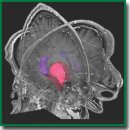
Hippocampal Functional Connectivity and Cognitive Stability in Parasellar Meningiomas (Studied by the “Virtually Implanted Electrode” Method)
Adaptive reactions of the brain ensure cognitive stability of the individual in a fairly wide range of pathological impacts, but the mechanisms for the implementation of such compensatory changes have been poorly studied.
The aim of the study was to describe changes in the functional connections of the hippocampus subject to a mild unilateral compression in a sample of patients with parasellar meningiomas.
Materials and Methods. A homogeneous sample of 28 patients with parasellar meningiomas adjacent to the hippocampus has been studied. In 16 patients, the tumor was diagnosed on the left side, in 12 patients on the right side. These two groups were comparable in terms of tumor morphometric characteristics and the degree of hemispheric compression. The control group consisted of 31 healthy subjects. All three groups were comparable in age and gender. The “Virtually Implanted Electrode” method was used to describe changes in brain network connectivity. The method allows for the reconstruction of electrical activity in any brain voxel based on its coordinates relative to scalp electrodes. To describe the functional connectivity of the brain, correlation coefficients between all pairs of the selected areas of interest were sequentially calculated.
Results. The comparison of functional connections of the hippocampus in clinical groups and in a group of healthy participants made it possible to identify the following types of dynamics. The first type involves strong and stable hippocampal connections that have not been affected by the pathological process. These are the connections of the hippocampus with the deep stem formations, amygdala, putamen, globus pallidus, and insula. The second type in the clinical groups is characterized by weakening of functional connections of the hippocampus with the structures that transform afferent information flows. Hypothetically, such a weakening could lead to a change in the thresholds of the hippocampal “marking the degree of novelty” of external information flows, being an important way to save individual’s resources. The third type is characterized by enhanced functional connections of the hippocampus with the structures supporting executive functions in clinical groups, which is consistent with the facts of increased voluntariness in the implementation of cognitive actions. Compensatory processes of the brain are not symmetrical. The left and right hippocampi differentially alter functional connectivity under adverse conditions. Restructuring of the interhemispheric interaction may also be considered as a factor ensuring cognitive stability.
Conclusion. Changes in the hippocampal functional connections, identified in the clinical groups by the “Virtual Implanted Electrode” method, can be considered as an adaptive brain reaction aimed at maintaining cognitive stability in parasellar meningiomas.
- Friston K.J., Kahan J., Biswal B., Razi A. A DCM for resting state fMRI. Neuroimage 2014; 94(100): 396–407, https://doi.org/10.1016/j.neuroimage.2013.12.009.
- Kawagoe T., Onoda K., Yamaguchi S. Subjective memory complaints are associated with altered resting-state functional connectivity but not structural atrophy. Neuroimage Clin 2019; 21: 101675, https://doi.org/10.1016/j.nicl.2019.101675.
- Anokhin K.V. Cognitome: in search of fundamental neuroscience theory of consciousness. Zhurnal vysshei nervnoi deyatelnosti imeni I.P. Pavlova 2021; 71(1): 39–71.
- Proshina E.A., Deynekina T.S., Martynova O.V. Neurogenetics of brain connectivity: current approaches to the study (review). Sovremennye tehnologii v medicine 2024; 16(1): 66–76, https://doi.org/10.17691/stm2024.16.1.07.
- Fox M.D., Raichle M.E. Spontaneous fluctuations in brain activity observed with functional magnetic resonance imaging. Nat Rev Neurosci 2007; 8(9): 700–711, https://doi.org/10.1038/nrn2201.
- Yeshurun Y., Nguyen M., Hasson U. The default mode network: where the idiosyncratic self meets the shared social world. Nat Rev Neurosci 2021; 22(3): 181–192, https://doi.org/10.1038/s41583-020-00420-w.
- Damulin I.V., Strutzenko A.A. Contemporary concept about organization of central nervous system: human connectome and neural networks. Medical alphabet 2021; 22: 42–47, https://doi.org/10.33667/2078-5631-2021-22-42-47.
