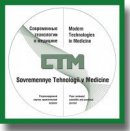
Autoregulation and Autoinhibition of the Main NO Synthase Isoforms (Brief Review)
Nitric oxide (II) (NO) is the most important mediator of a wide range of physiological and pathophysiological processes. It is synthesized by NO synthases (NOSs), which have three main isoforms differing from each other in terms of activation and inhibition features, levels of NO production, subcellular localization, etc. At the same time, all isoforms are structurally very similar, and these differences are determined by NOS autoregulatory elements.
The article presents an analysis of the autoregulatory and autoinhibitory mechanisms of the NOS reductase domain that determine differences in the productivity of isoforms, as well as their dependence on the concentration of Ca2+ ions. The main regulatory elements in NOS that modulate the electron transfer from flavin to heme include calmodulin (CaM), an autoinhibitory insert (AI), and the C-terminal tail (C-tail). Hydrophobic interactions of CaM with the surface of the NOS oxidase domain are assumed to facilitate electron transfer from flavin mononucleotide (FMN). CaM binding causes a change in the inter-domain distances, a shift of AI and the C-tail, and, as a result, a decrease in their inhibitory effect. CaM also shifts the conformational equilibrium of the reductase domain towards more open conformations, reduces the lifetime of conformations, their stereometric distribution, and accelerates the flow of electrons through the reductase domain. The AI element, apparently, induces a conformational change that hinders electron transfer within the reductase domain, similar to the hinge domain in cytochrome P450. Together with CaM, the C-tail regulates the electron flow between flavins, the distance and relative orientation of isoalloxane rings, and also modulates the electron flow from FMN to the terminal acceptor. Together with the C-tail, AI also predetermines the dependence of neuronal and endothelial forms of NOS on the concentration of Ca2+ ions, and the C-tail length affects differences in the productivity of NO synthesis. The inhibitory effect of the C-tail is likely to be reduced by CaM binding due to the C-tail shift due to the electrostatic repulsive forces of the negatively charged phosphate and aspartate residues. The autoregulatory elements of NOS require further study, since the mechanisms of their interaction are complex and multidirectional, and hence provide a wide range of characteristics of the observed isoforms.
- Carlström M. Nitric oxide signalling in kidney regulation and cardiometabolic health. Nat Rev Nephrol 2021; 17(9): 575–590, https://doi.org/10.1038/s41581-021-00429-z.
- Liy P.M., Puzi N.N.A., Jose S., Vidyadaran S. Nitric oxide modulation in neuroinflammation and the role of mesenchymal stem cells. Exp Biol Med (Maywood) 2021; 246(22): 2399–2406, https://doi.org/10.1177/1535370221997052.
- Iwakiri Y., Kim M.Y. Nitric oxide in liver diseases. Trends Pharmacol Sci 2015; 36(8): 524–536, https://doi.org/10.1016/j.tips.2015.05.001.
- Stuehr D.J., Haque M.M. Nitric oxide synthase enzymology in the 20 years after the Nobel Prize. Br J Pharmacol 2019; 176(2): 177–188, https://doi.org/10.1111/bph.14533.
- Campbell M.G., Smith B.C., Potter C.S., Carragher B., Marletta M.A. Molecular architecture of mammalian nitric oxide synthases. Proc Natl Acad Sci U S A 2014; 111(35): E3614–E3623, https://doi.org/10.1073/pnas.1413763111.
- Cinelli M.A., Do H.T., Miley G.P., Silverman R.B. Inducible nitric oxide synthase: regulation, structure, and inhibition. Med Res Rev 2020; 40(1): 158–189, https://doi.org/10.1002/med.21599.
- Król M., Kepinska M. Human nitric oxide synthase — its functions, polymorphisms, and inhibitors in the context of inflammation, diabetes and cardiovascular diseases. Int J Mol Sci 2020; 22(1): 56, https://doi.org/10.3390/ijms22010056.
- Tsutsui M., Tanimoto A., Tamura M., Mukae H., Yanagihara N., Shimokawa H., Otsuji Y. Significance of nitric oxide synthases: lessons from triple nitric oxide synthases null mice. J Pharmacol Sci 2015 127(1): 42–52, https://doi.org/10.1016/j.jphs.2014.10.002.
- Li H., Jamal J., Plaza C., Pineda S.H., Chreifi G., Jing Q., Cinelli M.A., Silverman R.B., Poulos T.L. Structures of human constitutive nitric oxide synthases. Acta Crystallogr D Biol Crystallogr 2014; 70(Pt 10): 2667–2674, https://doi.org/10.1107/s1399004714017064.
- Popova N.A., Klimanov I.A., Soodaeva S.K., Temnov A.A. Formation of the structural scheme for universal NO-synthase catalytic cycle model. Sovremennye problemy nauki i obrazovania 2022; 4, https://doi.org/10.17513/spno.31989.
- Kobayashi K., Tagawa S., Daff S., Sagami I., Shimizu T. Rapid calmodulin-dependent interdomain electron transfer in neuronal nitric-oxide synthase measured by pulse radiolysis. J Biol Chem 2001; 276(43): 39864–39871, https://doi.org/10.1074/jbc.m102537200.
- Hanson Q.M., Carley J.R., Gilbreath T.J., Smith B.C., Underbakke E.S. Calmodulin-induced conformational control and allostery underlying neuronal nitric oxide synthase activation. J Mol Biol 2018; 430(7): 935–947, https://doi.org/10.1016/j.jmb.2018.02.003.
- Nishida C.R., Ortiz de Montellano P.R. Autoinhibition of endothelial nitric-oxide synthase. Identification of an electron transfer control element. J Biol Chem 1999; 274(21): 14692–14698, https://doi.org/10.1074/jbc.274.21.14692.
- Li J., Zheng H., Feng C. Deciphering mechanism of conformationally controlled electron transfer in nitric oxide synthases. Front Biosci (Landmark Ed) 2018; 23(10): 1803–1821, https://doi.org/10.2741/4674.
- Roman L.J., Martásek P., Miller R.T., Harris D.E., de la Garza M.A., Shea T.M., Kim J.J.P., Masters B.S.S. The C termini of constitutive nitric-oxide synthases control electron flow through the flavin and heme domains and affect modulation by calmodulin. J Biol Chem 2000; 275(38): 29225–29232, https://doi.org/10.1074/jbc.m004766200.
- Panda S.P., Li W., Venkatakrishnan P., Chen L., Astashkin A.V., Masters B.S., Feng C., Roman L.J. Differential calmodulin-modulatory and electron transfer properties of neuronal nitric oxide synthase mu compared to the alpha variant. FEBS Lett 2013; 587(24): 3973–3978, https://doi.org/10.1016/j.febslet.2013.10.032.