLIM of NAD(P)H in lymphatic nodes resolves T-cell immune response to the tumor. Int J Mol Sci 2022; 23(24): 15829, https://doi.org/10.3390/ijms232415829.
- Patton E.E., Mueller K.L., Adams D.J., Anandasabapathy N., Aplin A.E., Bertolotto C., Bosenberg M., Ceol C.J., Burd C.E., Chi P., Herlyn M., Holmen S.L., Karreth F.A., Kaufman C.K., Khan S., Kobold S., Leucci E., Levy C., Lombard D.B., Lund A.W., Marie K.L., Marine J.C., Marais R., McMahon M., Robles-Espinoza C.D., Ronai Z.A., Samuels Y., Soengas M.S., Villanueva J., Weeraratna A.T., White R.M., Yeh I., Zhu J., Zon L.I., Hurlbert M.S., Merlino G. Melanoma models for the next generation of therapies. Cancer Cell 2021; 39(5): 610–631, https://doi.org/10.1016/j.ccell.2021.01.011.
- Izosimova A.V., Yuzhakova D.V., Skatova V.D., Volchkova L.N., Zagainova E.V., Chudakov D.M., Sharonov G.V. Deciphering repertoire of B16 melanoma reactive TCRs by immunization, in vitro restimulation and sequencing of IFNγ-secreting T cells. Int J Mol Sci 2021; 22(18): 9859, https://doi.org/10.3390/ijms22189859.
- Yuzhakova D.V., Volchkova L.N., Pogorelyy M.V., Serebrovskaya E.O., Shagina I.A., Bryushkova E.A., Nakonechnaya T.O., Izosimova A.V., Zavyalova D.S., Karabut M.M., Izraelson M., Samoylenko I.V., Zagainov V.E., Chudakov D.M., Zagaynova E.V., Sharonov G.V. Measuring intratumoral heterogeneity of immune repertoires. Front Oncol 2020; 10: 512, https://doi.org/10.3389/fonc.2020.00512.
- Zhigalova E.A., Izosimova A.I., Yuzhakova D.V., Volchkova L.N., Shagina I.A., Turchaninova M.A., Serebrovskaya E.O., Zagaynova E.V., Chudakov D.M., Sharonov G.V. RNA-seq-based TCR profiling reveals persistently increased intratumoral clonality in responders to anti-PD-1 therapy. Front Oncol 2020; 10: 385, https://doi.org/10.3389/fonc.2020.00385.
- Fontenot J.D., Rasmussen J.P., Williams L.M., Dooley J.L., Farr A.G., Rudensky A.Y. Regulatory T cell lineage specification by the forkhead transcription factor foxp3. Immunity 2005; 22(3): 329–341, https://doi.org/10.1016/j.immuni.2005.01.016.
- Skala M.C., Riching K.M., Bird D.K., Gendron-Fitzpatrick A., Eickhoff J., Eliceiri K.W., Keely P.J., Ramanujam N. In vivo multiphoton fluorescence lifetime imaging of protein-bound and free nicotinamide adenine dinucleotide in normal and precancerous epithelia. J Biomed Opt 2007; 12(2): 024014, https://doi.org/10.1117/1.2717503.
- Waldman A.D., Fritz J.M., Lenardo M.J. A guide to cancer immunotherapy: from T cell basic science to clinical practice. Nat Rev Immunol 2020; 20(11): 651–668, https://doi.org/10.1038/s41577-020-0306-5.
- Liu Y., Zheng P. Preserving the CTLA-4 checkpoint for safer and more effective cancer immunotherapy. Trends Pharmacol Sci 2020; 41(1): 4–12, https://doi.org/10.1016/j.tips.2019.11.003.
- Zheng K., Zheng X., Yang W. The role of metabolic dysfunction in T-cell exhaustion during chronic viral infection. Front Immunol 2022; 13: 843242, https://doi.org/10.3389/fimmu.2022.843242.
- Seidel J.A., Otsuka A., Kabashima K. Anti-PD-1 and anti-CTLA-4 therapies in cancer: mechanisms of action, efficacy, and limitations. Front Oncol 2018; 8: 86, https://doi.org/10.3389/fonc.2018.00086.
- Pires da Silva I., Ahmed T., Reijers I.L.M., Weppler A.M., Betof Warner A., Patrinely J.R., Serra-Bellver P., Allayous C., Mangana J., Nguyen K., Zimmer L., Trojaniello C., Stout D., Lyle M., Klein O., Gerard C.L., Michielin O., Haydon A., Ascierto P.A., Carlino M.S., Lebbe C., Lorigan P., Johnson D.B., Sandhu S., Lo S.N., Blank C.U., Menzies A.M., Long G.V. Ipilimumab alone or ipilimumab plus anti-PD-1 therapy in patients with metastatic melanoma resistant to anti-PD-(L)1 monotherapy: a multicentre, retrospective, cohort study. Lancet Oncol 2021; 22(6): 836–847, https://doi.org/10.1016/s1470-2045(21)00097-8.
- Zappasodi R., Serganova I., Cohen I.J., Maeda M., Shindo M., Senbabaoglu Y., Watson M.J., Leftin A., Maniyar R., Verma S., Lubin M., Ko M., Mane M.M., Zhong H., Liu C., Ghosh A., Abu-Akeel M., Ackerstaff E., Koutcher J.A., Ho P.C., Delgoffe G.M., Blasberg R., Wolchok J.D., Merghoub T. CTLA-4 blockade drives loss of Treg stability in glycolysis-low tumours. Nature 2021; 591(7851): 652–658, https://doi.org/10.1038/s41586-021-03326-4.
- Zhou B., Gao Y., Zhang P., Chu Q. Acquired resistance to immune checkpoint blockades: the underlying mechanisms and potential strategies. Front Immunol 2021; 12: 693609, https://doi.org/10.3389/fimmu.2021.693609.
- Kishton R.J., Sukumar M., Restifo N.P. Metabolic regulation of T cell longevity and function in tumor immunotherapy. Cell Metab 2017; 26(1): 94–109, https://doi.org/10.1016/j.cmet.2017.06.016.