- Raichle M.E. The brain’s default mode network. Annu Rev Neurosci 2015; 38: 433–447, https://doi.org/10.1146/annurev-neuro-071013-014030.
- Konishi M., McLaren D.G., Engen H., Smallwood J. Shaped by the past: The default mode network supports cognition that is independent of immediate perceptual input. PLoS One 2015; 10(6): e0132209, https://doi.org/10.1371/journal.pone.0132209.
- Chen T., Cai W., Ryali S., Supekar K., Menon V. Distinct global brain dynamics and spatiotemporal organization of the salience network. PLoS Biol 2016; 14(6): e1002469, https://doi.org/10.1371/journal.pbio.1002469.
- Kurgansky A.V. Human brain functional organization in the resting state. Zhurnal vysshei nervnoi deyatelnosti imeni I.P. Pavlova 2018; 68(5): 567–580, https://doi.org/10.1134/S004446771805009X.
- Feklicheva I.V., Chipeeva N.A., Zakharov I.M., Ismatullina V.I., Maslennikova E.P., Tabueva A.O., Soldatova E.L., Malykh S.B. Organization of psychological rehabilitation the personal staff of the internal affairs. Theoretical and Experimental Psychology 2020; 13(3): 65–78.
- Chenot Q., Lepron E., De Boissezon X., Scannella S. Functional connectivity within the fronto-parietal network predicts complex task performance: a fNIRS study. Front Neuroergon 2021; 2: 718176, https://doi.org/10.3389/fnrgo.2021.718176.
- Bukkieva Т.А., Chegina D.S., Еfimtsev А.Yu., Levchuk A.G., Iskhakov D.K., Sokolov A.V., Fokin V.A., Trufanov G.E. Resting state functional MRI. General issues and clinical application. Russian Electronic Journal of Radiology 2019; 9(2): 150–170, https://doi.org/10.21569/2222-7415-2019-9-2-150-170.
- Westlye E.T., Lundervold A., Rootwelt H., Lundervold A.J., Westlye L.T. Increased hippocampal default mode synchronization during rest in middle-aged and elderly APOE ε4 carriers: relationships with memory performance. J Neurosci 2011; 31(21): 7775–7783, https://doi.org/10.1523/JNEUROSCI.1230-11.2011.
- Li Q., Tavakol S., Royer J., Larivière S., Vos De Wael R., Park Bo-yong, Paquola C., Zeng D., Caldairou B., Bassett D.S., Bernasconi A., Bernasconi N., Frauscher B., Smallwood J., Caciagli L., Li S., Bernhardt B.C. Human brain function during pattern separation follows hippocampal and neocortical connectivity gradients. bioRxiv 2020, https://doi.org/10.1101/2020.06.22.165290.
- Wales R.M., Leung H.C. The effects of amyloid and tau on functional network connectivity in older populations. Brain Connect 2021; 11(8): 599–612, https://doi.org/10.1089/brain.2020.0902.
- Zhang Y., Gong X., Yin Z., Cui L., Yang J., Wang P., Zhou Y., Jiang X., Wei S., Wang F., Tang Y. Association between NRGN gene polymorphism and resting-state hippocampal functional connectivity in schizophrenia. BMC Psychiatry 2019; 19(1): 108, https://doi.org/10.1186/s12888-019-2088-5.
- Gao S., Ming Y., Wang J., Gu Y., Ni S., Lu S., Zhang R., Sun J., Zhang N., Xu X. Enhanced prefrontal regional homogeneity and its correlations with cognitive dysfunction/psychopathology in patients with first-diagnosed and drug-naive schizophrenia. Front Psychiatry 2020; 11: 580570, https://doi.org/10.3389/fpsyt.2020.580570.
- Wang X., Yin Z., Sun Q., Jiang X., Chao L., Dai X., Tang Y. Comparative study on the functional connectivity of amygdala and hippocampal neural circuits in patients with first-episode schizophrenia and other high-risk populations. Front Psychiatry 2021; 12: 627198, https://doi.org/10.3389/fpsyt.2021.627198.
- Geerlings M.I., Gerritsen L. Late-life depression, hippocampal volumes, and hypothalamic-pituitary-adrenal axis regulation: a systematic review and meta-analysis. Biol Psychiatry 2017; 82(5): 339–350, https://doi.org/10.1016/j.biopsych.2016.12.032.
- Zhu Y., Qi S., Zhang B., He D., Teng Y., Hu J., Wei X. Connectome-based biomarkers predict subclinical depression and identify abnormal brain connections with the lateral habenula and thalamus. Front Psychiatry 2019; 10: 371, https://doi.org/10.3389/fpsyt.2019.00371.
- Zhang B., Qi S., Liu S., Liu X., Wei X., Ming D. Altered spontaneous neural activity in the precuneus, middle and superior frontal gyri, and hippocampus in college students with subclinical depression. BMC Psychiatry 2021; 21(1): 280, https://doi.org/10.1186/s12888-021-03292-1.
- Qiu S., Chen F., Chen G., Jia Y., Gong J., Luo X., Zhong S., Zhao L., Lai S., Qi Z., Huang L., Wang Y. Abnormal resting-state regional homogeneity in unmedicated bipolar II disorder. J Affect Disord 2019; 256: 604–610, https://doi.org/10.1016/j.jad.2019.06.037.
- Perry A., Roberts G., Mitchell P.B., Breakspear M. Connectomics of bipolar disorder: a critical review, and evidence for dynamic instabilities within interoceptive networks. Mol Psychiatry 2019; 24(9): 1296–1318, https://doi.org/10.1038/s41380-018-0267-2.
- Brunec I.K., Bellana B., Ozubko J.D., Man V., Robin J., Liu Z.X., Grady C., Rosenbaum R.S., Winocur G., Barense M.D., Moscovitch M. Multiple scales of representation along the hippocampal anteroposterior axis in humans. Curr Biol 2018; 28(13): 2129–2135.e6, https://doi.org/10.1016/j.cub.2018.05.016.
- Velichkovsky B.M., Krotkova O.A., Sharaev M.G., Ushakov V.L. In search of the “I”: Neuropsychology of lateralized thinking meets dynamic causal modeling. Psychology in Russia: State of the Art 2017; 10(3): 7–27, https://doi.org/10.11621/pir.2017.0301.
- Velichkovsky B.M., Krotkova O.A., Kotov A.A., Orlov V.A., Verkhlyutov V.M., Ushakov V.L., Sharaev M.G. Consciousness in a multilevel architecture: evidence from the right side of the brain. Conscious Cogn 2018; 64: 227–239, https://doi.org/10.1016/j.concog.2018.06.004.
- Sun W., Advani M., Spruston N., Saxe A., Fitzgerald J.E. Organizing memories for generalization in complementary learning systems. Nat Neurosci 2023; 26(8): 1438–1448, https://doi.org/10.1038/s41593-023-01382-9.
- Vinogradova O.S. Gippokamp i pamyat’ [Hippocampus and memory]. Moscow: Nauka; 1975.
- Zeidman P., Maguire E.A. Anterior hippocampus: the anatomy of perception, imagination and episodic memory. Nat Rev Neurosci 2016; 17(3): 173–182, https://doi.org/10.1038/nrn.2015.24.
- Voss J.L., Bridge D.J., Cohen N.J., Walker J.A. A closer look at the hippocampus and memory. Trends Cogn Sci 2017; 21(8): 577–588, https://doi.org/10.1016/j.tics.2017.05.008.
- Maurer A.P., Nadel L. The continuity of context: a role for the hippocampus. Trends Cogn Sci 2021; 25(3): 187–199, https://doi.org/10.1016/j.tics.2020.12.007.
- Galkin M.V., Danilov G.V., Kaverina M.Y., Strunina Y.V., Krotkova O.A. Hippocampal dosimetry and mnemonic function changes after stereotactic irradiation of cavernous sinus meningiomas. Cureus 2021; 13(12):e20252, https://doi.org/10.7759/cureus.20252.
- Gonzalez-Castillo J., Kam J.W.Y., Hoy C.W., Bandettini P.A. How to interpret resting-state fMRI: ask your participants. J Neurosci 2021; 41(6): 1130–1141, https://doi.org/10.1523/JNEUROSCI.1786-20.2020.
- Hou X., Zhang Z., Zhao C., Duan L., Gong Y., Li Z., Zhu C. NIRS-KIT: a MATLAB toolbox for both resting-state and task fNIRS data analysis. Neurophotonics 2021; 8(1): 010802, https://doi.org/10.1117/1.NPh.8.1.010802.
- Arun K.M., Smitha K.A., Sylaja P.N., Kesavadas C. Identifying resting-state functional connectivity changes in the motor cortex using fNIRS during recovery from stroke. Brain Topogr 2020; 33(6): 710–719, https://doi.org/10.1007/s10548-020-00785-2.
- Das A., de Los Angeles C., Menon V. Electrophysiological foundations of the human default-mode network revealed by intracranial-EEG recordings during resting-state and cognition. Neuroimage 2022; 250: 118927, https://doi.org/10.1016/j.neuroimage.2022.118927.
- Abdulaev S.K., Tarumov D.A., Bogdanovskaya A.S. Resting state functional magnetic resonance imaging: an analysis of the connectivity of brain large-scale networks. Medical Visualization 2023; 28(1): 45–56, https://doi.org/10.24835/1607-0763-1374.
- Vartanov A.V. A new method of localizing brain activity using the scalp EEG data. Procedia Comput Sci 2022; 213: 41–48, https://doi.org/10.1016/j.procs.2022.11.036.
- Vartanov А.V. A new approach to spatial localization of EEG-based electrical activity. Epilepsy and Paroxysmal Conditions 2023; 15(4): 326–338, https://doi.org/10.17749/2077-8333/epi.par.con.2023.177.
- Vartanov A.V. Method for studying brain activity based on scalp electroencephalogram data. A.С. 278526 RU, IPC A61B5372. 2022.
- Chera B.S., Amdur R.J., Patel P., Mendenhall W.M. A radiation oncologist’s guide to contouring the hippocampus. Am J Clin Oncol 2009; 32(1): 20–22, https://doi.org/10.1097/COC.0b013e318178e4e8.
- Leal S.L., Yassa M.A. Integrating new findings and examining clinical applications of pattern separation. Nat Neurosci 2018; 21(2): 163–173, https://doi.org/10.1038/s41593-017-0065-1.
- Stark S.M., Kirwan C.B., Stark C.E.L. Mnemonic similarity task: a tool for assessing hippocampal integrity. Trends Cogn Sci 2019; 23(11): 938–951, https://doi.org/10.1016/j.tics.2019.08.003.
- Riphagen J.M., Schmiedek L., Gronenschild E.H.B.M., Yassa M.A., Priovoulos N., Sack A.T., Verhey F.R.J., Jacobs H.I.L. Associations between pattern separation and hippocampal subfield structure and function vary along the lifespan: a 7 T imaging study. Sci Rep 2020; 10(1): 7572, https://doi.org/10.1038/s41598-020-64595-z.
- Stevenson R.F., Reagh Z.M., Chun A.P., Murray E.A., Yassa M.A. Pattern separation and source memory engage distinct hippocampal and neocortical regions during retrieval. J Neurosci 2020; 40(4): 843–851, https://doi.org/10.1523/JNEUROSCI.0564-19.2019.
- Krotkova O.A., Kuleva A.Y., Galkin M.V., Kaverina M.Y., Strunina Y.V., Danilov G.V. memory modulation factors in hippocampus exposed to radiation. Sovremennye tehnologii v medicine 2021; 13(4): 6–13, https://doi.org/10.17691/stm2021.13.4.01.
- Krotkova O.A., Danilov G.V., Galkin M.V., Kuleva A.Yu., Kaverina M.Yu., Enikolopova E.V., Strunina Yu.V., Enikolopov G.N. Dissociation of cognitive changes under unilateral exposure of the hippocampus to radiation. Human Physiology 2024; 50(2): 87–98, https://doi.org/10.1134/s0362119723600546.
- Lisman J., Buzsáki G., Eichenbaum H., Nadel L., Ranganath C., Redish A.D. Viewpoints: how the hippocampus contributes to memory, navigation and cognition. Nat Neurosci 2017; 20(11): 1434–1447, https://doi.org/10.1038/nn.4661.
- Biderman N., Bakkour A., Shohamy D. What are memories for? The hippocampus bridges past experience with future decisions. Trends Cogn Sci 2020; 24(7): 542–556, https://doi.org/10.1016/j.tics.2020.04.004.
- Yassa M.A., Stark C.E. Pattern separation in the hippocampus. Trends Neurosci 2011; 34(10): 515–525, https://doi.org/10.1016/j.tins.2011.06.006.
- Tolentino J.C., Pirogovsky E., Luu T., Toner C.K., Gilbert P.E. The effect of interference on temporal order memory for random and fixed sequences in nondemented older adults. Learn Mem 2012; 19(6): 251–255, https://doi.org/10.1101/lm.026062.112.
- Yassa M.A., Lacy J.W., Stark S.M., Albert M.S., Gallagher M., Stark C.E. Pattern separation deficits associated with increased hippocampal CA3 and dentate gyrus activity in nondemented older adults. Hippocampus 2011; 21(9): 968–979, https://doi.org/10.1002/hipo.20808.
- Creer D.J., Romberg C., Saksida L.M., van Praag H., Bussey T.J. Running enhances spatial pattern separation in mice. Proc Natl Acad Sci U S A 2010; 107(5): 2367–2372, https://doi.org/10.1073/pnas.0911725107.
- Treder M.S., Charest I., Michelmann S., Martín-Buro M.C., Roux F., Carceller-Benito F., Ugalde-Canitrot A., Rollings D.T., Sawlani V., Chelvarajah R., Wimber M., Hanslmayr S., Staresina B.P. The hippocampus as the switchboard between perception and memory. Proc Natl Acad Sci U S A 2021; 118(50): e2114171118, https://doi.org/10.1073/pnas.2114171118.
- Donos C., Rollo P., Tombridge K., Johnson J.A., Tandon N. Visual field deficits following laser ablation of the hippocampus. Neurology 2020; 94(12): e1303–e1313, https://doi.org/10.1212/WNL.0000000000008940.
- Reyes A., Holden H.M., Chang Y.A., Uttarwar V.S., Sheppard D.P., DeFord N.E., DeJesus S.Y., Kansal L., Gilbert P.E., McDonald C.R. Impaired spatial pattern separation performance in temporal lobe epilepsy is associated with visuospatial memory deficits and hippocampal volume loss. Neuropsychologia 2018; 111: 209–215, https://doi.org/10.1016/j.neuropsychologia.2018.02.009.
- Ma T.M., Grimm J., McIntyre R., Anderson-Keightly H., Kleinberg L.R., Hales R.K., Moore J., Vannorsdall T., Redmond K.J. A prospective evaluation of hippocampal radiation dose volume effects and memory deficits following cranial irradiation. Radiother Oncol 2017; 125(2): 234–240, https://doi.org/10.1016/j.radonc.2017.09.035.
- Haldbo-Classen L., Amidi A., Lukacova S., Wu L.M., Oettingen G.V., Lassen-Ramshad Y., Zachariae R., Kallehauge J.F., Høyer M. Cognitive impairment following radiation to hippocampus and other brain structures in adults with primary brain tumours. Radiother Oncol 2020; 148: 1–7, https://doi.org/10.1016/j.radonc.2020.03.023.
- Boldyreva G.N., Kuleva A.Yu., Sharova E.V., Galkin M.V., Masherov E.L., Krotkova O.A. Search for functional markers of the hippocampus involvement in the pathological process. Human Physiology 2023; 49(2): 95–106, https://doi.org/10.1134/s0362119722700153.
- Krotkova О.А. Psychophysical problem and the hemispheric asymmetry. Vestnik Moskovskogo universiteta. Seriya 14. Psikhologiya 2014; 3: 47–62.